Introduction
Short-chain fatty acids (SCFA) such as acetate and butyrate are actively used as functional feed additives due to their positive effects on digestibility (Defoirdt et al., 2007). SCFA are known to improve immune response and disease resistance in aquatic animals such as rohu (Labeo rohita) (Baruah et al., 2005), red hybrid tilapia (Oreochromis sp.) (Koh et al., 2016) and crayfish (Procambarus clarkia) (Xiao et al., 2021). Also, Pacific white shrimp (Penaeus vannamei) fed SCFA-supplemented diet was reported to show a high disease resistance against Vibrio spp. (Duan et al., 2018; Pourmozaffar et al., 2017). SCFA are absorbed through the intestinal epithelium via passive diffusion and utilized in various metabolic pathways, notably contributing to energy production, such as adenosine triphosphate generation in the citric acid cycle (Lim et al., 2010). Thus, an efficient delivery of SCFA to the intestine is thought to be important. Unlike terrestrial animals, aquaculture feed is easily dissolved in the culture water, which makes it difficult for SCFA to effectively deliver to intestine (Defoirdt et al., 2009).
Poly-β-hydroxybutyrate (PHB) is a polymer that is synthesized by a wide range of microorganisms under conditions of physiological stress, such as nutrient limitation and carbon excess (Duan et al., 2017). PHB is a biodegradable plastic that was developed to reduce the environmental impact of the extensive accumulation of non-degradable plastic waste and is gaining interest in a variety of industrial sectors, including the packaging and medical industries (Shah et al., 2008). Recently, numerous studies have been dedicated to both effectively degrading PHB and exploring its effect as a feed additive on aquaculture. PHB has been studied as a potential feed additive in the aquafeed because of its beneficial functions on the growth, immunity and disease resistance of Nile tilapia (Oreochromis niloticus) (Situmorang et al., 2016), Pacific white shrimp (Kiran et al., 2020) and gibel carp (Carassius auratus gibelio) (Qiao et al., 2020). The growth-promoting effect of PHB as an Artemia enrichment was reported in giant river prawn (Macrobrachium rosenbergii) (Nhan et al., 2010), Siberian sturgeon (Acipenser baerii) (Najdegerami et al., 2012) and Chinese mitten crab (Eriocheir sinensis) (Sui et al., 2014). Gao et al. (2019) showed that the feeding of PHB-enriched Artemia to shrimp could improve their growth, survival, resistance against to V. anguillarum and tolerance to salinity shock. PHB can be biologically degraded to β-hydroxybutyric acid, a type of SCFA, by specific degradative enzymes in the intestines of aquatic animals and Freier et al. (2002) observed efficient degradation of PHB by digestive enzymes. The β-hydroxybutyric acid produced by the breakdown of PHB has been reported to play a beneficial role in the growth and immunity of aquatic animals (Duan et al., 2017; Situmorang et al., 2016). However, the information on the effects of PHB on gene expressions related to the growth, digestive enzymes and immunity is very limited in the shrimp at post-larvae stage.
Pacific white shrimp are one of the most important species in global shrimp aquaculture, due to their rapid growth and high resistance against environmental stressors even in the high-density cultures (Bardera et al., 2019). Successful production of high-quality larvae is one of the most important factors in the shrimp aquaculture. However, shrimp aquaculture has faced many challenges, such as critical diseases and water quality issues frome the high-density culture system leading to the increased mortality and the eventual low production (Walker & Mohan, 2009). In order to address these challenges and continue to improve the shrimp aquaculture industry, the development of effective feed additives is essential. Thus, this study was conducted to investigate the effects of feeding PHB-enriched Artemia on the growth performance and gene expressions related to the growth, digestive enzymes and innate immunity in Pacific white shrimp in post-larvae stage.
Materials and Methods
PHB used for nutritional enrichment of Artemia was provided by CJ Cheiljedang (Seoul, Korea). Three Artemia groups consisted as non-enriched group (Con) and an enriched groups with 0.25% and 0.50% of PHB dissolved water (designated as PHB25 and PHB50, respectively). Artemia cysts (Sep-art Artemia, INVE, Salt Lake City, UT, USA) were hatched using an Artemia incubator (ZH-2000, Ziss Aqua, Osan, Korea). The hatching conditions were same as follows, density: 0.75 g/L, water temperature: 30.0 ± 0.5°C and salinity: 30.0 ± 0.5 psu. Artemia cysts were incubated for 24 h and decapsulated using a magnetic rod. After 2 h of Artemia hatching, 500 ml of PHB dissolved water was supplemented into Artemia tanks according to each concentration of larvae. Artemia was separated using a 0.18 mm sieve (Ziss EZ sieve SF-1, Ziss Aqua) and washed with fresh water to remove salinity and attached materials. The approximate composition of PHB-enriched Artemia were determined by standard procedures (AOAC, 2005) and the results were provided in Table 1. After enrichment, Artemia were observed under an optical microscope (DM750, Leica Microsystems, Heerbrugg, Switzerland) equipped with a digital camera (ICC 50 E, Leica Microsystems) to confirm enrichment (Fig. 1).
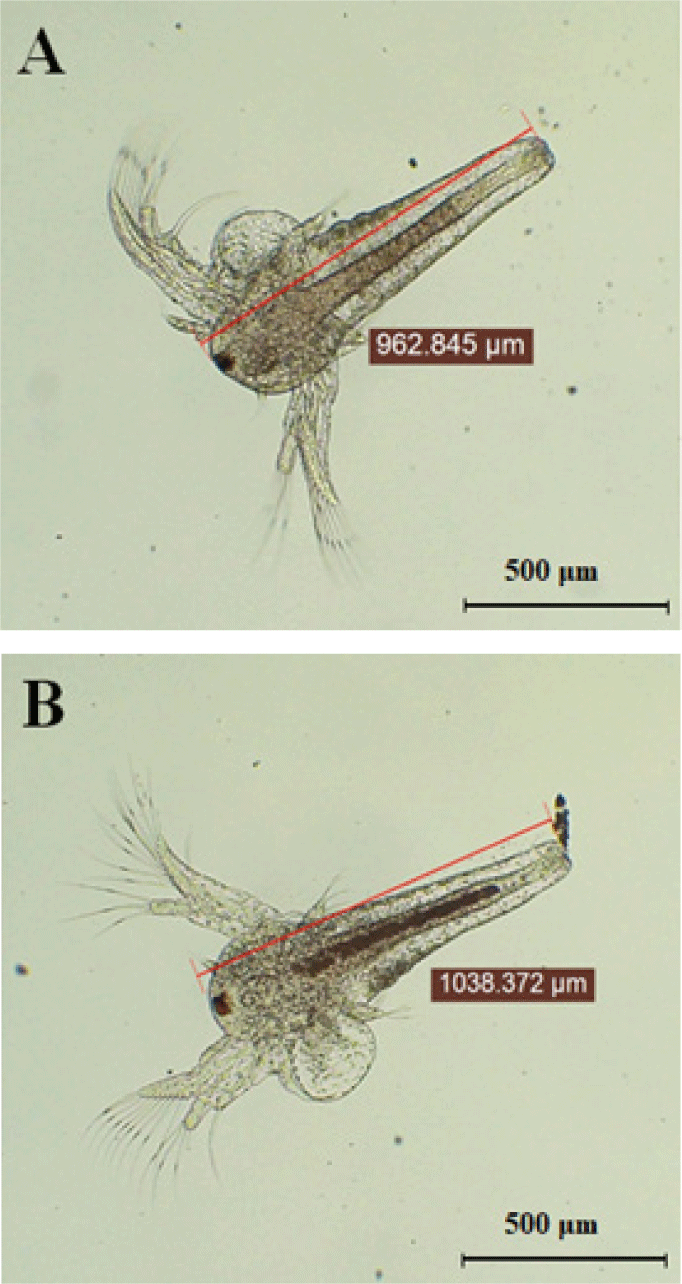
Pacific white shrimp post-larvae were obtained from Daedong Susan (Muan-gun, Korea). After acclimation to the experimental condition, 900 shrimp were randomly placed as triplicate groups in 9 acrylic tanks (96 L). The initial average body weight of the shrimp was 2.50 ± 0.01 mg and the initial average body length was 0.90 ± 0.01 cm. Dissolved oxygen (DO) in tank was maintained using aeration. The photoperiod was maintained at 12 light: 12 dark using a fluorescent lamp. The feeding trial was conducted for 14 days with experimental feed three times a day (07:00, 13:00 and 19:00 h). The daily feed amount was supplied referring to the method described by Alday-Sanz (2010), starting with 300 Artemia and increasing by 10% per day. Water temperature, DO (Pro20 Dissolved Oxygen Meter, YSI, Yellow Springs, OH, USA), salinity (Master-S28M Salinity Refractometer, ATAGO, Tokyo, Japan), pH (Seven Compact pH meter S210, Mettler Toledo, Columbus, OH, USA) and ammonia concentration (Strickland & Parsons, 1972) were measured daily during the experiment. During the feeding trial, the rearing conditions were followed: DO (6.59 ± 0.18 mg/L), salinity (32.1 ± 0.6 psu), pH (7.95 ± 0.10), water temperature (30.5 ± 0.5°C) and ammonia (0.02 ± 0.01 ppm).
After the feeding trial, weight and length measurements were taken for all shrimp in each tank. Based on the measurement results, final body weight (FBW), weight gain (WG), length gain (LG), specific growth rate (SGR) and survival were calculated. Then, 10 shrimps per tank (30 shrimps per experiment group) were randomly selected and anesthetized in ice water. The hepatopancreas was frozen using liquid nitrogen and then stored in a deep-freezer (–80°C). The hepatopancreas was analyzed for relative gene expression.
Hepatopancreatic RNA was extracted using TRI-zol® (93289, Sigma-Aldrich, St. Louis, MO, USA). The quantity and quality of total RNA were measured using a Nano drop 2000TM (Thermo Scientific, Wilmington, DE, USA). The 260/280 nm ratios of all samples ranged from 1.8 to 2.0; therefore, synthesis of cDNA was performed using the PrimeScriptTM RT reagent kit (TaKaRa Bio, Shiga, Japan). The synthesized cDNA was diluted 50-fold with nuclease-free water. The primer sequences of trypsin, chymotrypsin, amylase, insulin-like growth factor-I (IGF-I), insulin-like growth factor-binding protein (IGF-BP), prophenoloxidase (proPO) and penaeidin-3a (Pen-3a) used in the experiments are listed in Table 2. qPCR was performed using Real-Time System TP 950 Thermal Cycler DiceTM (TP950, TaKaRa Bio) and 2 μL of cDNA, 10 μL TaKaRa Ex TaqTMSYBR premix, 0.4 μL of forward & reverse primer and 7.2 μL of distilled water, with the total volume adjusted 20 μL for analysis. The analysis was carried out by adjusting the volume to 20 μL. qPCR was performed following the method of Lee et al. (2021). The relative levels of mRNA expression were calculated according to Pfaffl (2001) (2–ΔΔCT threshold cycles [CT]).
The statistical analysis utilized one-way analysis of variance (ANOVA) and the results were presented as mean ± SD. Data of each parameter were initially checked for normality. The statistical analysis was conducted using SPSS 24.0 (IBM, NY, USA). The mean differences between groups were compared using Duncan’s multiple range test (p < 0.05). Prior to analysis, the percentage data were transformed to an arcsine value.
Results
FBW, WG, LG and SGR were significantly higher in PHB25 and PHB50 groups than those in Con group (Table 3). Survival was not significantly different among all the groups. Relative expressions of trypsin and amylase were significantly upregulated in PHB25 group than in Con group (Fig. 2). Relative expression of chymotrypsin was significantly upregulated in both PHB25 and PHB50 groups compared to Con group. Relative gene expression of IGF-I was significantly upregulated in PHB25 group than in Con group (Fig. 3). Relative gene expression of IGF-BP was significantly upregulated in both PHB25 and PHB50 groups than in Con group. The gene expression of proPO was significantly upregulated in PHB25 and PHB50 groups as compared to Con group (Fig. 4). Pen-3a gene expression was significantly upregulated in PHB50 group as compared to Con group.
The Artemia nauplii was enriched with different levels of PHB by 0, 0.25 and 0.5% (designated as Con, PHB25 and PHB50, respectively).
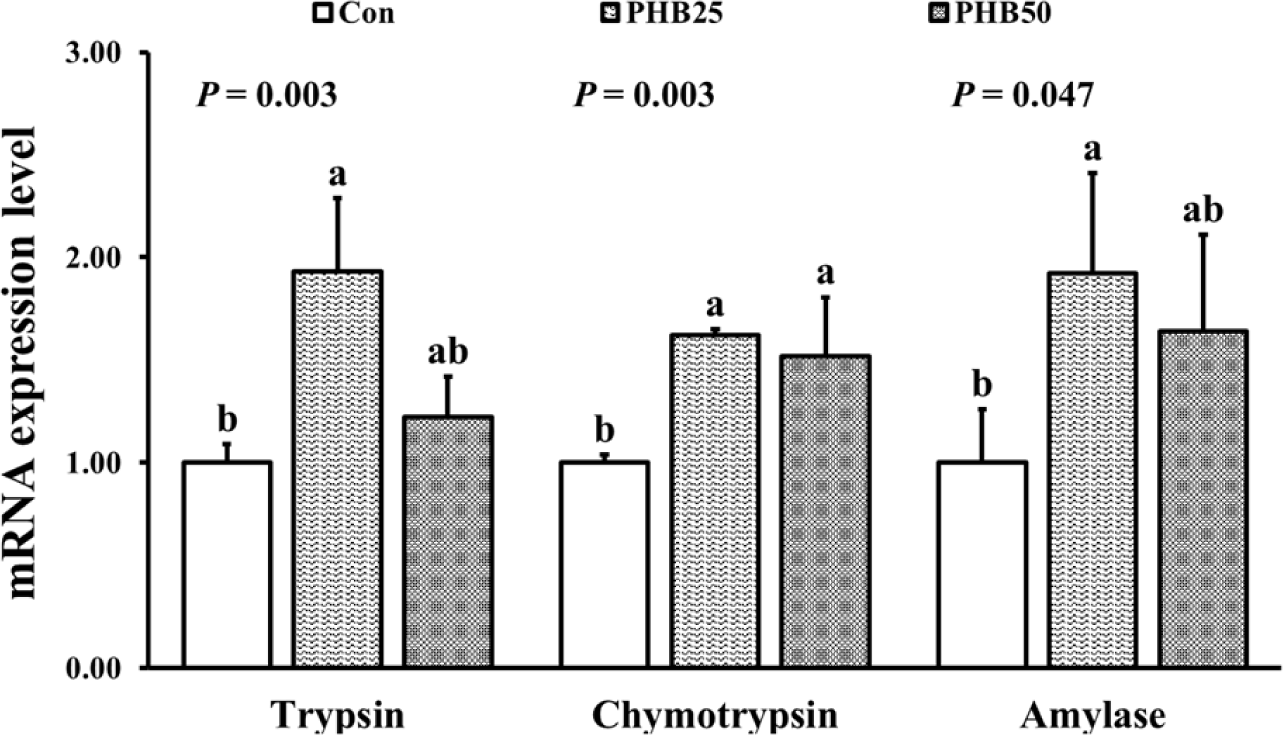
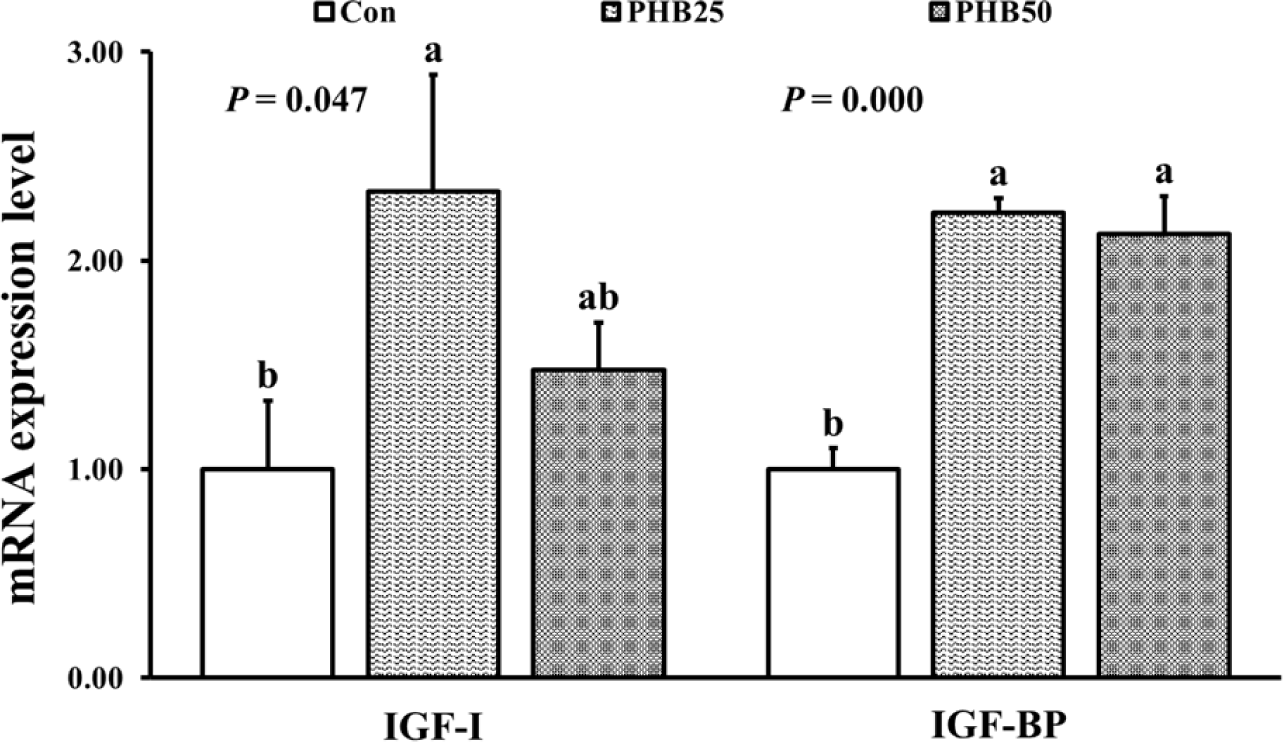
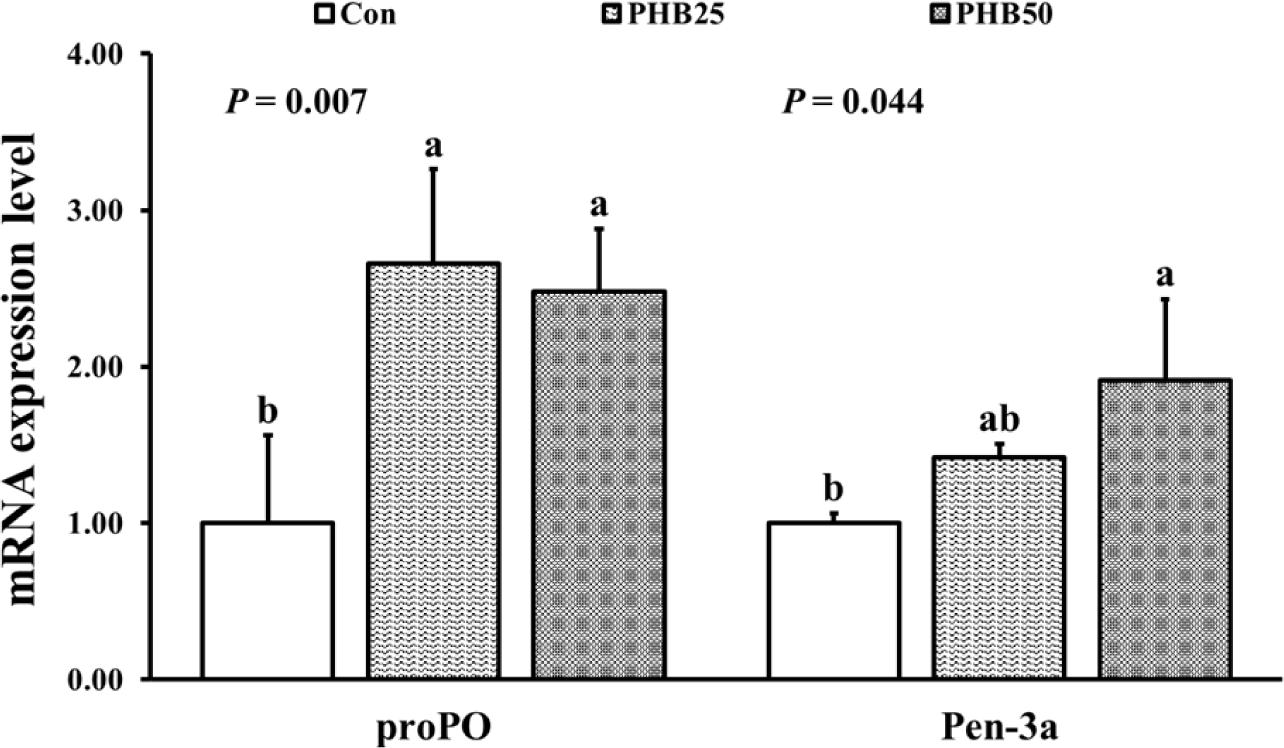
Discussion
PHB is known to be decomposed into β-hydroxybutyrate, one of the SCFA, by intestinal microorganisms such as Acidovorax, Acinetobacter and Ochrobactrum genera (Duan et al., 2017; Liu et al., 2010). Butyrate, serving as the primary energy source for colonic epithelial cells, plays a pivotal role in influencing cell proliferation and modulating mucosal blood flow through the release of growth factors or gastrointestinal peptides like gastrin (Blottière et al., 2003; Lee et al., 2021). Additionally, butyrate provides energy to intestinal epithelial cells, increasing intestinal health and resistance to infection and increases nutrient digestibility by increasing digestive enzyme activity (Defoirdt et al., 2009). Dietary PHB supplementation was reported to improve the growth of Siberian sturgeon (Najdegerami et al., 2012), Chinese mitten crab (Sui et al., 2016) and Pacific white shrimp (Gao et al., 2019) by inhibiting proliferation of pathogens and/or promoting the proliferation of some beneficial bacteria such as Bacillus and Lactobacillus. PHB addition at levels of 5–10 g/kg to sea cucumber (Apostichopus japonicus) feed led to an increase in the abundance of Rhodobacteraceae, known to positively contributed on growth and health, alongside observed increases in amino acid, lipid and nucleotide metabolism, as predicted by functional analysis of the gut microbiota (Liu et al., 2022). Duan et al. (2017) found that the addition of PHB to the diet (30 g/kg) significantly raised butyrate levels in the gut, potentially as a source for energy production and lipid synthesis. Silva et al. (2016) reported that the dietary supplementation of butyrate and PHB at 20 g/kg diet was able to improve the growth, survival, intestinal, length and width of villi and digestive enzymes activity of Pacific white shrimp. Several studies have demonstrated that nutritional enrichment of PHB can be effectively delivered through Artemia as prey for fish or crustacean larvae. In fishes, the feeding of PHB enriched Artemia was reportedly demonstrated to increase the growth performance of European bass (Dicentrarchus labrax) (de Schryver et al., 2010) and Siberian sturgeon (Najdegerami et al., 2015). In crustaceans, the feeding Artemia enriched with PHB improved the growth and survival of giant river prawns (Nhan et al., 2010), Chinese mitten crab (Sui et al., 2014) and Pacific white shrimp (Gao et al., 2019). Notably, similar to our study, Nhan et al. (2010) reported that 0.5% nutrient enrichment with PHB in Artemia culture water increased the growth and survival of giant river prawns. Sui et al. (2014) reported that 100 mg/L of PHB in Artemia culture water can be effectively transferred to Chinese mitten crab, which is sufficiently concentrated in Artemia, and can enhance growth by beneficially affecting the bioavailability of minerals and trace elements required for growth in the gastrointestinal tract. Defoirdt et al. (2007) found that β-hydroxybutyrate degraded from PHB provides energy to produce a stronger intestinal epithelium, thereby protecting shrimp from infection by pathogens such as Vibrio spp. Based on precedent studies and our research findings, it is presumed that PHB effectively delivered into the intestines of shrimp via Artemia not only activates metabolism through energy provision, enhancing digestive enzyme activity and growth factor expression but also enhances beneficial bacteria countering pathogens, thus likely exerting a positive influence on the gut microbial ecosystem. Improvements in the gut microbial community are known to have positive effects on physiological functions like nutrient digestion, including amino acids, vitamins, mineral and lipids, and protection from pathogens (Merrifield & Ringø, 2014). Therefore, we maintain that PHB can be used as a nutrient enrichment for live feed to effectively promote their growth into Pacific white shrimp post-larvae.
Nutrient availability is directly related to the growth of animals and the activity of digestive enzymes is important for the nutrient digestion and absorption. Trypsin, chymotrypsin and amylase are the most abundant protein and carbohydrate enzymes in the hepatopancreas of shrimp and they are developed in the post-larvae stage (Roy et al., 2018). Silva et al. (2016) reported that the supplementation of PHB (20 g/kg) in Pacific white shrimp diet significantly improved the activity of trypsin, chymotrypsin and amylase. Duan et al. (2017) found that the activities of pepsin, trypsin, amylase and lipase were significantly improved by supplementing the diet of Pacific white shrimp with PHB (30 g/kg). Shrimp that consumed feed containing 10 g/kg PHB were found to have improved digestion and absorption through increased digestive enzyme activity and minimized waste production, resulting in WG (Kiran et al., 2020). The heightened activity of digestive enzymes when utilizing PHB as a feed additive or nutritional enrichment might be due to a decrease in the intestinal pH of shrimp. The breakdown of PHB polymers into β-hydroxybutyric acid monomer in the intestines was believed to impact the intestinal pH which was gradually decreased with increasing PHB levels (de Schryver et al., 2010). This pH reduction might enhance the activity of digestive enzymes, potentially improving the ability to digest and absorb nutrients from the feeds (Baruah et al., 2005). From our findings, we conclude that the upregulated gene expression of trypsin, chymotrypsin and amylase within the hepatopancreas of shrimp post-larvae fed PHB-enriched Artemia significantly impacts nutrient digestion and absorption, thereby contributing to the observed accelerated growth.
The insulin signaling pathway, essential for regulating metabolism, growth and development, involves IGF-BP and its receptor, which play pivotal roles in the biological functions of IGF, insulin and insulin-like peptides (Pang et al., 2021). Gutiérrez et al. (2007) reported that IGF-I levels in the hepatopancreas and muscle of Pacific white shrimp are involved in carbohydrate metabolism and are involved in cell metabolism and growth. IGF-BP regulates IGF activity and induces inhibition of apoptosis and enhances cell growth (Zhang et al., 2017). Shrimp fed a diet supplemented with 2 g/kg monobutyrin exhibited upregulated IGF-BP expression (Lee et al., 2021). In the present study, similar phenomenon was observed in shrimp fed PHB25 and PHB50 diets showing higher IGF-I and IGF-BP expressions than shrimp fed non-PHB diet. Thus, Artemia could be a good couriers of PHB in the promotion of IGF-I and IGF-BP gene expressions, which may help the shrimp growth.
The proPO activates phenoloxidase (PO), which plays important roles in the biosynthesis of melanin and inhibition of pathogenic toxin production (Sangsuriya et al., 2016). Duan et al. (2018) reported that the succinic acid supplementation at 5 g/kg diet in the Pacific white shrimp diet significantly enhanced proPO gene expression. Similar to the findings in our study, previous research reported that dietary supplementations for Pacific white shrimp with 3 g/kg of citric acid (Su et al., 2014) and 2 g/kg of tributyrin (Lee et al., 2021) were able to enhance the expressions of proPO and PO activities, effectively inhibiting Vibrio bacteria. Penaeidin is an important antimicrobial peptide in shrimp that exhibits potent antimicrobial activity against pathogenic microorganisms, including bacteria, fungi and viruses (Song & Li, 2014). He et al. (2017) reported that the supplementation of 0.9 g/kg of organic acid mixture (citric acid, 25%; sorbic acid, 16.7%, w/w) in Pacific white shrimp diet significantly improved their penaeidin expression and disease resistance against V. parahaemolyticus. Also, it was reported that the supplementation of propionic acid at 5 g/kg diet for Pacific white shrimp increased the activity of Pen-3a (Pourmozaffar et al., 2017). Therefore, our results clearly suggest that PHB could be used as an effective immunostimulants in shrimp diet.
In conclusion, the findings from this study support that the growth of shrimp can be improved by feeding PHB-enriched Artemia. The gene expressions of trypsin, chymotrypsin, amylase, IGF-I and IGF-BP in the shrimp were upregulated by feeding the PHB-enriched Artemia, suggesting that Artemia serves as an effective vehicle for PHB delivery to the shrimp. Additionally, PHB appears to affect proPO and Pen-3a gene expressions indicating its potential as an immunostimulant for the shrimp. This study demonstrated that PHB acts as a functional nutritional enrichment for post-larvae Pacific white shrimp growth, digestibility and immunity. However, further research is needed on the optimal PHB concentration for nutritional enhancement to improve larval production efficiency.