Introduction
In fish, gonadal development and maturation are regulated by two gonadotropins (GTHs): follicle-stimulating hormone (FSH) and luteinizing hormone (LH). FSH mainly regulates vitellogenesis in females and initiation of spermatogenesis in males; thereafter, LH mainly regulates oocyte maturation in females and spermiation in males. FSH and LH secreted by the pituitary gland are glycoprotein hormones comprising an α-subunit and a β-subunit (Kim et al., 2005; Yaron et al., 2003). Although the α-subunits are common to these glycoproteins, the β-subunits are unique to each hormone (Byambaragchaa et al., 2018; Combarnous, 1992; Kim et al., 2016; Min et al., 1996).
A stable supply of seedlings and control of sex maturation of the bloodstock is very important for fish culture (Hamidoghli et al., 2019). However, in the case of female eels, the induction of sexual maturity is very difficult in artificial environmental conditions (Ijiri et al., 2011; Tanaka, 2015) because they have a very low ability to synthesize GTHs from the pituitary gland in such an environment (Kagawa et al., 1998; Kim et al., 2007). Therefore, a high concentration of exogenous hormones is periodically administered to induce sexual maturity. In the case of Anguilla japonica, gonadal development can be induced by salmon pituitary extract (SPE) administration (Kagawa et al., 1998, Kim et al., 2007; Tanaka et al., 2001; Yamamoto & Yamauchi, 1974). However, it has also been reported that repeated exposure of female eels to high doses of exogenous hormones results in eggs of poor quality, and fertilization malformations (Okamura et al., 2011; Shin, 2004). Likewise, spermiation of fishes, including eels, could be induced by human chorionic gonadotropin (hCG) as an LH effect (Miura et al., 1991). However, the duration of the spermiation and the quality of milt following hCG administration showed large individual differences in male eels (Kim et al., 2018; Otha et al., 1997). To solve these problems, it is necessary to develop a gonadotropic reagent for stable artificial seed production that improves egg quality and quantity.
Advancements in biotechnology have made it possible to isolate and clone the GTH genes of a variety of fishes (Choi et al., 2005; Kim et al., 2005) and to produce species-specific recombinant GTHs (rec-GTHs) using invertebrate bioreactor systems (Choi et al., 2016; Kazeto et al., 2008; Kobayashi et al., 2010). Furthermore, biological activity assays are being conducted by investigating the in vivo and in vitro effects of rec-GTHs in fish (Byambaragchaa et al., 2018; Kamei et al., 2003; Kim et al., 2017; Kim et al., 2019; Ko et al., 2007; Vischer et al., 2003). In particular, in our previous study, a baculovirus system was used to produce recombinant GTHs in an eel, and the biological activities of rec-GTHs were examined by measuring the percentage of germinal vesicle breakdown in vitro (Choi et al., 2016). The assay results revealed that rec-GTHs were positively involved in the induction of maturation under in vitro conditions (Choi et al., 2016). However, the in vivo effect of rec-GTHs on sexual maturity in female eels, and the biological activity of recombinant hormones in male eels had not been investigated.
In the present study, we attempted to produce rec-eel FSH and rec-eel LH from CHO-suspension (CHO-S) cell line, and assayed their biological effects on female and male eels both in vitro and in vivo.
Materials and Methods
Male Japanese eels (approximately 300–500 g each) and female eels (approximately 450–500 g each) were cultured in 1-ton tanks with aerated fresh water at a temperature of 20 ± 0.5°C, at the Inland Aquaculture Research Center, National Institute of Fisheries Science, Changwon. 20 male eels and 20 females were used in the experiment. All eels were acclimatized to seawater for one week before the experiment. A black shade film was installed on the water tank to stabilize the fish. Furthermore, ID microchips (∮ 2.1 × 12 mm) were inserted into the dorsal muscles for individual control of each eel, and a mini portable reader (HS5900LF, DESTRON Technologies, Kenilworth, NJ, USA) was used for identification.
cDNAs encoding eel LHβ/α and eel FSHβ/α were inserted into the pcDNA3 mammalian expression vector as previously reported (Byambaragchaa et al., 2018; Kim et al., 2016; Kim et al., 2019). CHO-S cells were transfected using the liposome transfection method, as previously described (Byambaragchaa et al., 2018; Kim et al., 2019). In brief, both plasmids were transfected into CHO-S cells using the FreeStyle MAX reagent transfection method. One day before transfection, CHO-S cells were passaged at a density of 5 × 105 cells/mL. The flasks were placed on an orbital shaker platform rotating at 360–405×g at 37°C in a humidified atmosphere of 8% CO2 in air. The next day, 260 µg of plasmid DNA was diluted in OptiPROTM serum-free medium to a total volume of 4 mL. A total of 260 µL of FreeStyleTM MAX reagent was diluted in OptiPROTM serum-free medium to a total volume of 4 mL. DNA-FreeStyleTM MAX was mixed and incubated for 10 min at room temperature. Then, the complex mixture was slowly added to 200 mL of medium containing the cells. The culture media were collected on day 7 after transfection and centrifuged at 100,000×g at 4°C for 10 min to remove cell debris. The supernatant samples were concentrated by freeze-drying. Recombinant proteins for eel LH and eel FSH were analyzed using an enzyme-linked immunosorbent assay as previously described (Kim et al., 2016).
Healthy eels were anesthetized with 2-phenoxyethanol (200 ppm), and the ovaries were removed to measure the gonadosomatic index (GSI) (gonadal weight/body weight). The ovarian tissue of GSI 2.3 was sectioned to 100 mg, and then a fragment (100 mg) of the ovarian tissue was cultured in each well of 24-well plates (n = 6). L-15 medium (penicillin G sodium 70 mg/L, streptomycin 100 mg/L, HEPES 10 mM, pH 7.4) was used for the culture of ovarian tissue and incubated at 20°C for 2 h. Then, rec-follicle-stimulating hormone (rec-FSH) and rec-LH, rec-luteinizing hormone (rec-LH) were added at concentrations of 0.05, 0.1, 0.5, and 1 µg/ mL per well and incubated at 20°C for 24 h. At the end of the incubation, the culture media were collected, and estradiol-17β (E2) concentration was measured using an ELISA kit (DRG, Estradiol ELISA, EIA-2693).
Female eels weighing 450–500 g each, were injected intramuscularly with eel’s ringer (control), SPE (20 mg/fish), rec-FSH (0.1 µg/g BW), rec-LH (0.1 µg/g BW), and rec-FSH + rec-LH (0.05 µg + 0.05 µg/g BW) once a week. After 4 and 8 injections, 8–10 eels from each experimental group were collected for blood sampling and GSI measurements. Blood samples were taken from the caudal vasculature with a heparinized 1-mL syringe and needle (23G) after anesthetization with 2-phenoxyethanol (200 ppm). The blood samples were centrifuged at 4°C and 15,000×g for 15 min and stored at −80°C until the assay. The plasma E2 levels in the female eels were measured using an ELISA kit (DRG, Estradiol ELISA, EIA-2693).
Male eels weighing 300–500 g each, were injected intramuscularly with eel’s ringer (control), hCG (1 IU/g of BW), rec-FSH (0.1 µg/g of BW), rec-LH (0.1 µg/g of BW), and rec-FSH + rec-LH (0.05 µg + 0.05 µg/g of BW) once a week. After 3 and 8 injections, 8–10 eels were collected for blood sampling and GSI measurements. Blood samples were taken from the caudal vasculature with a heparinized 1 mL syringe and needle (23G) after anesthetization with 2-phenoxyethanol (200 ppm). The blood samples were centrifuged at 4°C at 15,000×g for 15 min and stored at –80°C until the assay. Plasma testosterone (T) and 11-ketotestosterone (11-KT) levels in male eels were measured using an ELISA kit (MyBioSource, San Diego, CA, USA).
Results
The in vitro effects of rec-GTHs on E2 production in immature ovarian tissues are shown in Fig. 1. E2 levels were significantly higher in all treated groups than in the control group. Specifically, the highest E2 levels were achieved in groups treated with 0.1 µg/mL and 0.5 µg/mL of the rec-GTH.
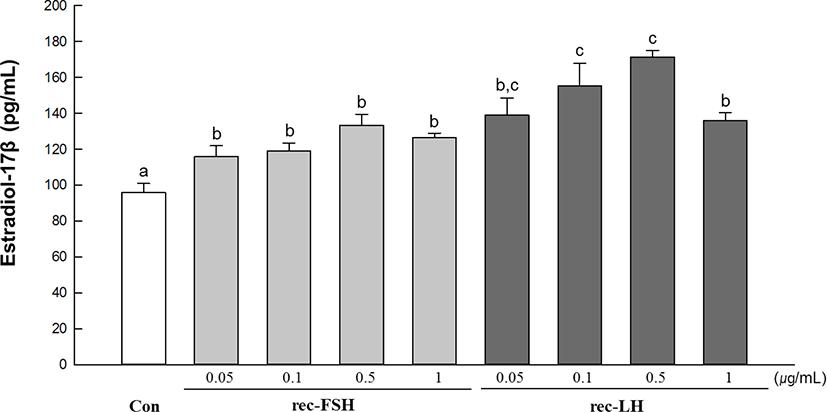
The changes in plasma E2 levels and GSI according to rec-GTHs and SPE administration are shown in Fig. 2. After 4 and 8 weeks of SPE administration, plasma E2 levels were significantly increased compared to the control. SPE treatment increased the E2 levels, as has been previously reported (Kim et al., 2007, 2008). However, the groups treated with rec-FSH and rec-LH achieved significantly increased the plasma E2 levels at 4 weeks after treatment, but there was no significant change in plasma E2 levels at 8 weeks compared to the control group. In the rec-FSH + rec-LH mixture treatment group, no significant changes were observed compared with the control group at 4 and 8 weeks after treatment. The GSI was significantly increased at 4 and 8 weeks after treatment compared to the control in the group treated with SPE. However, significant changes in GSI were not observed in any of the other groups.
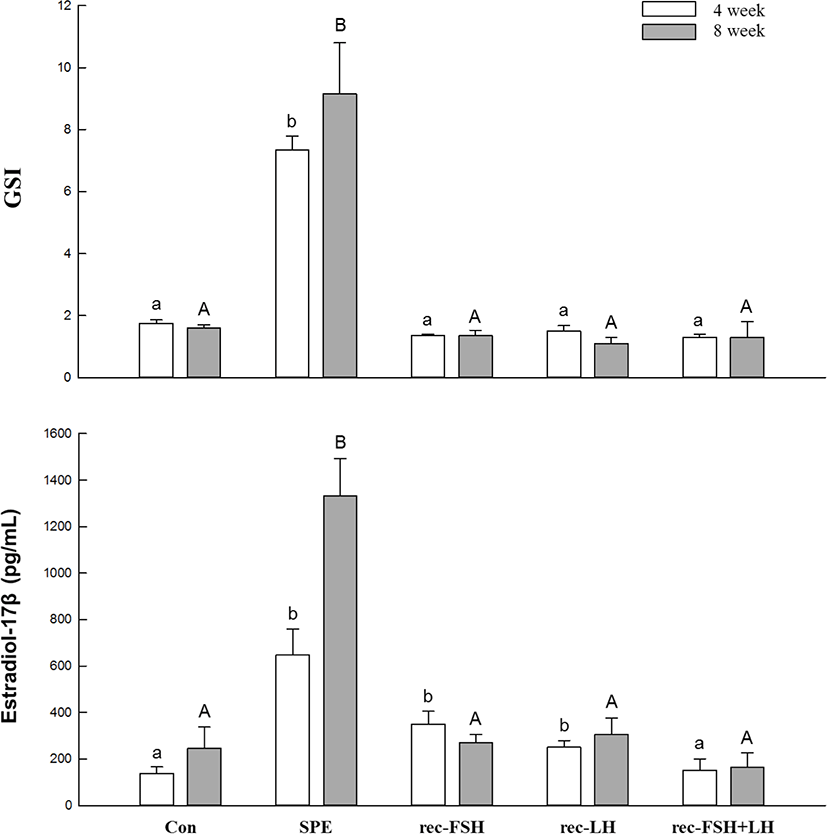
The changes in plasma T, 11-KT levels, and GSI according to rec-GTH and hCG administration are shown in Fig. 3. After 3 and 8 weeks of hCG administration, both plasma T and 11-KT levels were significantly increased compared to those in the control group. Furthermore, the GSI in the hCG-treated group was higher than that in the control group. However, it should be noted that GSI and hormone levels were not different from those in the control group for all other groups treated with rec-FSH and rec-LH.
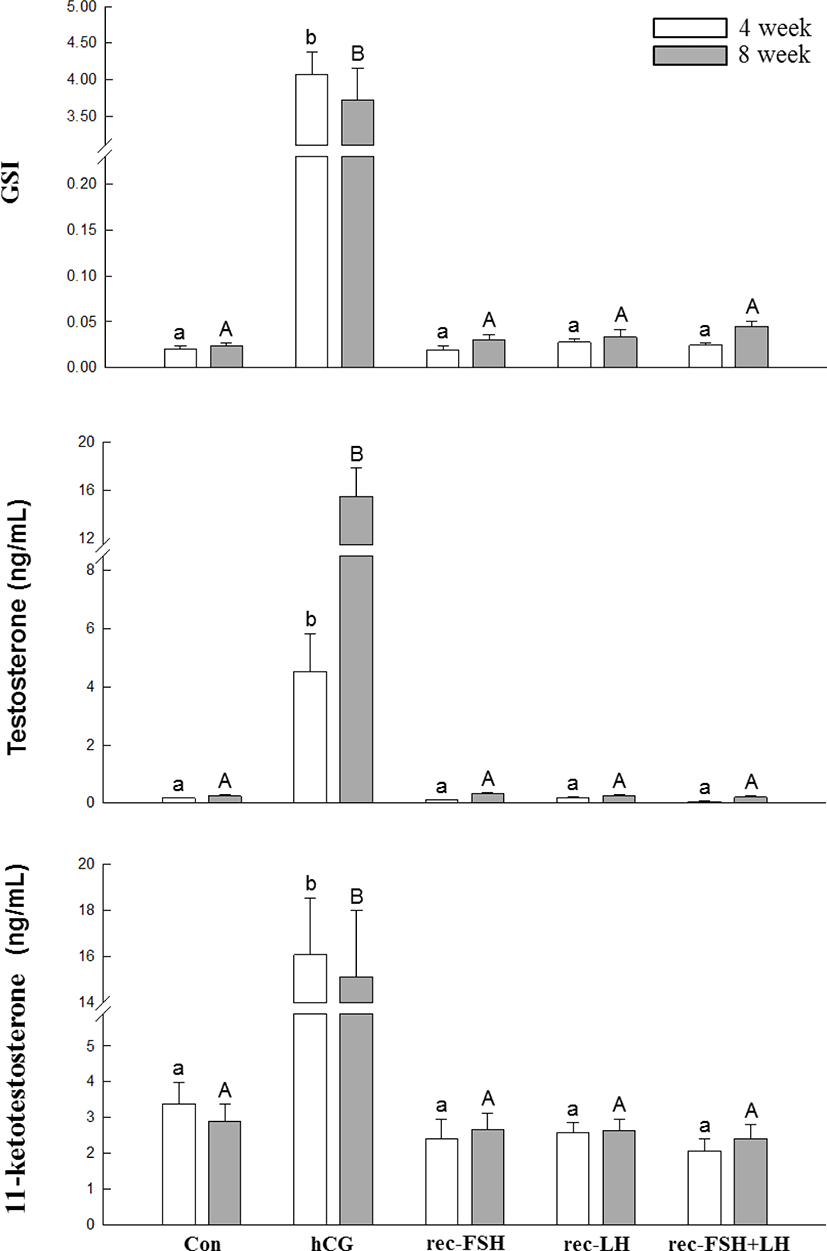
Discussion
The Japanese eel Anguilla japonica requires the administration of exogenous gonadotropins, such as SPE, for female eels, and hCG, for male eels, for the artificial induction of gonadal maturation. However, the eggs obtained thus far through exogenous hormone treatment have been known to be of poor quality, which results in lowering of fertility rates and induction of malformations (Shin, 2004). Furthermore, the milt obtained by injection of hCG showed individual differences in quantity and quality. To overcome these problems, species-specific recombinant GTHs were produced using silkworm larvae (Choi et al., 2016; Kim et al., 2008), mammalian cells (Molés et al., 2011), and Pichia pastoris (Kasuto & Levavi-Sivan, 2005) as a bioreactor, and the biological activity of these gonadotropic reagents was evaluated and improved (Ohta et al., 2017). It is expected that species-specific GTHs of eels may induce a more efficient method of hormonal manipulation in the aquaculture of Japanese eel. In this study, we produced rec-GTHs from CHO-S cells and evaluated their bioactivity both in vivo and in vitro.
The in vitro results of this study confirmed that rec-GTHs affected early ovarian development. When an immature ovary was treated with rec-FSH or rec-LH, E2 levels increased compared to the control. In vertebrates, including teleosts, estradiol-17β (E2), which acts as a major steroid in vitellogenesis, promotes vitellogenin (Vtg) gene transcription, promotes egg yolk formation in oocytes, and increases during the vitellogenic phase of ovarian development (Nagahama, 1994). Furthermore, E2-induced Vtg expression in the liver of eels has also been demonstrated both in vitro and in vivo (Kazeto et al., 2011). Therefore, it is suggested that rec-GTHs may play a positive role in early ovarian development in female eels through in vitro ovarian E2 production. In contrast, there were no clear differences between the effects of rec-FSH and rec-LH on E2 production in early ovarian tissue. Our previous study (Kim et al., 2016) showed that rec-LH treatment had a positive effect on the induction of final maturation of eel oocytes. The potency of LH is considered more effective than that of FSH in oocyte maturation.
Although the in vitro effects of rec-GTHs on the sexual maturity of female eels were revealed in this study, there was no obvious in vivo effect of rec-GTHs on maturation induction in male and female eels. After 4 weeks of rec-GTH administration, the plasma E2 levels were higher than those in the control; however, no significant change was observed after 8 weeks of treatment. Furthermore, rec-GTHs exhibited slight effects on gonadal development. Similarly, the effects of hCG administration on GSI and plasma hormone concentrations in male eels were significantly higher than those in the control, whereas the effects of rec-GTH administration on the maturity of male eels were not apparent. The results of this study are similar to those from the study that analyzed the biological activity of recombinant GTHs in Japanese eels using Drosophila S2 cells (Kazeto et al., 2008). Kazeto et al. (2008) reported that rec-GTHs induced biological activity in vitro but did not induce definite activity in vivo. This difference between in vitro and in vivo bioactivities is probably due to the residual time of recombinant hormones in the blood. In other words, the absence of in vivo bioactivity of rec-GTHs may be the result of the rapid clearance of the hormone in vivo. Legardinier et al. (2005) demonstrated that rec-GTHs have a rapid metabolic clearance in the blood; therefore, sufficient terminal sialylation of their carbohydrate chains is required to extend their half-life in blood. For this reason, rec-GTH bioactivity in female eels in this study was observed after 4 weeks of GTH administration but not at 8 weeks of administration. Therefore, it is necessary to confirm the half-life of rec-GTHs in the blood and to glycosylate the rec-GTHs with more complex and sialylated N-glycans to induce bioactivity in vivo and to evaluate the in vitro biological activity of the rec-GTHs in male eels.
In the present study, we demonstrated that rec-GTHs clearly have positive effects on the sex maturation of immature ovarian cultures in vitro. However, the results showed no obvious effects in males in vitro, and in both genders under in vivo conditions. Thus, we suggest that further studies are needed on in vitro maturity induction of male eels, complement rec-protein production systems, and additional glycosylation of rec-hormones to extend the half-life of rec-GTHs in the blood.