Introduction
Warming and disease outbreaks are two of the major challenges for tropical aquaculture (Naylor et al., 2021). This is because warming, particularly marine heatwaves (MHWs) can promote the growth of bacterial pathogens (Zhou et al., 2008) while weakening the immune responses of aquatic species (Altizer et al., 2013; Chen et al., 2015; Dinh et al., 2022), resulting in increased mortality and reduced growth rates (Le et al., 2020). Consequently, it has been predicted that by 2050 warming can reduce up to 40% of aquaculture species in tropical regions (Free et al., 2019; Froehlich et al., 2018; Oyinlola et al., 2020).
Heat stress not only increases pathogen prevalence but also induces oxidative stress in fish by generating an excess of reactive oxygen species (ROS), which can cause damage to proteins, lipids, and nucleic acids, ultimately leading to impaired physiological functions (Baker et al., 2020; Madeira et al., 2013). Under conditions of thermal stress, the antioxidant capacity of fish becomes crucial for mitigating cellular damage and maintaining normal biological functions. In this context, vitamin C is known to play an essential role as an antioxidant, helping to scavenge ROS and maintain cellular integrity (Dawood & Koshio, 2018; Tewary & Patra, 2008).
To alleviate the impact of warming, many studies have shown that vitamin C supplements in diets (hereafter referred to as vitamin C) can improve animal health and immunity against bacterial pathogens (Dawood & Koshio, 2018). Vitamin C plays a key role in protecting cells against the effects of free radicals and enhancing immune responses (Barros et al., 2015; Dawood & Koshio, 2018). Specifically, it contributes to the regeneration of other antioxidants such as vitamin E, promotes collagen synthesis which is vital for wound healing, and enhances the production and activity of immune cells, including neutrophils, macrophages, and lymphocytes. These immune-enhancing properties are particularly important for fish exposed to stressful conditions such as elevated temperatures, which are known to suppress the immune system and increase susceptibility to diseases (El‐Sayed & Izquierdo, 2022).
However, fish are not unable to synthesize vitamin C because a lack of the enzyme L-gulonolactone oxidase and they, therefore, have to obtain vitamin C from the diet (Dawood & Koshio, 2018). In the case of heat stress, vitamin C has been reported to mitigate the harmful effects of elevated temperatures by enhancing stress tolerance and reducing cortisol levels, a primary stress hormone in fish. This hormone regulation helps to maintain homeostasis, thereby reducing the physiological disturbances caused by thermal stress. Moreover, vitamin C has been shown to upregulate the expression of heat shock proteins (HSPs), which are molecular chaperones that assist in protein folding and protect cells against heat-included damage (Dawood & Koshio, 2018; Herrera et al., 2019). This mechanism further reinforces the hypothesis that vitamin C supplementation may be advantageous for fish exposed to MHWs.
Among farmed fish in tropical regions, cobia is one of the most significant species, with an annual production of 40,000–50,000 metric tonnes (Benetti et al., 2021). Cobia is valued in aquaculture for its rapid growth rate and high-quality flesh. However, it is also predicted to be highly susceptible to climate change, particularly due to its sensitivity to elevated temperatures, which can negatively affect growth and immune function (Dong et al., 2015; Nguyen et al., 2019). Given these vulnerabilities, it is crucial to explore strategies to enhance cobia’s resilience to thermal stress and maintain productivity under changing environmental conditions. Therefore, investigating the effects of vitamin C supplementation on cobia juveniles is worthwhile, given its potential to alleviate the impacts of heat stress by improving health, enhancing immune response, and promoting overall resilience against adverse conditions.
In this study, we hypothesized that vitamin C supplementation could mitigate the effects of MHWs on cobia (C. canadum) juveniles by improving the health status and immune responses, thereby improving survival and production. Since cobia cobia is a key marine finfish farmed in Vietnam’s coastal regions (Benetti et al., 2021), understanding the potential benefits of vitamin C under heat stress conditions is crucial for ensuring sustainable aquaculture in the face of climate change.
Materials and Methods
In accordance with the National Regulations for the Use of Animals in Research in Vietnam, specifically The Law of Animal Husbandry of Vietnam (2018) and Government Decree 32/2006/ND-CP on Management of Endangered, Precious, and Rare Species of Wild Plants and Animals, experiments involving cobia are exempt from ethical approval requirements. However, the authors adhered to best practices based on the EU Directive 2010/63 on the use of animals in research.
Juvenile cobia (Rachycentron canadum) were bred at the Cam Ranh Centre for Tropical Marine Research and Aquaculture, Nha Trang University, Vietnam. Initially, the fish (BW, body weight = 6.95 ± 1.60 g, TL, total length = 12.06 ± 0.68 cm) were acclimated to laboratory conditions for two weeks, following a protocol similar to described in our previous studies (Le et al., 2021; Nguyen et al., 2019). Ten randomly collected juveniles per group were placed into one of 18 rectangular fiberglass tanks (L × W × H = 0.8 × 0.5 × 0.5 m), each filled with 200 L of seawater. The tanks were divided randomly into two temperature treatments: a control group at 30°C and an extreme temperature of 34°C, with 9 tanks assigned to each treatment. The selection of 34°C as an extreme temperature was based on previous study and preliminary report that indicated that temperatures above 32°C cause significant physiological stress in cobia (R. canadum). For example, Sun & Chen (2014) reported that extreme temperatures between 27°C and 33°C led to increased nitrogen excretion and reduced growth performance, suggesting that temperatures beyond 33°C may induce considerable stress. Similarly, Sun et al. (2006) also demonstrated that temperatures exceeding 32°C had negative effects on nutrient absorption and growth in cobia. Nguyen et al. (2019) specifically reported the effects of extreme temperature (34°C) on juvenile cobia and showed significant impacts on feed intake and neuropeptide expression related to appetite regulation, indicating higher stress at this temperature compared to the normal sea temperature of 30°C in Vietnam. Furthermore, Le et al. (2020) reported that 34°C, in the context of MHWs, significantly affected growth, metabolism, and overall health of cobia. Collectively, these findings justify the use of 34°C as an extreme temperature in the present study to evaluate its effects on cobia in an aquaculture environment. Each tank was designed with continuous aeration to maintain dissolved oxygen at saturation levels.
Cobia juveniles were cultured at one of six conditions with two temperatures (30°C and 34°C) × vitamin C levels (0, 200, or 400 mg/kg diet) with three replications per treatment. The control temperature of 30°C shows the average seawater temperature in aquaculture ponds at the study location (Nguyen et al., 2019). This temperature has been indicated the promote the fastest growth in cobia juveniles (Le et al., 2020; Nguyen et al., 2019). The temperature of 34°C simulated an extreme condition, which frequently occurs from February to October (Le et al., 2020).
The basal diet was formulated according to the nutritional requirements for marine fish. The detailed feed content is presented in Table 1. To the basal diet, ascorbic acid (vitamin C) powder (acid ascorbic vitamin C 99%-E300, DSM/Shandong Luwei, Zibo, China) was added to create experimental feeds containining 100 and 400 mg vitamin C per k of diet. The ascorbic acid levels in the diet were confirmed using reverse-phase high-performance liquid chromatography (HPLC). The relative composition of the experimental diets, following vitamin C supplementation, are also provided in Table 1. The diets were stored in a refrigerator at 4°C.
Vitamin premix: Vitamin A, 1,000,000 UI; Vitamin D3, 300.000 IU, Vitamin C monophosphate 10.000 mg; Vitamin B6, 500 mg; Vitamin B2, 320 mg; Vitamin B12, 5 mg (Provimi Vietnam, Bien Hoa City, Vietnam). Mineral premix: Zn (ZnO), 4.750 mg; Mn (MnSO4.H2O), 1.900 mg; Mg (MgO), 1.050 mg; Ca (CaHPO4.2H2O), 0.8%; Co (CoCO3), 47.5 mg; Se (Na2SeO2), 47.5 mg (Provimi Vietnam).
The feeding trial duration was 10 weeks. Juveniles were fed two times a day at around 08:00 and 17:00. The feeding regimen consisted of 5% of the fish BW per day for the first 5 weeks, following by 3% for the final five weeks. The rearing conditions were maintained with salinity at 29 ± 3.1 ppt, pH between 7.6 and 7.8, unionized ammonia nitrogen below 0.05 mg/L, and dissolved oxygen levels not lower than 6.0 mg/L. To maintain ammonia levels within safe limits, we regularly monitored water quality parameters, including ammonia concentration, throughout the experimental period. Partial water changes were conducted as needed between the scheduled seawater refills every two weeks to prevent ammonia buildup. Additionally, biofilters were used to enhance nitrification and maintain stable water quality. Every two weeks, juveniles were collected from the tanks and weighed as groups. The tanks were cleaned and refilled the fresh filtered seawater before groups of fish were returned to the same tanks.
At the end of the experiment, we measured the specific growth rate (SGR), body indices, proximate composition, blood and immune parameters in similar methods as described in (Le et al., 2021).
At the end of the experiment, three fish from each experimental tank were collected to determine growth, body indices, hematological parameters, immune response, and proximate composition. An additional seven fish per tank were used for another test. Prior to handling for growth and physiological parameters, the fish were anesthetized using monophenyl ether glycol solution at a concentration of 150–200 ppm. Samples were collected as follows:
Blood samples were collected from each fish using a 1-mL syringe from the caudal vein. These samples were used to determine lysozyme activity (see Serum lysozyme activity Section) and hematological parameters (see Hematological parameters Section). BW was measured with a laboratory scale with a range of 0–200 g and an accuracy of 0.01 g. The SGR (%/day) was calculated as 100 × (Ln Wt – Ln Wi) / t, where t is the experimental duration in days. Feed conversion ratio (FCR) was determined by calculating the amount of feed consumed (g, dry weight) per gram of wet weight gained. The protein efficiency ratio (PER) was calculated as the weight gain (g) divided by protein intake (g). Protein productive value (PPV) was determined as the retained protein (g) relative to the consumed protein (g), using the formula: PPV = (final protein content – initial protein content) × (protein consumed)–1.
The fish were then dissected to remove the visceral organs and liver for measuring body indices, including liver and visceral weights (g), the viscerosomatic index (VSI), and hepatosomatic index (HSI). VSI (%) was calculated as percentage of viscera weight (g) relative to BW (g), while HSI (%) was determined as the percentage of liver weight (g) relative to BW (g). The liver and body samples from each treatment group were pooled for vitamin C concentration analysis, as described in Ascorbic acid assay Section. Finally, the fish bodies were used to determine proximate composition (see Proximate composition analyses Section).
Blood was collected from the caudal vein using a 1-mL syringe and pooled into 2 mL centrifuge tubes for lysozyme activity analysis. These tubes were kept at 4°C overnight and then centrifuged at 400×g for 10 min. The resulting supernatant sera were transferred into new tubes and stored at –80°C until further analysis. A volume of 25 µL of each serum samples was placed into three wells of a titer plate (in triplicate). For the control, 25 µL of hen egg-white lysozyme (HEWL; Sigma-Aldrich, St. Louis, MO, USA) in 0.1M phosphate/citrate buffer at concentrations of 20, 10, 5, 2.5, 1.25, 0.625, and 0.3125 µg/mL in 0.1M phosphate/citrate buffer solution with 0.09% NaCl, pH 5.8 was pipetted into six wells of the titer plate. Then, 175 µL of Micrococcus lysodeikticus 0.075% suspension was added to each wells, quickly mixed, and absorbance was measured at 450 nm using a microtiter reader. Changes in suspension turbidity (absorbance) in each well were recorded for 5 min at 30 s intervals. Lysozyme activity of the samples was calculated relative to the control HEWL solutions.
Blood samples were collected from the caudal vein of each fish using a heparin-rinsed syringe and transferred to individual sterilized vials kept at 4°C. These samples were then analyzed for white blood cells (WBC), red blood cells (RBC), haematocrit (HTC), and hemoglobin (Hb) using the Sysmex XT-1800i Blood Analyzer (Sysmex Corporation, Hyogo, Japan). Plasma triglycerides and plasma protein levels were measured using a 600i D×C General Chemistry Analyzer (Thermo Scientific, Waltham, MA, USA).
Liver, diet, and whole-body samples were collected from three fish per tank and pooled for vitamin C concentration analysis. Approximately 2.0–5.0 g of the experimental diet, fish liver, or whole body was finely ground and placed into 100 mL e-tubes. Fifteen mL of 0.05 M KH2PO4 buffer (adjusted to pH 2.5 with phosphoric acid) was added, and mixture was vortexed for 1–2 min. The e-tubes were then centrifuged at 15,000 rpm for 5–10 min. The supernatants were filtered through 0.2 µm Whatman paper 1–2 times before analysis. For vitamin C analysis, 10 µL of the sample was injected into the column oven L-2300 set at 30°C. Vitamin C content in the fish liver, whole body, or experimental diets was determined by reverse-phase HPLC using an Intersil ODS-3 C18 column (GL Sciences, Tokyo, Japan; 5 µm, 250 × 4.6 mm). The mobile phase was an aqueous solution of 0.05 M KH2PO4 (pH 2.5) at a flow rate of 1 mL per min, with the effluent detected and monitored by a UV detector at a wavelength 245–257 nm.
Body protein content was analyzed using a Kjeltec Auto 1030 analyzer (Foss Tecator, Höganäs, Sweden). Lipid content was determined by petroleum ether extraction using a Soxhlet extraction system. Moisture content was measured by drying samples at 105ºC in an oven (Thermotec 2000, Contherm Scientific, Lower Hutt, New Zealand). Ash content was determined by combustion at 550°C for 24 h in an electric furnace (Carbolite Gero, Sheffield, UK).
We employed general linear models to test fixed effects of vitamin C, temperature and their interactions on all response variables. Survival data were log(x + 1)-transformed prior to analysis. All statistical analyses were performed using Statistica 12 (TIBCO Software, Palo Alto, CA, USA).
Results
Survival was 5% and 8% higher at the vitamin C concentrations of 200 and 400 mg/kg, respectively, than in the control (p = 0.035, Table 2 and Fig. 1A). Survival was reduced at 34°C, but only in the control diet, not in vitamin C supplementary treatments (vitamin C × temperature interaction, p = 0.035, Table 2 and Fig. 1A).

The SGR was reduced by 5% at 34°C compared to 30°C (temperature effect, p = 0.09, Table 2 and Fig. 1B). The SGR increased by 4% and 9% at the vitamin C concentration of 200 and 400, respectively (vitamin C effect, p = 0.003, Table 2 and Fig. 1B). The effect of vitamin C on SGR was independent on temperatures (p = 0.625, Table 2).
The PPV and PER values were reduced by 22% and 11% at 34°C (temperature effect, p = 0.026 and p = 0.011, respectively; Table 2 and Fig. 1D). Vitamin C supplements substantially increased PER and PPV (vitamin C effects, p = 0.003 and p = 0.010, respectively; Table 2, Fig. 1C and 1D), but these effects were more substantial at 30°C than at 34°C (temperature × vitamin C interaction, p = 0.482 and p = 0.885, respectively; Table 2, Fig. 1C and 1D).
Overall, elevated temperature resulted in reductions in protein (p = 0.026) and lipid content (p = 0.005) while not affecting the ash (p < 0.001) and moisture content (p = 0.002) of cobia juveniles (Table 2 and Fig. 2A–2D). Vitamin C supplements increased protein content (p = 0.010) while decreasing the lipid (p = 0.797) and ash content (p = 0.363; Table 2 and Fig. 2A–2C). The effects of vitamin C on the protein, lipid and ash content were independent of temperature (p-values for vitamin C × temperature interaction: protein = 0.885, lipid = 0.726, ash = 0.163).
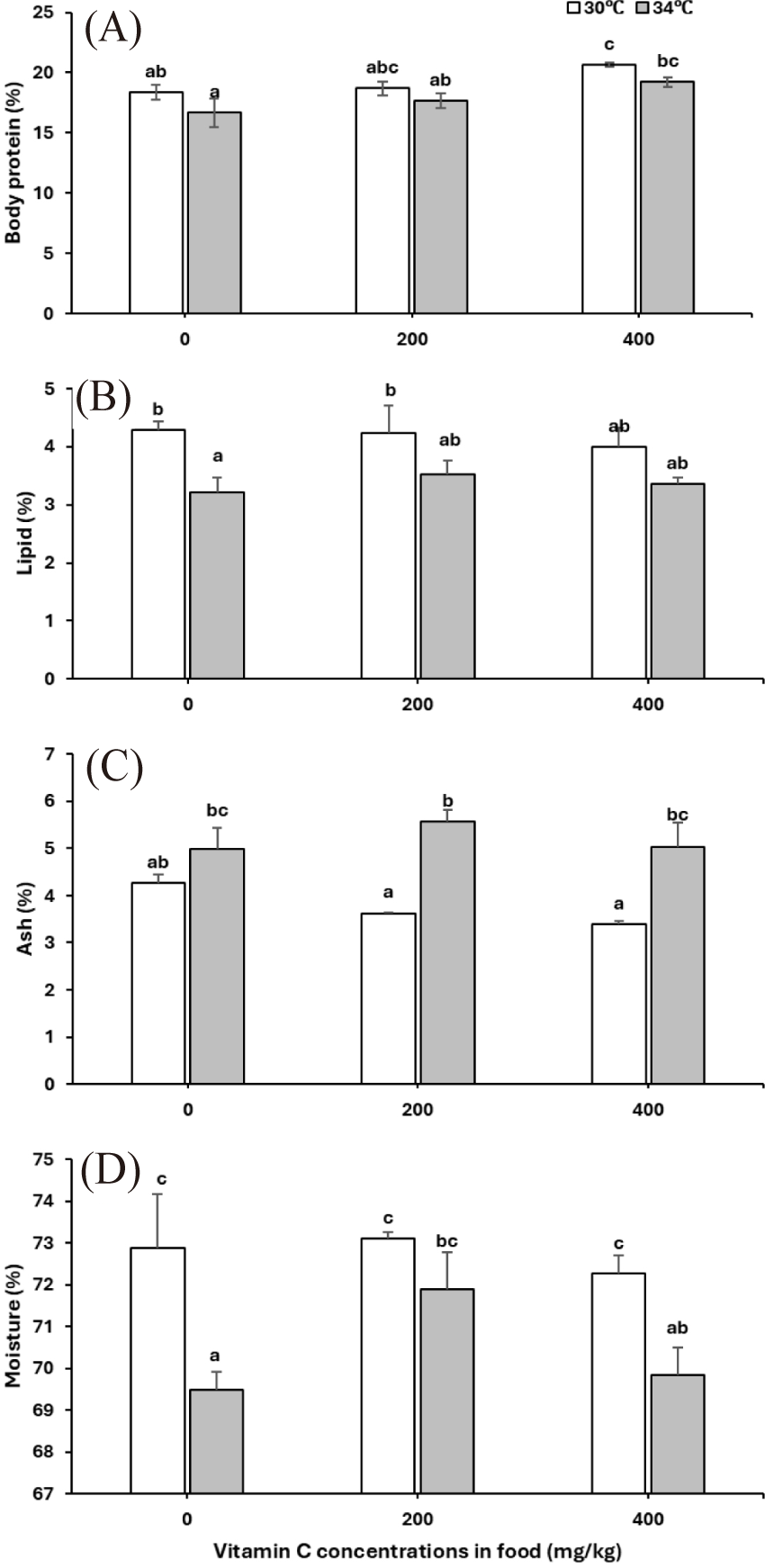
The weight of the liver (p = 0.11) and viscera (p = 0.003) of cobia juveniles was reduced under warming but increased with vitamin C supplements (liver: p = 0.432, viscera: p = 0.002; Table 2, Fig. 3A and 3B). The effect of temperature and vitamin C was independent of one another (p-values for vitamin C × temperature interaction: liver = 0.125, viscera = 0.321). There were no effects of temperature (HSI: p = 0.415, VSI: p = 0.119) or vitamin C (HSI: p = 0.144, VSI: p = 0.115), nor the interaction of both factors (HSI: p = 0.042, VSI: p = 0.008) on the HSI and VSI of cobia juveniles (Table 2, Fig. 3C and 3D).
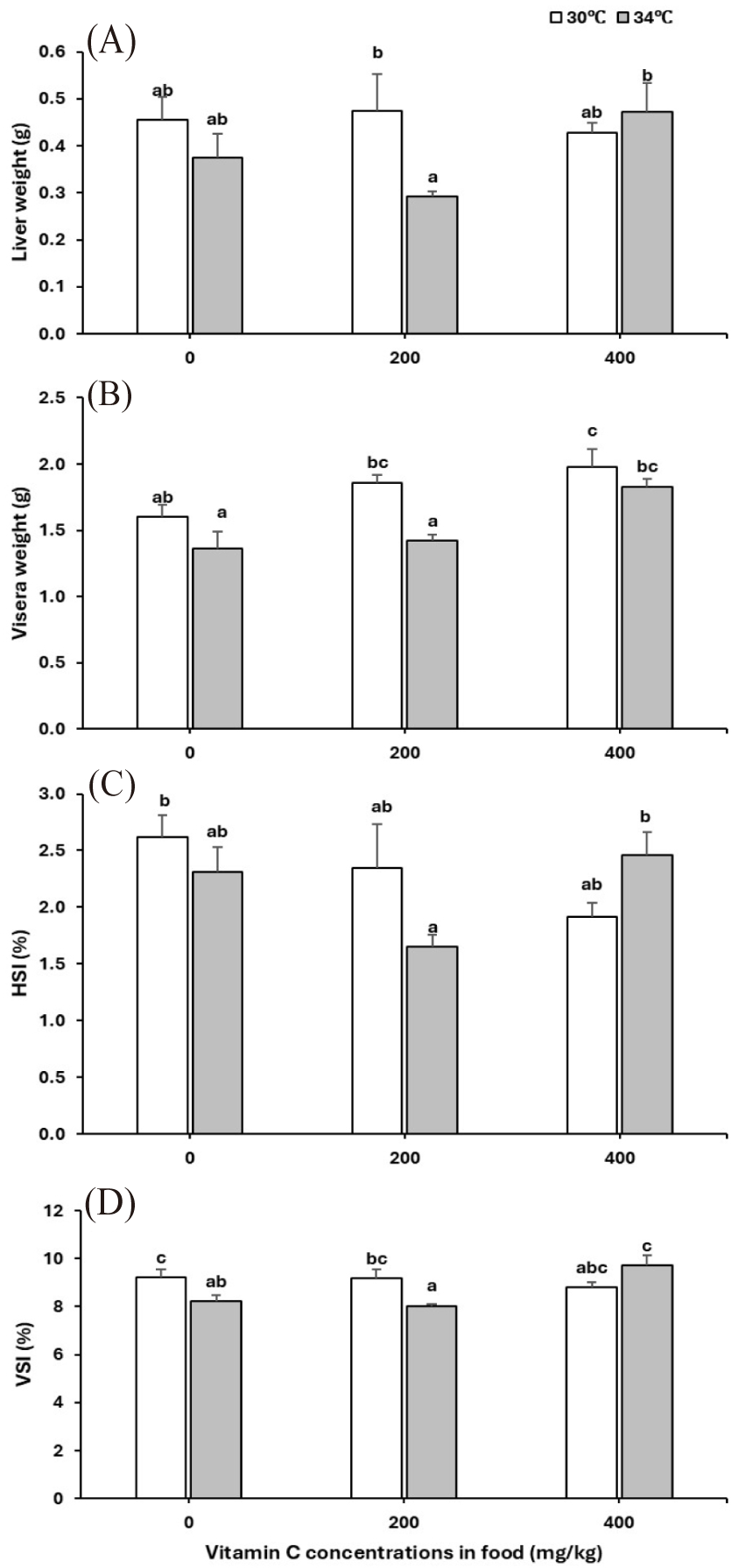
WBC, RBC, Hb, HCT levels and lysozyme activity were significantly reduced at 34°C (WBC: p < 0.001, RBC: p < 0.001, Hb: p = 0.08, HCT: p = 0.716, lysozyme: p = 0.037), while increased in juveniles fed with vitamin C supplements (WBC: p < 0.001, RBC: p = 0.014, Hb: p < 0.001, HCT: p = 0.057, lysozyme: p = 0.012) (temperature and vitamin C effects, Table 2 and Fig. 4A–4E). The increased Hb level in fish fed with 400 mg vitamin C per kg diets was stronger at 30°C than at 34°C (temperature × vitamin C interaction, p = 0.002, Table 2 and Fig. 4C).
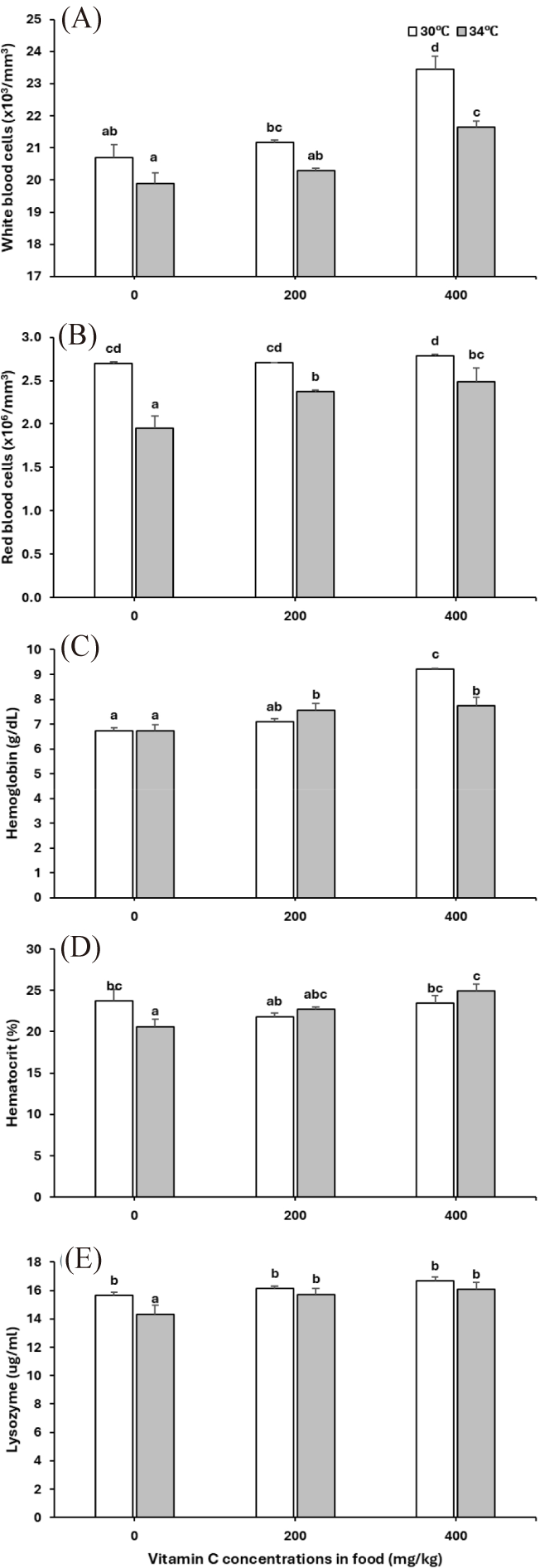
Similarly, the ascorbic acid concentrations in the liver (p = 0.024) and fish body (p = 0.006) were reduced at 34°C while increased in fish fed with vitamin C supplements in the diet (p < 0.001 for both liver and body; Table 2, Fig. 5A and 5B). The effect of vitamin C on the increased ascorbic level in the liver was less pronounced at 34°C than at 30°C (vitamin C × temperature interaction, p = 0.016 for liver, p = 0.025 for body; Table 2, Fig. 5A and 5B).
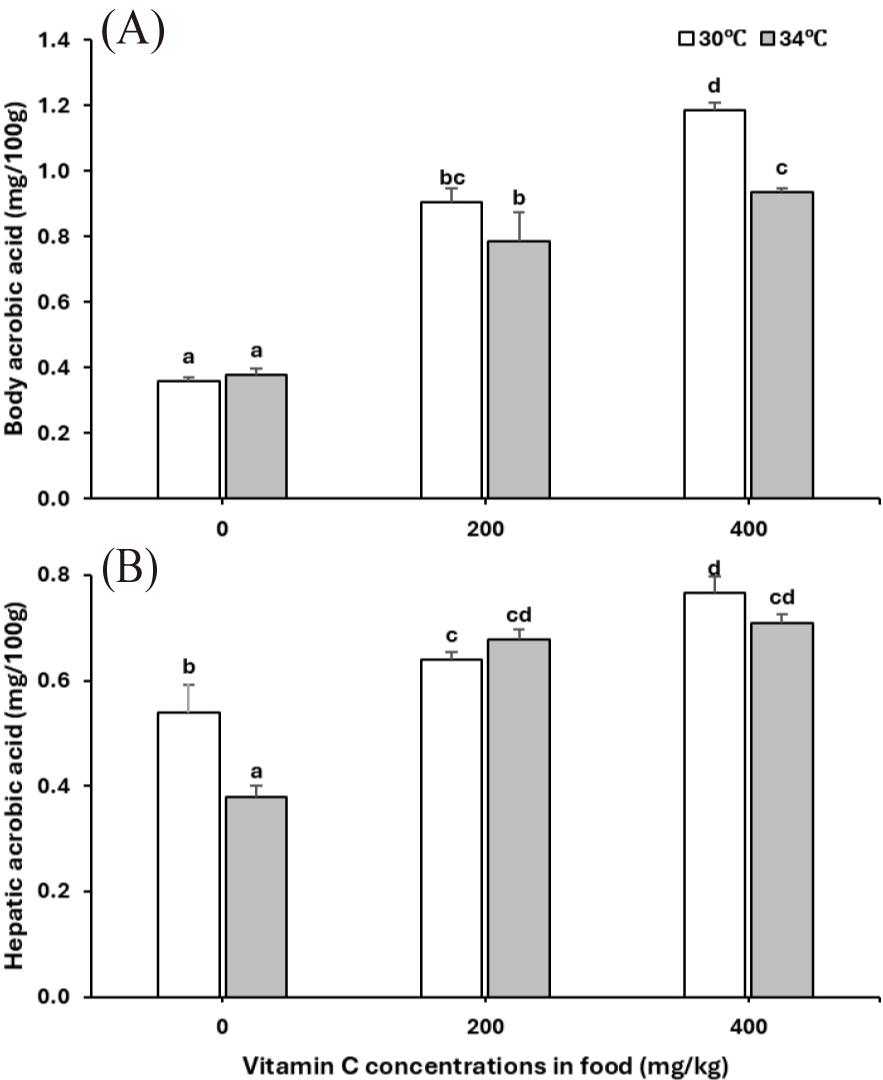
Discussion
All productive parameters of cobia juveniles were strongly reduced at 34°C. The reduced juveniles survival was similar to the findings in previous studies on Waigieu seaperch (Psammoperca waigiensis; Le et al., 2021), including cobia (Le et al., 2020; Nguyen et al., 2019). Temperature may cause mortality in fish due to physiological impairment or dysfunction. A reduced RBC, Hb may cause inefficient oxygen delivery while the cellular oxygen requirement may increase to meet higher metabolic rates under elevated temperatures (Angilletta et al., 2004). The Hb levels have been shown to negatively correlate to fish survival (Hamre et al., 1997). Temperature extremes can also cause instability of the cell membrane (Somero, 1995), increase oxidative stress, and depress the upregulation of the HSPs (Dinh et al., 2016).
Although extreme temperatures often results in a faster growth rate and development of many temperate species (Deutsch et al., 2008), it tends to inhibit the growth rate of tropical fish species such as spinefoot rabbitfish (Siganus rivulatus), Waigieu seaperch (P. waigiensis; Le et al., 2021) and cobia (R. canadum; Le et al., 2020, 2021; Nguyen et al., 2019). In the present study, a reduced SGR of cobia juveniles was associated with a higher FCR and a lower PER and PPV, suggesting lower efficiency of digestion and nutrient assimilation, while the energy demand for basal maintenance was likely higher due to increased metabolism (Killen et al., 2016). It is further reflected in multiple indicators such as lowered lipid content, liver and viscera weights, HSI and VSI, suggesting that the level of energy reserves in cobia juveniles were reduced by extreme temperatures (Chellappa et al., 1995). A lowered protein and lipid content also indicated a lower flesh quality of fish in extreme temperatures.
In a similar way, 34°C caused strong reductions in all blood and immune parameters, suggesting that the health status of cobia juveniles was strongly impacted in extreme temperatures (Le et al., 2021). Reduced blood parameters may result in a lower capacity to deliver oxygen to meet cellular metabolism under elevated temperature (see above). A reduced immune response such as lowered lysozyme activity, and body and hepatic ascorbic acid suggests an increased vulnerability of cobia juveniles to pathogenic agents, which are likely more prevalent under warming conditions (Islam et al., 2022).
A positive effect of vitamin C on cobia juvenile SGR observed in the present study can be attributed to several underlying mechanisms. Vitamin C is known to play a crucial role in enhancing nutrient assimilation and metabolic processes that contribute to growth. Specifically, vitamin C aids in improving the efficiency of FCR, PER, and PPV. These parameters indicate the efficiency of converting feed into body mass, suggesting that vitamin C improves the digestive and metabolic capacity of cobia juveniles to utilize nutrients effectively. A similar result has been observed in other marine fish species largemouth bass or Chu’s croaker (Zhou et al., 2008, 2012; Zou et al., 2020). From a mechanistic perspective, vitamin C acts as an essential cofactor in collagen synthesis, which is critical for tissues, thereby contributing to improved growth performance and overall health. Furthermore, vitamin C aids in regenerating other antioxidants, such as vitamin E, and supports alleviate oxidative stress, which can otherwise dislocate metabolic processes, protein synthesis, and nutrient assimilation under extreme temperatures (Dawood & Koshio, 2018). By scavenging ROS, vitamin C decreases cellular damage, enabling fish to allocate more energy toward growth instead of repair, ultimately enhancing growth performance.
The effect of vitamin C on feed utilization is also obvious from the rise in PER and PPV values in cobia juveniles supplemented with vitamin C. Vitamin C plays a role in maintaining the activity of digestive enzymes and ensuring optimal gastrointestinal health, which leads to better nutrient absorption and assimilation (Cai et al., 2022). By reducing oxidative stress in the gastrointestinal tract and maintaining a healthy gut environment, vitamin C improves the digestion and absorption of dietary proteins, thereby contributing to more efficient feed utilization and conversion into body mass.
Furthermore, vitamin C had generally positive effects on the viscera weight, protein content, and blood parameters such as RBC, Hb, and HTC, advising that it can help cobia juveniles improve their health status respiration, and survival. Vitamin C supports iron metabolism and erythropoiesis, leading to increased Hb synthesis and an improved capacity for oxygen transport (Liang et al., 2017). This is particularly important under extreme temperatures, where increased metabolic rates necessitate higher oxygen demands. By supporting efficient oxygen delivery, vitamin C confirms that metabolic and growth processes can continue effectively, even under heat stress.
Regarding immune response, vitamin C has been shown to significantly enhance immunity by increasing the number of WBCs and boosting lysozyme activity. Lysozyme is a key enzyme involved in the innate immune response, providing a first line of defense against bacterial infections. Vitamin C enhances the production and activity of immune cells, including neutrophils, macrophages, and lymphocytes, thereby improving the fish’s ability to resist infections and cope with pathogen challenges, which are more prevalent under elevated temperatures (Liang et al., 2017; Zhou et al., 2012). The observed increase in WBCs count and lysozyme activities in cobia juveniles supplemented with vitamin C demonstrates the role of this vitamin in modulating immune function and providing greater resilience to disease outbreaks, which are often exacerbated by warming conditions (Islam et al., 2022). The lack of vitamin C effect on the lipid content may suggest that it had no role in lipid metabolism in cobia juveniles.
One of the most important findings in this study was that a supplementation with 200 or 400 mg of vitamin C per kg feed fully mitigated the lethal effect of 34°C on juvenile cobia. This protective effect can be understood through the mechanism of reduced oxidative damage. Under elevated temperatures, the production of ROS increases, causing oxidative stress and cellular damage, which can be lethal if unchecked. Vitamin C, as an effective antioxidant, directly scavenges these ROS, thereby reducing oxidative damage to a level below what is lethal (Cai et al., 2022; Dawood & Koshio, 2018). This mechanism ensures that the physiological functions necessary for survival, such as respiration and immune defense, are maintained, even under extreme temperature stress.
However, despite these positive effects, vitamin C supplementation did not alleviate the negative impact of heat on growth performance (e.g., SGR). This suggests that while vitamin C can reduce oxidative stress and improve health status, it may not directly influence the metabolic adjustments required for efficient nutrient utilization and protein synthesis under extreme temperatures. The latter result may relate to the insignificant interaction of vitamin C and temperature on FCR, PER, and PPV. It suggests that vitamin C cannot help cobia juveniles increase the efficiency of protein digestion and synthesis under extreme temperatures (Chen et al., 2015; Le et al., 2021). The insignificant interactive effects of vitamin C and temperature on most of the blood and immune parameters suggest that the beneficial effects of vitamin C on these parameters were not reduced by exposure to 34°C. This may be because the metabolic pathways involved in growth are fundamentally altered by temperature extremes, and vitamin C alone is insufficient to restore the optimal metabolic state required for growth.
Moreover, while vitamin C had generally positive effects on various blood and immune parameters, the lack of significant interactive effects with temperature suggests that its benefits were maintained independently of temperature stress. This implies that vitamin C consistently enhances immune parameters such as WBC count and lysozyme activity, providing a baseline level of immune support that is not compromised by elevated temperatures (Liang et al., 2017). Thus, while vitamin C can mitigate some of the adverse effects of heat stress, its role in enhancing growth performance under such conditions may be limited.
Conclusions and perspectives
The results of the current study provide a mechanistic understanding of how vitamin C supplementation can improve the health, immunity, and survival of cobia juveniles exposed to extreme temperatures, although it does not fully mitigate the effects on growth performance. Vitamin C’s role in enhancing antioxidant defense, oxygen transport, and immune function is crucial for mitigating the impacts of MHWs, which pose a significant threat to finfish aquaculture. While finfish aquaculture is one of the fastest-growing sectors in global food production (Blanchard & Novaglio, 2024) and plays an important role in global food security. However, cobia aquaculture, one of the few globally important marine-farmed fish (Pethiyagoda & Gill, 2013), is challenged by MHWs and the outbreak of bacterial diseases (Free et al., 2019; Oyinlola et al., 2020). Similar to our previous study on the Waigieu seaperch (P. waigiensis; Le et al., 2021), the results of the current study provided a mechanistic understanding of how temperature extremes could reduce the survival, and growth rate of cobia juveniles by lowering the efficiency of food utilization and protein conversion while weakening the respiratory and immune systems. Heatwaves can strongly reduce finfish production in tropical Southeast Asia, contributing to about a quarter of global farmed fish production (FAO, 2022), potentially threatening the food security of hundreds of millions of people in the region.
A simple technical solution is supplementing enough vitamin C in the fish diet can mitigate the mortality of cobia juveniles, likely as a result of increasing fish health and immunity. However, this cannot fully mitigate the effects of heatwaves on reduced growth rate and flesh quality as indicated by lowered protein and lipid content. Future studies should extend our studies to explore whether and how MHWs and vitamin C may affect finfish aquaculture at the growth out phase and determine the potential loss in the production at an industrial scale.