Introduction
Spotted scat Scatophagus argus has a high nutritional value and is among the most widely consumed fish species in Asia. The demand for fingerlings for this species’ growth-out culture is currently high due to a lack of hatchery supply. The problems that cause high mortality during this species’ hatchery stage, from fry to fingerlings, are not well understood. The suitability of the feed provided as live feed, as well as other biological and physiological characteristics of the larvae, need to be studied. In aquaculture, antibiotics are mainly used to prevent and treat diseases in fish, but they can be agents that cause the development of drug-resistant bacteria and their residues in fish meat. As an alternative safety solution, probiotics have recently been used in aquaculture to promote the growth rate of farmed fish or to inhibit the growth of pathogens (Harada & Ishikawa, 1997; Rengpipat et al., 1998). Probiotics are defined as live microbial feed additives that provide beneficial effects to fish by improving their intestinal microbial balance (Fuller, 1989) and do not change the physiology or reduce the immunity of the fish (Magouz et al., 2020). Recently, not only living cells but also dead cells and metabolites have been recognized as biological products. Probiotics are used in aquaculture by mixing them into food or introducing them into the water environment (Corre et al., 2000; Nogami et al., 1997). The use of probiotics can improve physical growth, digestive enzymes, disease resistance, and immune gene expression and reduce infection rates in fish (Monier et al., 2023). It has been observed that probiotics treatment can increase the response to antibacterial proteins and immune gene expression in shrimp larvae and juvenile fish significantly (Fraser Clark et al., 2013; Haryanti et al., 2021).
Bacteria ingested through food can colonize the surface of the digestive tract and can grow in large numbers within the digestive tract (Olafsen, 2001). In addition, bacteria added to the culture water are also considered to enter the fish’s digestive tract and grow into colonies in the digestive tract. Beveridge et al. (1991) and Matěna et al. (1995) demonstrated that Tilapia and carp can eat bacterial cells suspended in water and that fluorescently labeled bacterial cells were detected in the intestines of experimental fish. Several researchers have examined the effects of microorganisms on the digestive ability of the fish (Cahu et al., 1998; Tovar et al., 2002). Some bacteria can promote fish digestive enzyme synthesis and digestion. High digestibility is associated with high growth rates in fish. The use of digestive enzymes to support food digestion (probiotics) aims to make the metabolic process more efficient, helping the growth, survival rate, and disease resistance of fish larvae. The development of a combination of culture methods and the use of probiotics as food supplements is very promising. In this study, we clarify the effectiveness of adding probiotics to the food and rearing water environment of spotted scat after 15 days of the first-feeding stage. The experiment was performed to provide information about the solution to stimulate digestive enzyme synthesis using commercial probiotics.
Materials and Methods
The experiment was designed according to a completely randomized (CRD) method with 4 treatments and triplicates. Experimental fish were fed twice daily at 5% of body weight with commercial pellet feed of NRD 2/3 (INVE, Phichit, Thailand), containing 55% protein, and 9% lipid. Probai A (Bayer) commercial probiotics (1 × 109 CFU/g) contain heterotrophic microorganisms, including Bacillus subtilis and Bacillus licheniformis, used for feeding and/or adding to the water environment daily. The amounts of probiotics in the treatments were calculated at 1% feed daily amount used (5% × fish biomass × 1%). For the oral supplementation method, probiotics were dissolved in clean water in a small spray bottle, then sprayed, and mixed well in pelleted feed (as recommended). Then, the feed was air-dried for 30 minutes before feeding. On the contrary, after dissolving the probiotics into a small bottle, the solution was used directly in the rearing tanks for supplementation of the environmental method.
Control treatment (CT): Probiotics were not used during the experiment (probiotics were not added to food or water environments).
Oral treatment (OR): All (1%) probiotics were mixed thoroughly into shrimp feed.
Oral and Environmental treatment (OE): Probiotics were mixed into food at 0.5% and were added to the water environment at 0.5%.
Environmental treatment (EN): All (1%) probiotics were added to the water environment.
The experiment uses a rectangular plastic tank with a volume of 170 liters (78 × 50 × 43 cm) containing 150 liters of clean filtered seawater. One experimental tank was arranged with a mini pump and a separate bio-filter box with a dimension of 40 × 20 × 15 cm. In this way, water in the tank was recirculated at the flow rate of 7.5 liters per minute. 20-day-old fish spotted scat was sourced from the Center of Research, Application, and Technology Transfer of the University of Agriculture and Forestry, Hue University. Larval fish were artificially produced from the State-level project code NVQG-2023/DA.01. After acclimation, fish were stocked at 20 individuals/tank, equivalent to 133 fish/m3.
During the experiment, water temperature, dissolved oxygen (DO), and pH were maintained at 28 ± 1.5°C, 6.12 ± 0.42 mg/L, and 7.9 ± 0.34, respectively. DO was measured using a digital DO meter (Model: CE 225908) in mg/L. Water temperature was measured by using a digital thermometer (Model: CE 225908) in °C and pH was recorded by digital pH meter (Model: CE 224469). Water samples were collected every 3 days in 100 mL bottles and kept at –20°C before being filtered through a 0.2 µm filter (DISMIC-25AS, Advantec) to determine NH4-N, NO3-N, and PO4-P concentrations. An unfiltered 10 mL water sample and 2 mL reagent of NaOH + K2S2O8 for total nitrogen (TN) or 2 mL reagent of K2S2O8 for total phosphorus (TP) were digested at 120°C for 30 min, and the product (NO3-N or PO4-P) was analyzed for TN or TP (Jackson et al., 2003). All water parameters of water samples were measured by the spectrophotometric method using a continuous flow auto-analyzer (QuAAtro 2-HR, Bltec, Osaka, Japan).
10 fish/tank were randomly collected periodically on days 15, 30, and 45 to determine the length and weight. The total length of each fish was measured to the nearest 0.1 cm with an electronic digital caliper (China), and the body weight was recorded to the nearest 0.01 g using a superior mini digital balance scale (China).
The conditional factor (CF) was calculated according to the formula:
where:
BW: is the total body weight of the fish
BL: total fish body length
Specific growth rate (SGR) by length (SGRL) or by weight (SGRW) measures the percentage increase in fish weight per day and was calculated according to the formula:
where X1 is the body mass or length at measurement time t1 and X2 is the body mass or length at measurement time t2.
Survival rates (SR) (%) were calculated with the formula mentioned below:
The hepatosomatic index (HSI) was calculated using
Digestive enzymes such as amylase and trypsin were periodically determined by randomly sampling 3 individuals/tank on days 15, 30, and 45. After collection, fish were deeply anesthetized with Aqui-S® Elanco Vietnam at a concentration of 25 mL/m3, then dissected to remove the digestive tube (separated stomach and intestines) to analyze amylase and trypsin activity. After collection, the sample was washed with 0.9% NaCl saline solution and placed in a 1.5 mL Eppendorf tube. Hepatosomatic index (HSI) was only determined once at the end of the experiment.
The separate specimens of the intestine and stomach were homogenized and then centrifuged at 4,000 rpm at 4°C for 30 minutes to remove lipids and tissue debris. The supernatant was separated for enzyme activity analysis and was immediately kept in Eppendorf at −30°C for later analysis. All enzymatic activity quantifications were carried out in triplicates right after the extraction.
Trypsin activity was determined according to the method of Taoka et al. (2007). Specifically, 200 μL of the enzyme extract was added to 800 μL of 25 mM ammonium bicarbonate buffer (pH 7.8) and 1 mM of N-α-benzoyl-L-argine p-nitroanilide hydrochloride solution and incubated at 25°C for 30 minutes. Sample adsorption was measured at 410 nm. Trypsin activity was determined as the difference in adsorption between the enzyme extract and the control per 1 mg of protein in the extract.
Amylase activity was assayed according to the method of Nolasco-Soria (2021). Briefly, 375 μL of the crude enzyme solution was mixed with a 20 mM phosphate buffer containing 10 mM NaCl (pH 6.9) and incubated at 25°C for 30 minutes. The adsorption solution was measured at 540 nm. One unit of amylase activity was defined as 1 μg glucose released per minute under assay conditions.
The total protein concentration in the extract was determined using the Bradford Test kit (Thermos Fisher Scientific, USA) with bovine serum albumin as the standard. Enzyme activity was measured by adsorption using a UV-vis spectrophotometer (Zuzi 4111RS, Romania).
All experiment data was compared for statistically significant differences between treatments in terms of weight, length, survival rate, fish growth rate, and enzyme parameters using “One Way ANOVA” with Tukey’s post-hoc test. Computational analysis was run by SPSS 16.0 software at 5% significance level (p < 0.05).
Results
The environmental parameters in the rearing system during the experiment were less volatile and fluctuated within a range of the optimal for fish growth. Fish were raised in a recirculated system so the water environment seems to be consistent among the treatments: temperature 28.6 ± 1.35°C, pH 7.81–8.32, DO 6.12 ± 0.34; salinity 20 ± 1.25‰. The concentrations of NO3-N 1.24 ± 0.46 mg, NH4-N 0.16 mg/L, and PO4-P (mg/L) gradually increased over experimental time but were still within the normally acceptable range for fish growth. Similarly, total nitrogen (TN) and total phosphorus (TP) ranged between 0.40–1.26 mg/L and 0.05–0.25 mg/L, respectively. According to Sarkar et al. (2006), the concentrations of inorganic nutrients in a culture system are high or low depending on the density of algae and sunlight intensity. This study was conducted in an indoor system, so the density of algae was very low, and sunlight was controlled, so the fluctuation of nutrients in inorganic form was very low.
There were no differences in growth performance among the groups of the experiment as presented in Table 1. Fish survival showed 100% for all treatments throughout the culture period. It can be easily seen that the average weight and length of fish after 45 days were the highest in the OR treatment with values of 4.72 ± 0.03 g and 4.84 ± 0.05 cm, respectively (p < 0.05). Moreover, higher growth in weight was also recorded between OE or EN treatments and control treatment. Although, there were no significant differences in fish growth between OE and EN treatment (p > 0.05). However, the growth rate in length and weight was significantly different between the probiotic treatments and control treatment, but this value also did not show a significant difference among the experimental treatments (p > 0.05). In addition, the results also show that the supplemental methods of probiotics did not affect the growth and survival rates of spotted scat at the fingerling stage.
Conditional factor shows differences between probiotic treatments and control treatment for all sampling occasions (p < 0.05), but these values had no significant differences among experimental treatments (p < 0.05) (Table 2). However, HSI coefficient showed significant differences between EN treatment and other remaining treatments (p < 0.05).
Amylase (Fig. 1A and 1B) and Trypsin (Fig. 2A and 2B) activities in the stomach and intestine increased during the culture period in all experimental treatments. After 15–45 days, only amylase activity in the stomach was observed significantly higher than in the control group (CT) (p < 0.05), while amylase in the intestine and trypsin in both the stomach and intestine were significantly higher than that of control treatment during 15–30 days (p < 0.05). Further, no significant differences in trypsin activity in both the stomach and intestine were found from day 30 to 45 (p > 0.05). Moreover, there were no significant differences in enzymatic activity in the stomach and intestine among the experimental treatments throughout the experiment (p > 0.05).
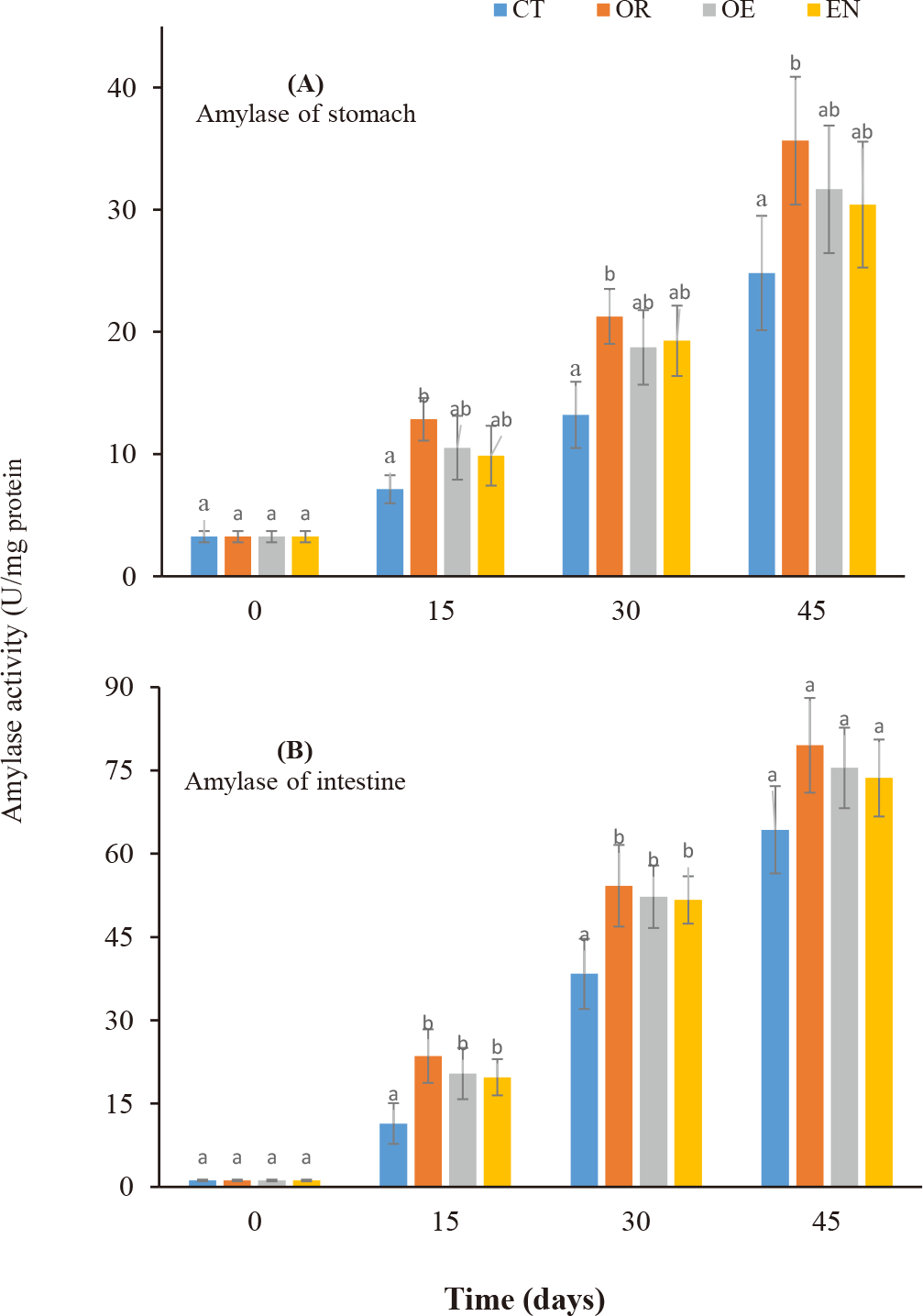
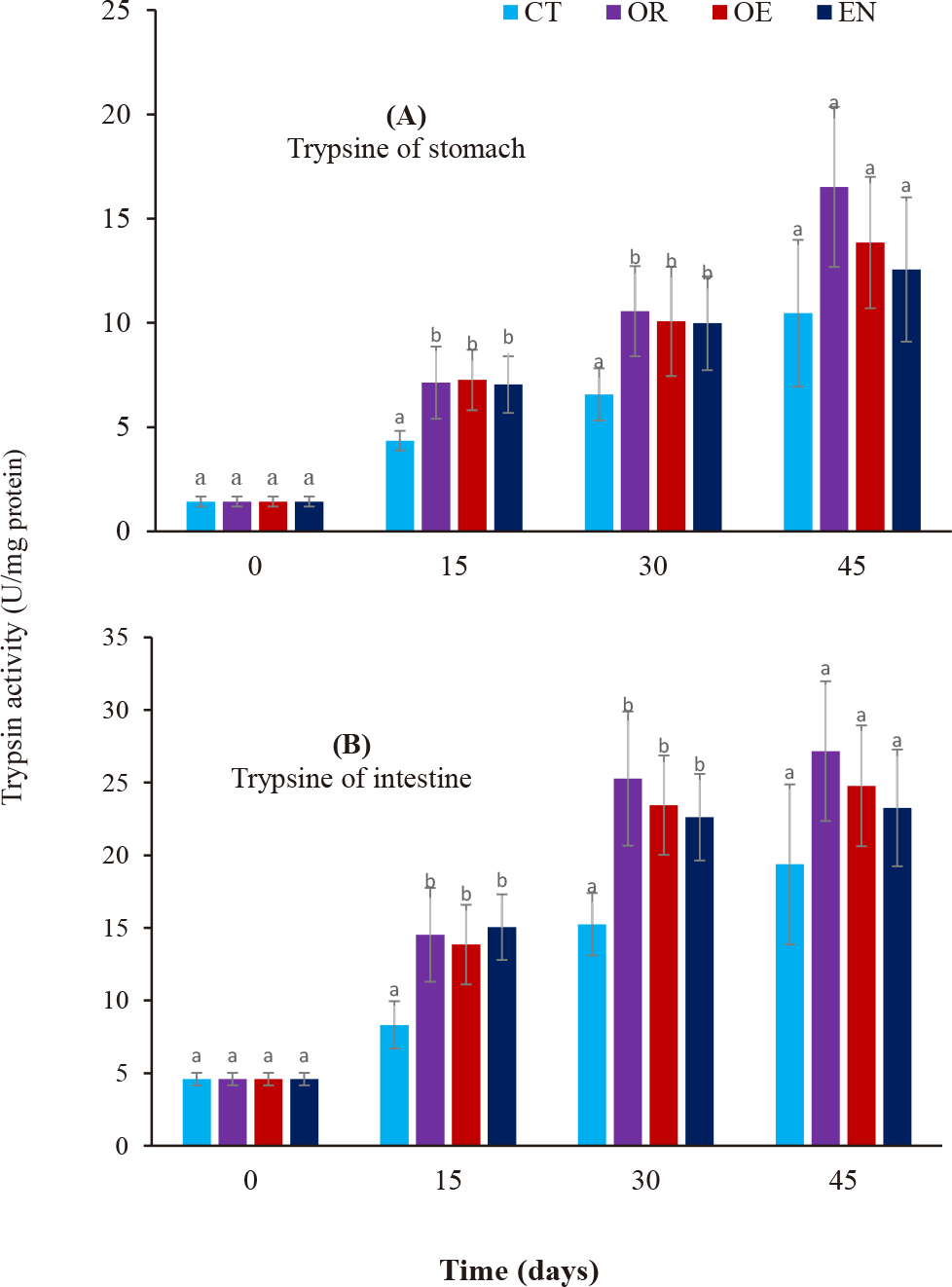
Discussion
The use of probiotics is highly promising in marine larviculture by direct addition to the rearing water environment (Jahangiri & Esteban, 2018) or through feeding Taoka et al. (2007). The application of probiotics in larviculture is becoming more important due to the problem of antibiotic use in aquaculture, resulting in antimicrobial-resistant bacteria (Vine et al., 2006). Moreover, there are many challenges in marine fish larviculture with high mortalities due to many unknown causes (Ottesen & Olafsen, 2000). Cordero et al. (2015) recommended that probiotic administration in aquaculture systems through egg incubation, nursery systems, rearing, and culture systems has improved water quality and immunization of aquatic animals. For instance, Ottesen & Olafsen (2000) have reported that probiotic L. plantarum has enhanced the survival rate of larvae-stage Atlantic halibut (Hippoglossus hippoglossus). By applying probiotics to live food, Mandiki et al. (2011) have demonstrated the roles of probiotics on survival, growth, lysozyme activity, and total immunoglobulins in perch larvae. Likewise, probiotics were also administrated in live food to improve the digestive enzyme of gilthead sea bream (Sparus aurata) larvae (Suzer et al., 2008). According to Lauzon et al. (2010), up to 70% of the microbial population in the intestine of cod larvae was from probiotic administration by adding to the rearing environment. Likewise, the administration of probiotics by adding to rearing water or through food has assisted gastrointestinal tract development, enhanced the immunity of Snook larvae, inhibited pathogens, and improved water quality parameters. This study demonstrates that commercial probiotics improved growth and the digestive enzyme activity of amylase and trypsin in the stomach and intestine of spotted scat fingerlings. The survival rate of fish reached 100% during the experiment proving that the larvae got used to the commercial pellet feed (after 20 days). These results are consistent with the publication of Taoka et al. (2007) on Tilapia fingerlings using commercial probiotics. Amylase activity of the stomach and intestine increased during the cultural period in all treatments and there was a significant difference between experimental treatments and the control treatment on days 15 and 30 of rearing. The variability of amylase activity in the digestive tract on fish larval development has been reported, with the appearance of maximum amylase activity during the early development of fish larvae (Yúfera et al., 2018). This proves that using commercial probiotics through feeding or adding them to the culture environment has the effectiveness of stimulating the activity of the above enzymes. Dung et al. (2017) have also found changes in the digestive enzyme activity in snakehead Channa striata from fry to 35 days old. The activity of digestive enzymes in the larval stage of catfish depends on the environmental temperature; the higher the temperature, the stronger the activity of digestive enzymes (Do et al., 2020; Magouz et al., 2020). The role of probiotics in increasing enzyme activity in yellowfin tuna larvae has been demonstrated to increase intestinal microorganisms, reduce disease incidence, and stimulate enhanced metabolism (Haryanti et al., 2021). Rønnestad et al. (2013) have reported that amylase activity patterns include a high activity level after first feeding and then tend to decrease during the following weeks or reach the maximum activity in the middle of the larval stage. In addition, Moyano et al. (2014) suggested that high amylase activity level tends to disappear in the transition to juvenile with either a second increase at the end of the larval stage or a continuous increase during larval development. Amylase activity in fish is also useful for measuring the ability to digest starch in feed materials in the digestive tract and is also useful for modeling the hydrolysis of feed (Gilannejad et al., 2018). Trypsin activity had a pattern similar to amylase activity in both stomach and intestine, increasing sharply during 15–30 days and then slightly increasing in the stomach and keeping stable in the intestine until day 45. Dung et al. (2017) documented that trypsin activity depended on types of food intake.
The HSI is the main index to evaluate metabolic activity in animals, as an indicator of the energy reserves in fish liver (Verma & Prakash, 2019). Meanwhile, the CF condition index has been an index to evaluate the health status of fish since the early 20th century. Table 2 shows that the CF index of spotted scat tends to increase from day 15 to day 30 and then decrease to day 45 in all treatments. These values are higher than that of Rohu fish (Taoka et al., 2007). However, the HSI coefficient in spotted scat in this study ranged from 2.28% to 3.23% equivalent to that published by Magouz et al. (2020) on Tilapia and lower than that of Rohu fish according to the publication of Taoka et al. (2007).
Conclusion
Supplementing probiotics into the food or rearing environment for spotted scat in the nursery stage has better growth rate and enzymatic activity as indicators to evaluate the health status of fish, but there are no differences in survival rates between probiotic treatments and control treatment. This study shows that supplementing probiotics through feeding was the best way to increase the growth weight of spotted scat. However, there is still no clear explanation of the mechanism of probiotic effects on the synthesis of digestive enzymes in fish. Further research on the mechanism of stimulating digestive enzyme synthesis when probiotics are used in commercial fish seed production is required.