Introduction
The giant gourami (Osphronemus goramy; Lacepède, 1801) is one of the main freshwater fish of economic importance in Indonesia (Arifin et al., 2019). The fish is a native fish of Indonesia that has high economic value and has become a main fishery commodity in Indonesia. Gourami has a compact and delicious meat, so market demand is stable at a high price. In addition, gourami can be cultivated in environments with low dissolved oxygen content with relatively low production costs because they are herbivores with low protein feed requirements. Several gourami production centers have developed in several regions of Indonesia, both in Java and Sumatra. National gourami production has increased by 35.09% per year from 2015 to 2018 (Ministry of Marine Affairs and Fisheries [KKP], 2018). So, gourami farming has high prospects for development.
Intensive gourami farming is faced with the problem of infection with a number of diseases that have an impact on fish deaths. The main disease in gourami farming is Motile Aeromonad Septicemia (MAS) caused by Aeromonas hydrophila bacteria (Novita et al., 2021). As reported by Purwaningsih et al. (2014), MAS in addition to causing death, also has an impact on decreasing the quality of fish produced. The mass death of 47 tons of gourami occurred in West Sumatra in 2006 (Department of Marine Affairs and Fisheries, Padang Pariaman Regency, 2015). MAS infection also causes mass mortality of carp of up to 87%–100% in the Purwokerto area, Central Java (Khumaidi & Hidayat, 2018).
Therefore, a number of efforts were made to overcome the problem of disease in gourami, both through a vaccination approach (Purwaningsih et al., 2014), feed (Farisi et al., 2020), immunostimulants (Wahjuningrum et al., 2010), and probiotics (Novian et al., 2023). Disease control in gourami through genetic approaches has not been reported, although such approaches have been widely applied to a number of important fish commodities such as tilapia (Zhou et al., 2013), and goldfish (Hayuningtyas et al., 2013). The success of selection in a number of commodities opens the hope of obtaining superior gourami that has resistance to disease. One method of selection to obtain disease-resistant fish is the molecular marker approach.
Molecular markers encoding disease-resistant characters have been studied in a number of aquaculture species. Allele-specific major histocompatibility complex (MHC) II correlates with disease resistance in tilapia (Zhou et al., 2013), goldfish (Hayuningtyas et al., 2013), rainbow trout (Dijkstra et al., 2013), Chinese long snout catfish (Shen et al., 2011), turbot Scophthalmus maximus (Du et al., 2012), Catfish (Alimuddin et al., 2018), and three-spined fish sticklebacks (Gasterosteus aculeatus) (Consuegra & de Leaniz, 2008; Kurtz et al., 2006; Wegner et al., 2006). It is also reported that there is a close relationship between MHC gene variation and resistance traits, such as in Atlantic salmon Salmo salar (Landry & Bernatchez, 2002), sockeye salmon, Oncorhynchus nelka (Miller et al., 2001). Thus, further research on MHC II in gourami is needed to strengthen the data and information regarding the resistance of Jambi and Kalimantan gourami strains to MAS disease.
Materials and Methods
This experiment was conducted at the gourami hatchery, the microbiology laboratory, and the physiology and genetics laboratory of the Research Institute for Fish Breeding, Subang, Indonesia. For this investigation, seeds of Jambi gourami (GJ) and Kalimantan gourami (GK) strains (body weight: 13–18 g) were used. The fish seeds were obtained from a seed production process at the institute. Acclimatization was carried out on test fish in glass tanks (100 × 50 × 40 cm) containing 40 L of freshwater. Fish were manually fed fish meals (26 percent crude protein) at 08:00 and 16:00 hours every day until they were satisfied. Water was aerated to maintain an oxygen level of 5 mg/L, and the water temperature was kept at 28°C–30°C with a thermostat heater.
The bacteria used in this study was an isolated A. hydrophila from the ulcerated lesion of a disease-infected gourami. Isolation, characterization, Koch postulate test, and the growth curve of the bacteria refer to Wahjuningrum et al. (2010). The bacteria stock in Rimler Shotts (RS) media was cultured in Trypticase Soy Broth (TSB) media, and incubated for 24 hours at 29°C. The determined lethal dose of 50% (LD50) of the bacteria in the GJ and GK strains refers to Febrianti et al. (2021).
The recent experiment consisted of three phases, the challenge test of gourami seeds to A. hydrophila infection, the detection of MHC II as a molecular marker, and the evaluation of the growth performance of the gourami seed.
The gourami seeds (GJ and GK) from nursery ponds were acclimated to laboratory conditions for 3 days. For the challenge test, 0.1 mL of 1 × 105 CFU/mL of A. hydrophila suspension was injected intramuscularly from each treatment group (in seven replicates), i.e., GJ and GK. As negative controls, each GJ and GK group was injected with 0.1 mL of sterile PBS. After injection, 10 fish were transferred to a glass container previously filled with 50 L of sterile water. The water quality in the containers was maintained at 28°C–32°C in temperature, 8.1–8.4 in pH, and 6.7–7.5 mg/L in dissolved oxygen concentration. Observation of clinical symptoms and fish mortality was performed every day for a period of 14 days.
The fish were fed two times a day at 08:00 and 16:00 using commercial fish feed (31%–33% crude protein, Cargil, Indonesia) at a daily level of 3% of the biomass. Fecal matter and uneaten feed were removed daily, and 10% of the water volume was replaced. The observed parameters consist of survival rate, clinical signs, histopathology, and blood performance tests. The water quality during the challenge test was monitored at the initial and end of the study. To confirm the cause of fish mortality, bacteria were isolated from the fish’s liver, kidneys, and lymph using RS media. The isolated bacteria from the dead fish were identified by KIT API 20 NE (Biomerieux, Rue des Aqueducs, France) and standard PCR using A. hydrophila-specific 16S ribosomal RNA gene primers.
The hematological analysis consists of erythrocyte total, leucocyte total, hemoglobin level, and hematocrit level. Three fish samples from each treatment were taken to analyze the blood profile. The erythrocyte and leucocyte analysis refers to Blaxhall & Daisley (1973), and the hemoglobin level analysis refers to (Wedemeyer & Yasutake, 1977). Blood was drawn into a capillary hematocrit tube with one end sealed with cha-seal tube sealing compound (Medline, Northfield, IL, USA), and centrifuged for 5 min using a micro hematocrit centrifuge (Biochemical Laboratories, Edgewood, NM, USA). Hematocrit values were obtained using the micro hematocrit tube reader (01502-00, Hawksley & Sons, Lancing, UK).
Blood smears were prepared by placing a small drop of blood near one end of a clean glass slide and spreading it evenly with a spreader slide oriented at an angle of about 30°. The smears were air-dried, fixed in a methanol-based fixative solution, and stained using the Diff-Quik Staining Technique. Each blood smear was dipped in a solution containing buffered eosin Y (Solution I) followed by dipping in buffered methylene blue and azure A dye (Solution II). After staining, the smears were rinsed in distilled water to remove excess dye and allowed to air dry. The blood cells were examined and counted under the microscope (40×) using a differential counter and expressed as a percentage of the total cells counted. The concentration of blood hemoglobin is determined using the Sahli method, which converts blood hemoglobin into the form of hematin acid. The Sahli tube is filled with 0.1 N HCl to the lowest term limit. The blood sample is sucked using a hemoglobin pipette up to the 20 mm mark, and inserted into the Sahli tube. The change that appears is the formation of hematin acid, which is brown or dark brown. The aqueous is dripped while shaking carefully until the color is the same as the standard color. The hemoglobin level is read by looking at the lower meniscus of the fluid in the Sahli tube.
The detection of the presence of the MHC II gene in gourami was conducted using a PCR assay. Genomic DNA was extracted from the fins of living and dead fish after the challenge test. Genomic DNA extraction is performed using the GeneJet Genomic DNA Purification Kit (K0721, Thermo Scientific, Waltham, MA, USA) according to the procedure in the kit manual.
The extracted genome DNA was then amplified using the Thermal Cycler tool (Bio-Rad, Hercules, CA, USA). Amplification of DNA samples on the Thermal Cycler machine is conducted with the MyTaq HS Red Mix (PCR) Master Mix (2×) kit (BIO-25048, Bioline, Cincinnati, OH, USA). The exon fragments of two genes were amplified using primers, namely forward: 5’-ATG GAA GAT GAA ATC GCC GC-3’ and reverse: 5’-TGC CAG ATC TTC TCC ATG TCG-3’ (Wegner et al. 2006). PCR reactions are conducted with a total volume of 25 μL. The mixture for the PCR reaction includes ultrapure water (ddH2O) of 18.5 μL; 10× PCR buffer of 2.5 μL; the volume of each primer was 0.2 mM, 0.2 mM for dNTP, 1 U Taq polymethine, and 0.1 μL DNA template. The DNA amplification program was determined with an initial pre-denaturation phase of 95°C for two minutes, followed by 35 cycles as follows. Denaturation at 95°C for 30 seconds, annealing at 41°C for 45 seconds, and chain elongation at 72°C for one minute were followed by a final elongation process at 72°C for 5 minutes. Electrophoresis was performed using 1.5% agarose gel (w/v), at a voltage of one hundred volts for 35 minutes. DNA was visualized using Peqgreen and further visualized using a Gel Doc UV transilluminator (Vilber Loumat, Marne La Vallée, France).
Water quality observations aim to ensure the fish’s mortality was not caused by poor water quality. Water quality parameters including temperature, pH, and dissolved oxygen (DO) concentration were measured using a water quality checker (WQC-22A, TOA-DKK, TOKYO, JAPAN), whereas alkalinity, total ammonium nitrogen (TAN), and nitrite were measured using the standard procedures described in American Public Health Association (APHA, 1998).
The data were presented as the average standard error of replicate measurements. All survival data were arcsine transformed. The normality and homogeneity of the variance were determined using the Sapiro-Wilk and Levene tests, respectively. Statistical analyses were carried out using the statistical software SPSS 25 (IBM, Armonk, NY, USA). The differences between treatments were determined using the independent F-test, followed by the post hoc Duncan test, all at a significance level of 0.05.
Results
The mortality rate of Kalimantan gourami (GK) in the challenge test with A. hydrophila was highest (67.14%) and significantly different (p < 0.05) than other treatments (Table 1). The GJ and GK infected with the bacteria experienced mortality at 72 hours post-infection (hpi). Mortality increased at the 96 hpi infection in both GJ and GK. On the eighth day post-infection (dpi), GJ fish were no longer experiencing mortality, while mortality in GK fish still occurred. From the ninth dpi until the end of the experiment, none of the test fish populations experienced mortality anymore (Fig. 1).
Treatment | Initial number (fish) | Dead number (fish) | Mortality (%) |
---|---|---|---|
KJ | 70 | 0 | 0.00 ± 0.00a |
KK | 70 | 0 | 0.00 ± 0.00a |
GJ | 70 | 37 | 52.86 ± 9.51b |
GK | 70 | 47 | 67.14 ± 7.56c |
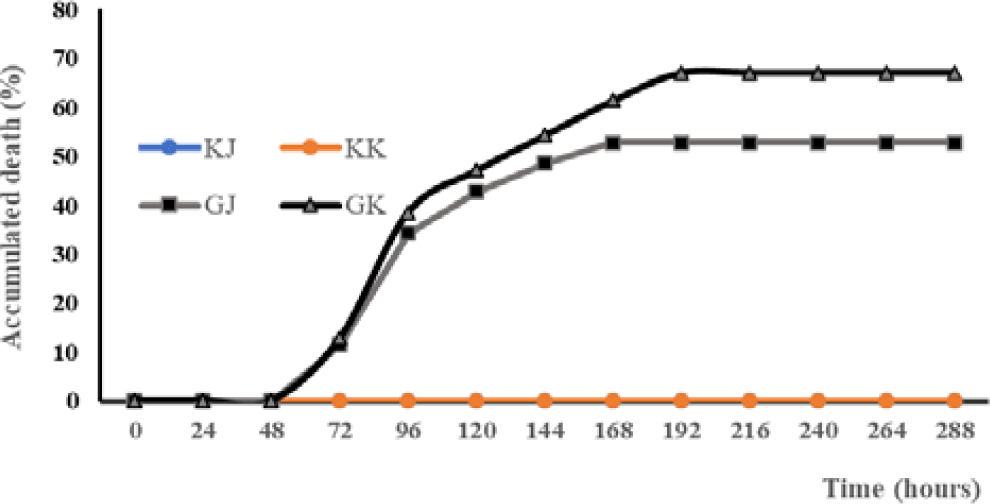
Fish showed different clinical symptoms between treatments. In the treatment of KJ and KK, no clinical symptoms appeared. In contrast, clinical symptoms appear in the treatment of GJ and GK (Table 2). There is a difference in the onset of clinical symptoms between GJ and GK fish. Based on the Mann-Whitney U-test there are significant differences in the parameters of loss of appetite (LA), disturbed body balance, weak (W), hemorrhagic (H), necrosis (N), loss of balance (LB), and death (D). The clinical symptoms of GK fish look more severe than those of GJ fish.
The clinical symptoms in the form of ulcers on the test fish’s body are starting to be observed in infected tilapia by the pathogenic bacteria, both in GJ and GK fish. On the third day of observation, a number of dead fish were observed, with their bodies undergoing necrosis or ulceration. On the seventh day of testing, besides the observation of some fish that did not show any wounds, there were also some other fish that showed wound healing, both in the GJ and GK populations.
Observation of blood profiles of erythrocytes, leukocytes, hemoglobin, and hematocrit levels was carried out in this study because blood parameters are indicators of fish health status (Esmaeili, 2021). In this study, the total number of erythrocytes in the GJ and GK treatments tended to decrease from day one to day four and increase from day six to day fourteen. Statistically, the total number of erythrocytes in KK and KJ is not significantly different. Significant differences are observed between infected and uninfected gourami fish. Differences are also noticeable between GJ and GK. During the study, the erythrocyte number ranged from 1.30–2.58 × 106 cells/mm3 (Fig. 2).
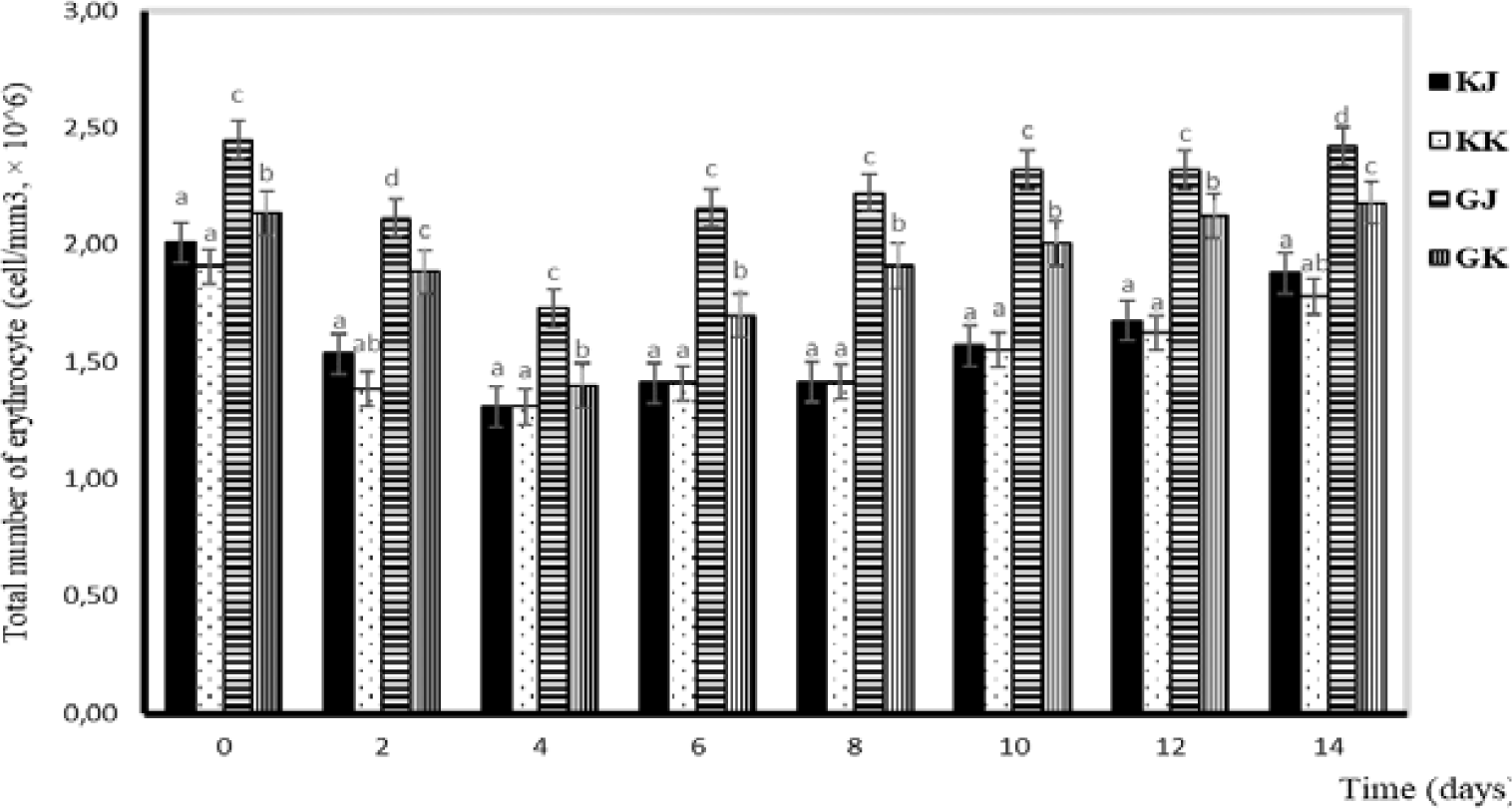
Leucocytes are one of the components of blood cells that function as non-specific defenses that will localize and eliminate pathogens (Anderson & Siwicki, 1993). Total leucocytes in this study tended to increase from day one to day four and decrease from day four in GJ and GK fish. Statistically, the total number of leucocytes in KK and KJ is not significantly different. Significant differences are observed between infected and uninfected gourami fish and between GJ and GK. The total number of leucocytes during the challenge test is shown in Fig. 3.
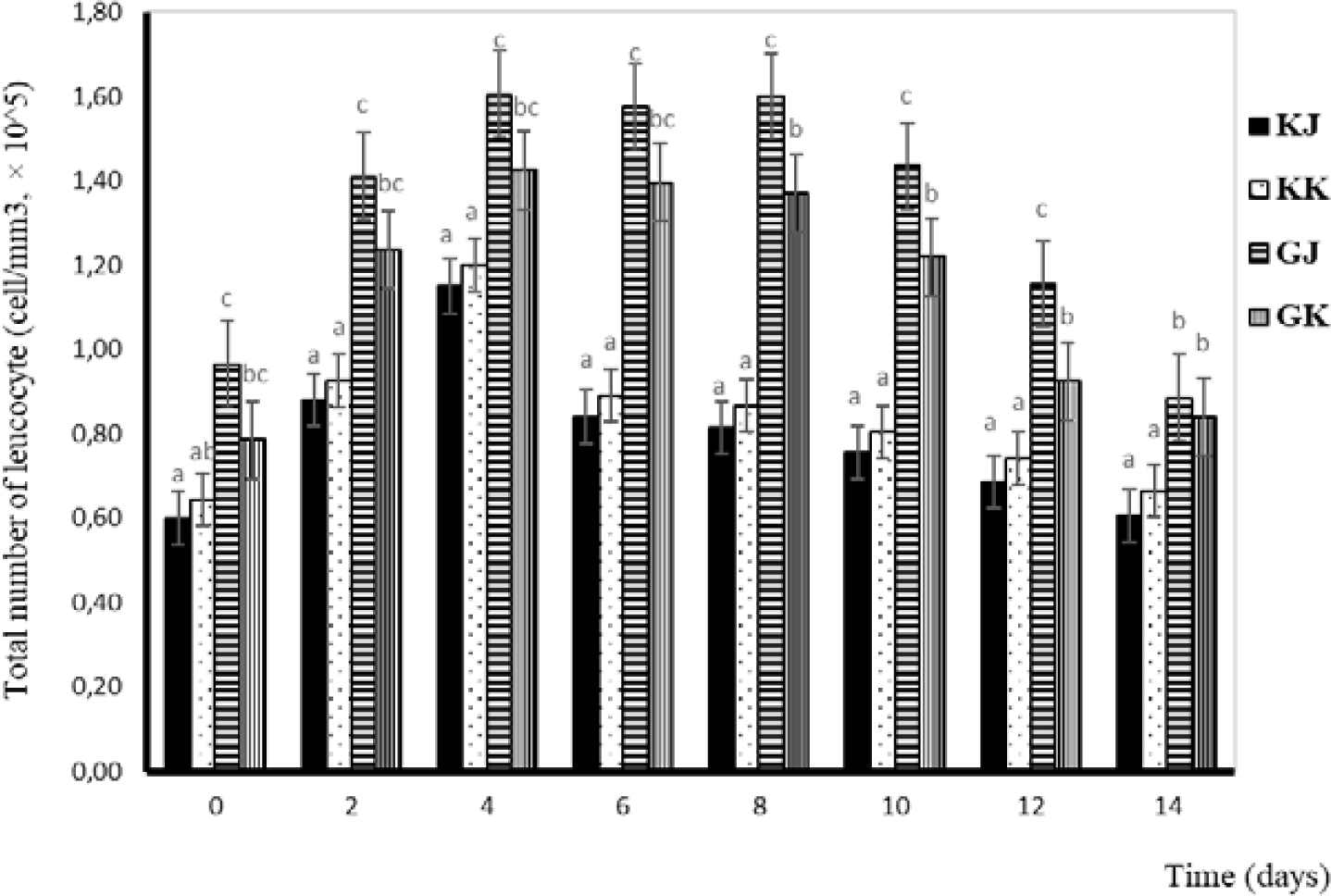
Hemoglobin is a critical component of red blood cells and is responsible for oxygen transport in the body. Its structure and function are tightly regulated, and any abnormalities in hemoglobin can have significant health implications. In this study, changes in hemoglobin levels have almost the same pattern as those in total erythrocytes. The lowest Hb level occurs on the fourth day and continues to increase until the 14th day. The Hb levels during the challenge test are displayed in Fig. 4.
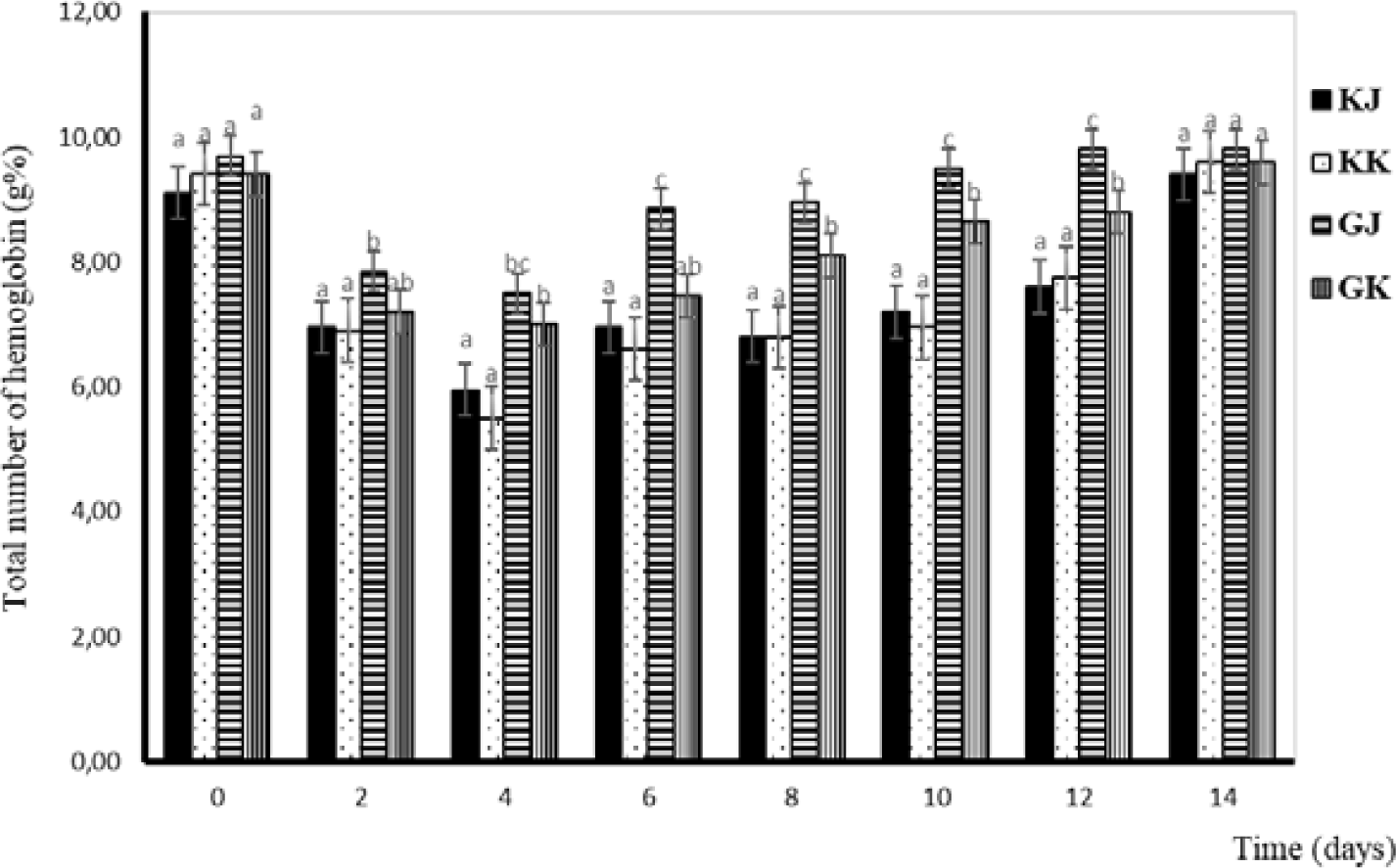
Hematocrit is a medical term that refers to the ratio of the volume of red blood cells to the total volume of blood. It is typically expressed as a percentage. Hematocrit is an important component of a complete blood count (CBC), which is a common blood test used to diagnose a variety of health conditions. Changes in hematocrit levels have almost the same pattern as total erythrocytes. The lowest hematocrit level occurs on the fourth day and continues to increase until the 14th day. The lowest hematocrit level was observed on day four and continued to increase until the day of the 14th. From a statistical perspective, there is no significant difference in the hematocrit levels between KK and KJ. However, substantial variations are evident when comparing the hematocrit levels of infected and uninfected gourami. Additionally, distinctions can be observed between GJ and GK. Hematocrit levels of the gourami during challenge testing with bacteria A. hydrophila are shown in Fig. 5.
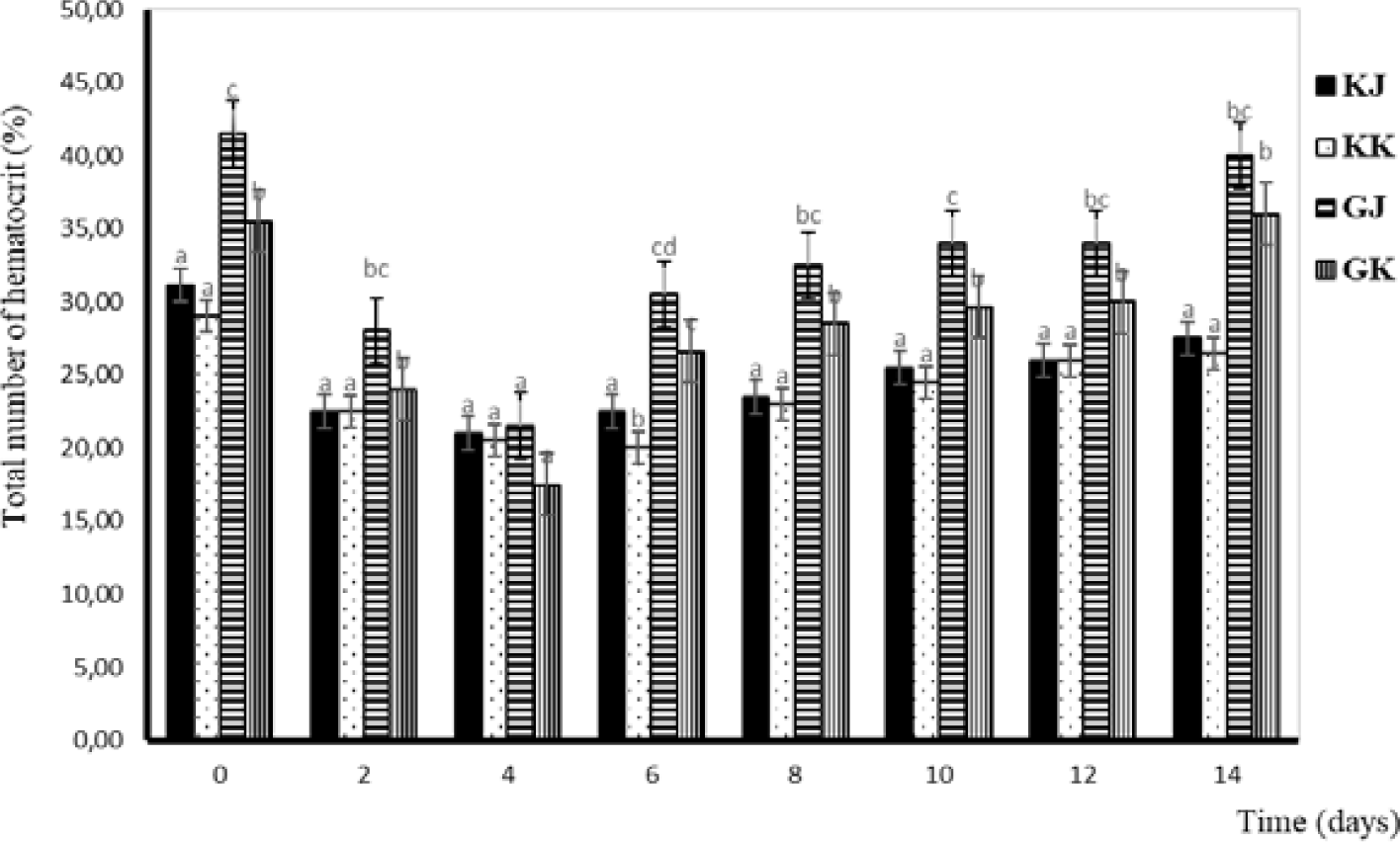
MHC II gene amplification using primer pairs (in the methodology) resulted in the expected amplicon. This indicates that the DNA templates of these individuals contain binding sites for the primers used. According to Jakaria et al. (2007), the success of DNA template amplification was influenced by complementary sites, thermal conditions, reaction components in PCR, and the PCR machine conditions used. The detection results of MHC II markings in resistant and susceptible gourami are presented in Fig. 6.
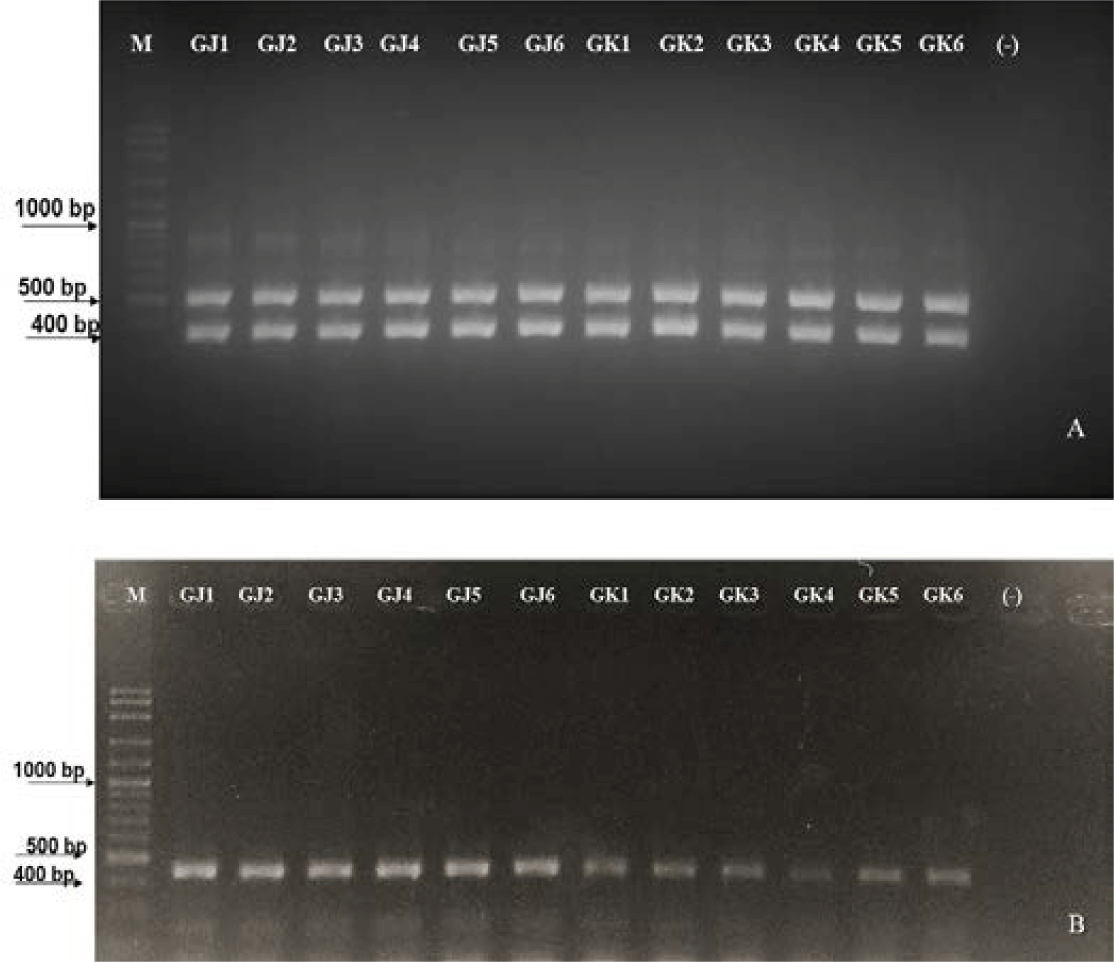
Water quality parameters were monitored to ensure fish deaths caused by A. hydrophila infection. The water quality parameters during the challenge test were within the acceptable ranges recommended for gourami farming, there were 7.0–8.1 mg/L of DO; 27.0°C–28.92°C of temperature; 7.90–8.55 of pH; 0.012–0.0195 mg/L of total ammonia; 0.01–0.041 mg/L of nitrite; and 0.04–0.188 mg/L of nitrate (Table 3).
Discussion
The challenge test through infection with pathogenic bacteria is one method to determine the level of resistance of fish to a pathogen. During the challenge test with A. hydrophila, the Kalimantan gourami (GK) fish population experienced a higher mortality rate compared to the Jambi gourami (GJ). The mortality data indicates that the GJ is more resistant to the pathogen. The difference in resistance level to A. hydrophila among GK and GJ was correlated to the genetic differentiation in each strain (Al-Harbi, 1996). Changes in the physical and chemical conditions of the environment can increase the abundance and virulence of pathogens, as well as genetic mutations (Tripathi et al., 2018). However, other factors, such as the level of host susceptibility, also affect the level of virulence of pathogens in causing disease (Tripathi et al., 2018). Therefore, disease in fish can be said to be the end result of the interaction between host susceptibility, pathogen virulence, and environmental factors (stressors) (Gjedrem, 2015).
The Jambi (GJ) and Kalimantan (GK) gourami used in this study were collected from areas with a significant geographic distance, namely Sumatra Island and Kalimantan Island. In previous research reported by Sularto et al. (2016), it was stated that gourami from Kalimantan showed faster growth performance compared to those from Jambi. This is suspected to be due to the fact that gourami from Kalimantan has undergone a relatively strict selection process related to growth characteristics, which may potentially result in the reduction of genes associated with resistance in that population.
In observing clinical symptoms, the Kalimantan gourami infected with A. hydrophila bacteria also showed more severe clinical symptoms than the Jambi gourami. Clinical symptoms that appear in fish infected with A. hydrophila bacteria begin with the appearance of white spots on the body, mucus throughout the body decreases, and the abdomen bulges swollen and yellowish white. The pectoral fins are whitened and there are red patches.
In addition to visually observed clinical symptoms, fish infected with A. hydrophila bacteria also show other symptoms on the inside of their bodies, namely yellow fluid in the abdominal cavity, pale red and flabby kidneys, brownish-red liver, heart, gills, pale intestines, a bulging stomach filled with water. Fish infected with A. hydrophila also experience changes in swimming patterns, including aggressive fish with an inflated dorsal fin or swimming weakly at the bottom of the aquarium.
Blood serves as a valuable means for assessing the health condition of fish, particularly when they are afflicted by diseases. Significant alterations in blood characteristics, such as hematocrit, hemoglobin, erythrocyte, and leukocyte counts, can indicate pathological conditions (Anderson & Siwicki, 1993). In pathological conditions, the total number of erythrocytes can deviate from normal levels, either increasing or decreasing depending on the causative factors. Salasia et al. (2001) stated that the normal number of erythrocytes in freshwater fish was 40.76–94.37 × 106 cells/mm3. The normal numbers of it in catfish and walking catfish were 72.76–73.04 × 104 and 2.31 × 106 cells/mm3, respectively (Alifuddin, 1999). In this study, the total number of erythrocytes in infected gourami was 1.40–2.48 cells/mm3, which was higher than in uninfected gourami where there were 1.31–2.01 × 106 cells/mm3 (Fig. 2). The function of erythrocytes is to play a role in carrying oxygen from the gills to the tissues. Erythrocytes contain carbonic acid, which functions to catalyze the reaction between carbon dioxide and water so that blood can transport carbon dioxide from tissues to gills in large quantities (Fujaya, 2004).
Hemorrhaging in fish infected with A. hydrophila resulted in ruptured blood vessels, and the number of red blood cells decreased after the challenge test (Azis, 2015). The presence of A. hydrophila, which produces the enzyme exotoxin hemolysin, can lyse erythrocytes and release hemoglobin so that the erythrocyte average of test fish generally decreases or is lower than normal until the 96th hour (Hardi, 2018). In addition, the low number of red blood cells is thought to be due to a bacterial infection that causes anemia. This phenomenon is in accordance with the research of Witeska et al. (2022), which states that low red blood cells are an indicator of anemia, while a high number of red blood cells indicates fish are in a state of stress.
The total number of leucocytes tends to increase after injection. This is due to the presence of an A. hydrophila infection, which causes fish to send more leucocytes to the area of infection as a defense effort. The leucocytes work as cells that phagocyte existing bacteria so that they do not develop and spread virulence in the host’s body (Hardi, 2018). When there are antigens that enter the body, white blood cells will respond by increasing their number as a form of defense. It was emphasized by Baratawidjaja (2002) that the immune response will increase by activating leucocytes. There is a difference in the number of leucocytes in infected fish (0.79–1.61 × 105 cells/mm3) and in uninfected fish (0.60–1.20 × 105 cells/mm3) (Fig. 3). The total number of leukocytes varies among fish species. According to Salasia et al. (2001), the total number of normal leucocytes in freshwater fish is 3,390–14,200 mm3. Normal leucocyte cell counts in walking catfish and catfish were 21,040–21,491 and 462,000 cells/mm3 respectively (Alifuddin, 1999).
The Hb levels in this study decreased in the early days and increased after the fourth-day post-injection. The increase in Hb is influenced by the increase in the number of erythrocytes in the body of fish, while low Hb results in anemic fish. Prasetio et al. (2017) stated that low Hb causes the metabolic rate to decrease, and the energy produced to be low. The condition makes the fish weak, have no appetite, and tend to the bottom or hang below the surface of the water. Salasia et al. (2001) stated that normal Hb in freshwater fish was 5.05–8.22 g%. Tilapia has an Hb value of 6–11 g%, walking catfish have an Hb of 10 g%, and catfish have an Hb of 11 g% (Lusiastuti & Hardi, 2010).
The lowest hematocrit level occurs on the fourth day and continues to increase until the 14th day. The hematocrit value is influenced by the size and number of erythrocytes (Witeska et al., 2015). Hematocrit is used to measure the ratio between erythrocytes and plasma or total blood volume in the body (Putranto & Syaputra, 2019). The hematocrit of infected gourami has decreased from normal since 48–96 hours, indicating that the fish has symptoms of anemia. According to Witeska (2015), anemia has an impact on inhibiting fish growth because the low number of erythrocytes results in a reduced food supply to cells, tissues, and organs, so the metabolic process of fish is inhibited. One of the causes of anemia is a lack of food. Fish injected with bacteria do not respond to the feed given (Hardi, 2018). The low value of hematocrit after injection can also be due to bleeding in the wound, such as in fish that show changes in anatomical pathology (Hardi, 2018).
Minaka et al. (2012) reported that the hematocrit level of normal gourami ranged from 33%–43%. Meanwhile, Nabib & Pasaribu (1989) stated that hematocrit levels below 30% indicate erythrocyte deficiency. In general, the average hematocrit at the time of the study was considered small, and it is suspected that the low hematocrit value is because the fish have anemia, nutritional deficiencies in feed, and are attacked by disease due to the bacterial infection A. hydrophila.
Fish mortality caused by A. hydrophila infection is confirmed by monitoring water quality measures. Referring to the standard level of the main water quality parameters, including DO, temperature, pH, ammonia, nitrite, and nitrate level, the rearing water condition during the challenge test of the gourami with A. hydrophila is still optimum for the fish (Boyd & Tucker, 1998). Optimal water quality parameters and the absence of death in uninfected fish indicate that fish mortality is definitely due to infection with the pathogen.
Widespread mortality resulting from A. hydrophila bacterial infections remains a prevalent issue in intensive gourami farming. Therefore, the search for molecular markers related to the resistance of gourami to pathogenic bacteria A. hydrophilah, the causative agent of MAS, is urgently needed to support the breeding program of disease-resistant gourami strains. Because MHC is the main gene that plays a role in the fish’s immune system against diseases, the search for resistant gourami fish markers to pathogen A. hydrophila is focused on MHC II markers. MHC II contributes to the stimulation of phagocytic cells for antibody production and immune system activation, participating in the eradication of parasites, and bacteria, and the neutralization of viruses (Rakus et al., 2009).
According to the results of MHC II gene amplification using the primer pair, the amplicon is right on target. Wegner et al. (2006), states that MHC gene amplification of the total genome of three-spine stickleback fish using a pair of primers obtained PCR products with a molecular weight of 360 bp. Meanwhile, amplification of gourami MHC genes using the same primer obtained PCR products with a molecular weight of 400 bp. This indicates that the DNA templates of these individuals have attachment sites for the primers used. It is stated by Jakaria et al. (2007) that the amplification of DNA templates is influenced by complementary sites, thermal conditions, reaction materials in PCR, and the conditions of the PCR machine used.
Based on Fig. 6, gouramis resistant to A. hydrophila bacterial infection have two bands, size at 400 bp and 585 bp, while gouramis susceptible to A. hydrophila bacterial infection have one band at 400 bp. The difference is caused by different DNA sequences that, when undergoing the translation process, will produce different protein codes. Fish, when fighting pathogens, must regulate transcription factors precisely (in a timely manner) after recognizing the pathogen to activate defense-related genes. Translational products will produce R genes (resistant genes) (Campbell et al., 2008). The role of the R gene is to be a receptor that will pair with the ligand of the pathogen, namely the avirulence (Avr) gene. The ligand’s binding to its receptor triggers a signal transduction pathway that produces a defensive response in infected fish. The number of amplification results shows that genes that control disease resistance are polygenes, meaning that disease resistance is controlled by many genes that react with each other to cause broad resistance.
There were large variations in the resistance characteristic of Jambi gourami (GJ) and Kalimantan gourami (GK) to A. hydrophila. The percentage of resistant fish in both populations is relatively small, which is the cause of the low survival of gourami when MAS infection occurs. Therefore, it is crucial to conduct a selection program aimed at creating a gourami strain resistant to A. hydrophila using MHC II primers.
Conclusion
Based on the fish survival rate and clinical signs during the challenge test, the Jambi strain gourami is more resistant to A. hydrophila bacterial infection than the Kalimantan strain gourami. The MHC II gene was detected in Jambi and Kalimantan gourami strains, both surviving and dying after the challenge test. The MHC II gene fragment with a molecular weight of about 585 bp is a specific molecular marker candidate for A. hydrophila-resistant gourami. The candidate for MHC II markers in gourami fish needs further research through verification and validation tests.