Introduction
Red seabream is a marine carnivore fish species originally distributed in East Asian region. It is an important aquaculture species in Japan and South Korea due to its unique taste and cultural importance. The total red seabream aquaculture production in South Korea was 6,283 metric tons in 2023 (KOSIS, 2024). In red seabream aquaculture, they were generally fed diets containing a high amount of conventional fish meal (FM) due to their strictly carnivore food habit (Hossain et al., 2019; Herault et al., 2023).
Aquaculture production in the world is currently dependent on the sustainable utilization of FM which is a limited resource facing increasing demand and constrained supply. Therefore, alternative protein sources are used in red seabream diet to expand its aquaculture while increasing the production. Both plant and animal-based protein sources were evaluated in red seabream diets as substitutes for FM over the last few decades.
Studies have been conducted since the late 1990s. Aoki et al. (1997) evaluated a mixture of soybean meal, meat meal (MM) and corn gluten (CG) in red seabream diet and reported that approximately 50%–60% of FM can be replaced from a control containing 65% FM. They also suggested that CG level in red seabream diet should be limited to 10%. Another study conducted by the same research team (Aoki et al., 1998) tested the possibility of defatted soybean meal and soy protein concentrate (SPC) as FM replacers in red seabream diet. Then, they reported that mixture of krill meal, defatted soybean meal, CG, MM, meat and bone meal, poultry feather meal and blood meal could replace 100% FM from red seabream diets after 10 weeks long feeding trial. Further studies on red seabream have reported that combined supplementation of alternative protein ingredients was more effective than dietary inclusion of single protein source (Takagi et al., 2000). Studies were then conducted using different other protein sources including insect meal (Takakuwa et al., 2022b), blood meal (Takakuwa et al., 2022a), dehulled soybean meal (Kader et al., 2012), algae meal (Seong et al., 2021) and rapeseed meal (Dossou et al., 2019) and their combinations to improve low FM diets for red seabream with time.
A mixture of protein sources, MM, chicken byproduct meal (CBM), SPC, and CG, which are available in the local market were evaluated in the present study. MM is produced using inedible flesh remaining after meat processing. MM is reported to improve fish and feed performance when it was used as a substitute for FM in their diets (Ha et al., 2021a; Rahman et al., 2022). CBM is also produced using processing waste which is not suitable for human consumption. Studies on CBM revealed successful results as a substitute for FM in diets for different fish species (Ha et al., 2021b). SPC is protein rich ingredient prepared by defatting and removing water soluble carbohydrates from soy flour. It was reported to replace 40%–70% of FM from red seabream diets after supplemented with other protein sources and functional feed additives (Biswas et al., 2019; Gunathilaka et al., 2021; Tola et al., 2019). CG meal is produced as a byproduct of corn processing which is used as animal feed ingredient. It was successfully used with other protein sources as substitute for FM in fish diets (Biswas et al., 2022).
Efficiency of FM replacement in fish diets is mainly dependent on the feed formulation. The efficiency is slightly affected by some other factors such as water temperature, photoperiod (Biswas et al., 2005), fish growth stage (Ishibashi et al., 2005), ingredient quality (Gunathilaka et al., 2021) and cost of production (Baek & Cho, 2024). Therefore, development of low FM diets including highly available protein sources in local market is a sustainable aquaculture practice when considering a country or a specific region. In this regard, we evaluated the efficacy of available protein sources as substitutes for FM in red seabream diet in a previous study (Gunathilaka et al., 2023). The study revealed that fish performance was not restored when 30% FM was replaced with CG as the sole FM replacer or as a mixture with SPC. A diet containing 30% FM and a mixture of MM, CBM, SPC, and CG in similar proportion improved growth performance and feed utilization of red seabream compared to a control diet containing 60% FM. Therefore, in accordance with these results, we designed the present study to evaluate an effective FM replacement level with the mixture of MM, CBM, SPC, and CG in diets for juvenile red seabream.
Materials and Methods
Three experimental diets containing approximately 49% crude protein and 13% crude lipid were prepared as shown in Table 1. Diets were formulated based on the previous study (Gunathilaka et al., 2023) with slight improvements. A diet formulated to contain 60% FM was considered as control (CON). Two other diets were designed by reducing FM levels to 40% and 20% with 5% or 10% of each MM, CBM, SPC, and CG to contain 20% or 40% protein source mixture in each diet (named as MX20 and MX40 respectively). The total crude protein levels were balanced with wheat flour. Diets were prepared after measuring the ingredients at the feed manufacturing laboratory of Gangneung-Wonju National University. Dry ingredients of each diet were then mixed by adding the required amount of fish oil, according to feed formulation, and distilled water in order to making doughs. Each dough was extruded using a noodle machine, crushed to a size of 3–5 mm before dry at 35°C for 12 h. Dry diets were kept at –20°C till the feeding trial began. Fatty acids and amino acids composition of diets are presented in Tables 2 and 3.
Vitamin mixture composition (unit/kg mix): ascorbic acid, 6,400 mg; tocopherol acetate, 37,500 mg; thiamin nitrate, 5,000 mg; riboflavin, 10,000 mg; pyridoxine hydrochloride, 5,000 mg; nicotinic acid, 37,500 mg; Ca-D-pantothenate, 17,500 mg; inositol, 75,000 mg; biotin, 50 mg; folic acid, 2,500 mg; menadione sodium bisulfite, 2,500 mg; retinol acetate, 5,000,000 IU; cholecalciferol, 1,000,000 IU; cyanocobalamin, 25 mg; riboflavin, 10,000 mg.
Proximate composition of protein sources are shown in Fig. 1.
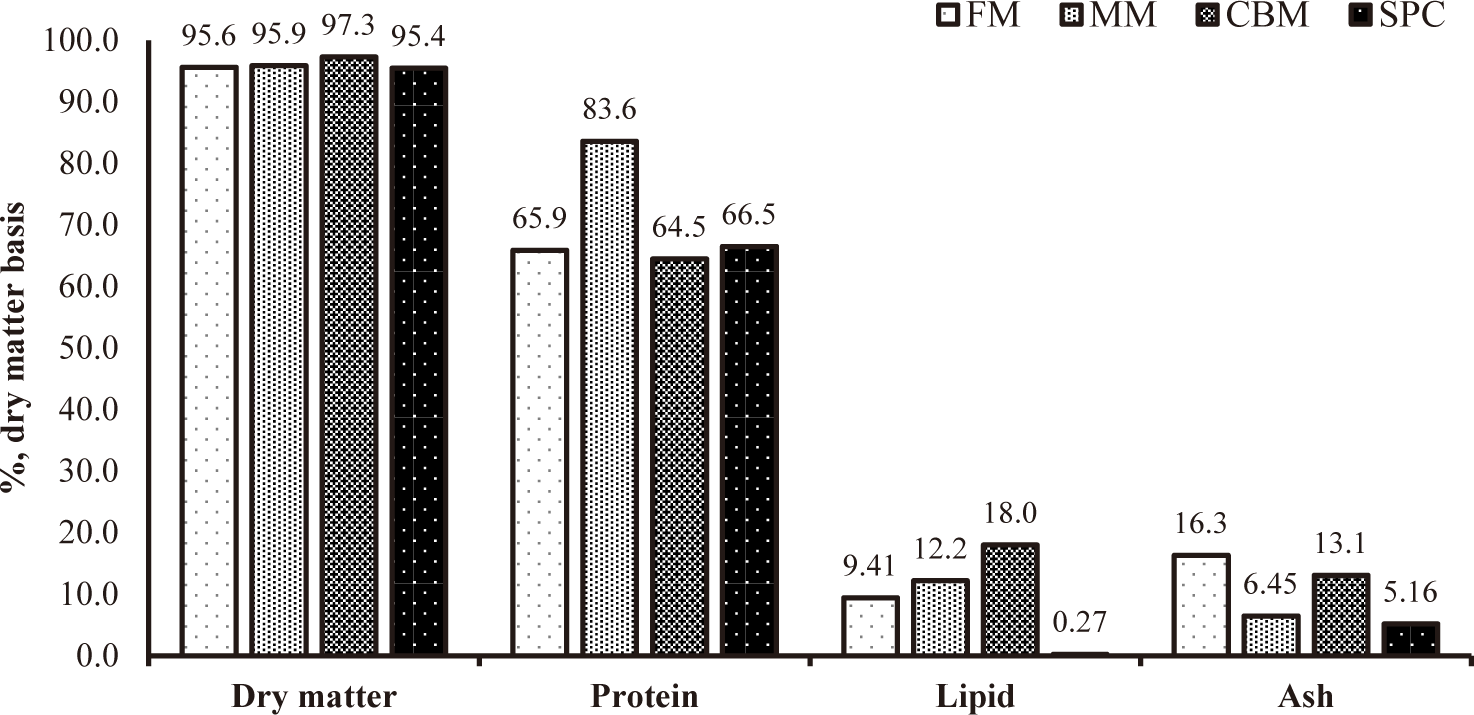
Juvenile red seabream were provided by a private hatchery (Uljin county, Korea). They were then stocked at the Research Facilities of Gangneung-Wonju National University, Marine Biology Center. Following an acclimation period for two weeks, juveniles, averaging 4.57 ± 0.03 g, were stocked at a density of 40 fish per tank in 9 tanks (300 L). Three diets were divided into three replicates, and each tank was randomly allocated to one of them. Fish were fed one of the experimental diets until satiation (twice a day at 09:00 and 17:00 h) for eight weeks. The photoperiod was adjusted to match the season’s actual day duration as precisely as possible. Water temperature (17.7°C–21.3°C), pH (7.47–7.87), and dissolved oxygen (6.57–7.29 mg/L) were recorded daily.
Fish were fasted for 24 h after the trial period, counted, and weighed for the calculation of final body weight (FBW), survival and other parameters including weight gain (WG), specific growth rate (SGR) feed intake (FI), feed efficiency (FE) and protein efficiency ratio (PER). Randomly selected sets of six fish from each tank were frozen at −20°C for later analysis of muscle proximate composition, amino acids, and fatty acid levels. Blood samples were collected from six individual fish from each experimental tank using heparinized syringes to separate the plasma for subsequent hematological analyses. Another six fish were captured and collected blood samples with non-heparinized syringes to separate serum samples for analysis of non-specific immune parameters. Remaining fish in all tanks were sedated separately, total lengths were measured to closest 0.1 mm and they were dissected to weighed viscera and livers to calculate condition factor (CF) and organosomatic indices.
Standard procedures were followed to assess the moisture and ash content of fish muscle samples and experimental diets (AOAC, 2005). A Kjeltec Analyzer (Buchi, Flawil, Switzerland) was used to test crude protein, and a Soxhlet extractor (VELP Scientifica, Milano, Italy) was used to analyze crude lipid. The fatty acid composition of the experimental meals and muscle samples was examined using a gas chromatographic method. Amino acid compositions were analyzed using automatic amino acid analyzer (Hitachi, Tokyo, Japan). Lysozyme activity of serum samples was measured on the basis of turbidimetric technique with Micrococcus lysodeikticus (Sigma-Aldrich, St. Louis, MO, USA) as a substrate (Khosravi et al., 2015). Superoxide dismutase activity (SOD) of serum samples was analyzed with an assay kit (Sigma 19,160). An automated blood analyzer was used to analyze plasma biochemical parameters including glutamic-oxaloacetic transaminase, alkaline phosphatase, total cholesterol, triglycerides, glutamate-pyruvate transaminase, total protein, albumin and glucose levels.
Data were analyzed using one-way analysis of variance. Duncan’s multiple range test (Duncan, 1955) was performed to identify any significant variations in the mean values of the parameters, using SPSS version 20.0 (SPSS, Chicago, IL, USA). Statistical significance was determined at p < 0.05. Data were presented as mean ± standard error (SE). Arcsine transformation was performed on percentage data prior to statistical analysis.
Results
Growth performance and feed utilization of red seabream are presented in Table 4. FBW, WG and SGR of fish fed CON and MX20 diets were significantly higher than those of fish fed MX40 diet. FI of fish in CON group was significantly higher compared to MX40 group. However, FE, PER and survival were not significantly different.
Values with different superscripts in the same row are significantly different (p < 0.05). The lack of superscript letters indicates no significant differences among treatments.
Results of lysozyme and SOD activities are shown in Table 5. Fish in CON group exhibited slightly improved lysozyme activity. However, both lysozyme and SOD activities were not significantly different among dietary treatments.
Lysozyme1) | SOD2) | |
---|---|---|
CON | 38.6 ± 1.23 | 59.2 ± 2.67 |
MX20 | 36.5 ± 1.39 | 59.8 ± 1.78 |
MX40 | 35.8 ± 0.81 | 56.6 ± 0.59 |
Results of plasma biochemical analyzes are presented in Table 6. Only the TG level was significantly higher in fish fed CON diet compared to that of MX40 group. Other parameters were not significantly changed by the diets at the end of the feeding trial. However, total cholesterol level exhibited a decreasing trend and glucose level exhibited an increasing trend with the increase of ingredient mixture in diet.
Results of muscle proximate analysis of fish fed the experimental diets are presented in Table 7. Muscle dry matter, protein, lipid or ash levels were not significantly affected by dietary treatments although ash level exhibited decreasing level with the increase of ingredient mixture in diet.
Dry matter | Protein | Lipid | Ash | |
---|---|---|---|---|
CON | 24.2 ± 0.39 | 19.9 ± 0.66 | 2.31 ± 0.41 | 1.78 ± 0.14 |
MX20 | 24.3 ± 0.47 | 19.3 ± 0.71 | 2.11 ± 0.37 | 1.77 ± 0.19 |
MX40 | 24.1 ± 0.92 | 18.8 ± 0.69 | 2.09 ± 0.31 | 1.80 ± 0.16 |
Muscle fatty acid profile of fish fed experimental diets are shown in Table 8. Palmitic acid (16:0) level of fish fed CON diet was significantly lower than that of fish fed MX20 and MX40 diets. Dihomo-gamma-linolenic acid (20:3 n-6) was significantly higher in CON group compared to that of fish fed MX20 and MX40 diets. Other than these two, other fatty acids were not significantly affected by experimental diets although there were some slight variations. Docosanoic acid (22:0) was not detected in fish fed MX40 diet and eicosatrienoic acid (20:3 n-3) was not detected in that of fish groups fed both MX20 and MX40 diets.
Results of muscle amino acid analysis are shown in Table 9. Amino acid profiles were not significantly different among fish groups fed three experimental diets at the end of 8 weeks feeding trial.
Biometric parameters of fish are shown in Table 10. CF, HSI, or VSI were not significantly affected by three experimental diets at the end of feeding trial. However, CF level was slightly reduced with the increase of ingredient mixture in diet.
CF1) | HSI2) | VSI3) | |
---|---|---|---|
CON | 1.75 ± 0.22 | 1.63 ± 0.31 | 7.74 ± 0.88 |
MX20 | 1.68 ± 0.12 | 1.65 ± 0.42 | 6.92 ± 0.72 |
MX40 | 1.58 ± 0.08 | 1.82 ± 0.39 | 7.06 ± 0.66 |
Discussion
Growth performance of red seabream fed CON diet was restored by inclusion of 20% MX mixture. Inclusion of 40% MX mixture resulted in significantly lower performance compared to the CON diet indicating that optimum inclusion level was less than 40% and higher than 20% in diets. In the previous study, we observed restored growth performance when diets were prepared with 30% of MX mixture (Gunathilaka et al., 2023). However, in the present study, experimental diets were prepared with slight variations compared to the previous formulation. Fish growth stages were also different in both studies. Therefore, proper inclusion level of MX mixture in red seabream diet might be lies within 20%–40%. Fatty acid and amino acids are one of main reasons by which fish growth performance was accelerated (Gunathilaka et al., 2019; Kotani et al., 2013; Takagi et al., 2001). Imbalance of fatty acids or amino acids in diets resulted in reduced growth performance in fish (Glencross et al., 2003; Kader et al., 2012). In the present study, CON diet was prepared by adding soybean oil. MX20 and MX40 diets were prepared replacing soybean oil from fish oil to balance marine originated lipid levels. However, EPA and DHA levels were gradually reduced with the increase of alternative protein sources in diet (Table 2) while amino acid profiles exhibit slight variations. EPA and DHA enhance the growth performance of fish (Kotani et al., 2013). The reduced growth performance observed in the MX40 group might be attributed to low EPA and DHA levels. Huang et al. (2007) observed that replacement of dietary fish oil with canola oil had no significant influences on growth performance of red seabream when diets contained 50% FM. Mzengereza et al. (2021) reported that total fish oil level in red seabream diets can be replaced with camelina oil and additional EPA, DHA and highly unsaturated fatty acid when diets contained 46% FM and 20% soybean meal. Therefore, we suggest that MX40 diet should be modified with additional EPA and DHA to increase growth performance of red seabream. Moreover, CG level was 10% in MX40 diet prepared in the present study. in the previous study, we observed that high level of CG in diets resulted in reduced growth performance of red seabream (Gunathilaka et al., 2023). Aoki et al. (2000) also reported that CG level in red seabream diets should not exceed 10%. The reduced growth performance in fish fed MX40 diet might also be attributed to high GC level. In contrast, we formulated the control diet including 5% CG (Table 1). It is well known that CG can be included in diets containing high FM levels without adverse effects on fish (Gunathilaka et al., 2019; Huang et al., 2007). Future studies should be conducted with additional essential fatty acids and low CG level to evaluate these assumptions.
The FI of red seabream was reduced with the increase of MX mixture in diets. Low FI is one of the main reasons to reduce growth performance of fish. FE and PER were not significantly reduced in MX diets in the present study indicating that the FI was one of reasons to reduce growth performance of fish fed MX40 diet. FI was reduced due to low palatability (Biswas et al., 2019). High level of alternative protein sources in diets resulted in reduced palatability in red seabream as represented by low FI (Biswas et al., 2019; Gunathilaka et al., 2021). Addition of palatability enhancers in low FM diets was reported as an effective strategy to improve palatability of red seabream diet. Reports are available introducing different types of palatability enhancers including protein sources, protein hydrolysates (Herault et al., 2023), amino acids (Takagi et al., 2001), taurine (Gunathilaka et al., 2019), organic acids (Hossain et al., 2007), mineral compounds (Hossain et al., 2007) and plant derived compounds (Seong et al., 2020). We suggest adding one or more palatability enhancers in MX diets to improve FI of fish in future studies. Feed utilization as represented by FE and PER was not significantly deteriorated due to FM replacement in the present study although the values were slightly reduced. Mixtures of several feed ingredients were reported to enhance feed utilization compared to a single ingredient (Gunathilaka et al., 2023). Feed utilization was increased in red seabream when nutrient digestibility of ingredients and diets was high. MM and SPC were reported to exhibit approximately 75% ingredient digestibility which was higher than several other plant protein ingredients in olive flounder (Paralichthys olivaceus) (Rahman et al., 2022, 2023). Kim et al. (2010) reported that nutrient digestibility of MM and CG in olive flounder were approximately 73% and 60% respectively. Therefore, feed utilization can be lower when diets contain high CG compared to MM, CBM and SPC. It was also reported that nutrient digestibility of low FM diets containing high SPC levels were significantly lower than diets supplemented with SPC and palatability enhancers or feed attractants (Gunathilaka et al., 2021; Herault et al., 2023) by which provide amino acids, fatty acids and/or other compounds to accelerate digestion process. Accordingly, we assumed that the addition of palatability enhancer in MX diets will improve utilization of MX diets in addition to enhancing FI.
Serum lysozyme and SOD activities were not significantly affected by experimental diets in the present study. The results indicate that immune status of red seabream was not influenced by replacement of FM or inclusion of alternative protein sources. Number of studies reported that low FM diets containing high levels of plant or animal protein sources causes significant malfunctions in red seabream immune mechanism with reduced lysozyme and/or SOD activities (Dossou et al., 2019; Khosravi et al., 2015). Several studies observed no significant influences on immune parameters of red seabream after feeding low FM diets (Hossain et al., 2019; Sony et al., 2020). Hossain et al. (2019) assumed that dietary inclusion of inosine monophosphate was the reason for unaffected lysozyme activity due to FM replacement. Sony et al. (2020) did not observe significant changes in lysozyme activity of red seabream after feeding low FM diets containing fucoidan extracted from mozuku. Feed formulations of both studies contained increasing levels of lysine and methionine in diets indicating that the functional feed additive was important to maintain immune functions of fish even after replacing 100% of FM. In the previous study, we observed significantly improved lysozyme activity and comparable SOD activity in fish fed diets containing the same ingredient mixture without lysine or methionine and with vitamin C (Gunathilaka et al., 2023). Therefore, it is debatable to conclude about the exact reason as the effects of diets can be different according to several other factors including growth stage and environmental conditions and ingredient quality (Biswas et al., 2005; Gunathilaka et al., 2021; Ishibashi et al., 2005). We assumed that the functional feed ingredients might have played a positive role in fish to maintain immune parameters unaffected with FM replacement down to 20% in the present study.
Plasma triglyceride level of red seabream was gradually decrease with increase of protein mixture in diets. MX40 group exhibited significantly lower values compared to CON group. Total cholesterol level also exhibited decreasing trend although values were not significant among three dietary groups. Triglyceride is the absorption form of lipid which was synthesized using monoglycerides and fatty acids in enterocytes. Triglycerides then circulate throughout the body with blood. Excess triglycerides were stored in adipose tissues and liver. The stored triglycerides were converted to glycerides and fatty acids in events of energy requirements (Alves-Bezerra & Cohen, 2017). Therefore, the triglyceride level can be affected due to these three events namely lipid digestion, storage and excess energy requirement. An enzyme called lipoprotein lipase is responsible for converting triglyceride to fatty acids and glycerol. Expression of gene related to lipoprotein lipase and plasma triglyceride levels of gilthead seabream was significantly changed with season and time of year (Saera-Vila et al., 2005). They also observed significantly lower lipoprotein lipase expression in adipose tissue and significantly higher expression in liver when fish fed low FM diets compared to high FM diets indicating that the protein sources can affect triglyceride metabolism in fish. It was reported that high levels of plant protein in diets induce liver steatosis and hypotriglyceridaemia in fish (Seong et al., 2020). Accordingly, triglyceride level might be reduced in fish fed diets containing high plant protein in the present study. However, liver lipid level should be investigated to prove the assumption in future studies because high levels of plasma triglycerides was observed in red seabream after feeding SPC based non-FM diets (Tola et al., 2019) compared to control diet containing high level of FM.
Muscle proximate and amino acid compositions were not significantly affected by the dietary treatments. Muscle palmitic acid level was significantly lower in CON group while dihomo-gamma-linolenic acid was significantly higher compared to MX20 and MX40 reflecting the pattern of fatty acid in the diets. Other fatty acids such as EPA and DHA levels were gradually changed after FM replacement with ingredient mixture in the MX20 and MX40 diets. The changes were not exhibited in the muscle composition. Similarly, the differences in fatty acid profile in diets were not reflected in muscle samples in the previous study (Gunathilaka et al., 2023). We assumed that the muscle growth was limited with the fatty acid availability. Huang et al. (2007) observed no significant effects of oil source to whole-body crude lipid level in red seabream. However, they observed that fatty acid concentration in whole-body and liver were significantly affected reflecting dietary composition after feeding diets containing increasing canola oil. In the present study, we did not analyze whole-body or liver fatty acid levels. It is well known that excess fatty acids which are not utilized were stored in liver and mesenteric adipose tissue of fish (Medagoda et al., 2022; Sarker et al., 2011). Therefore, whole-body or liver fatty acid levels might reflect the dietary composition although muscle samples contain fatty acid used to build structure and remain in cells. In contrast, Martínez-Llorens et al. (2007) observed significantly different muscle fatty acid levels when fish oil was replaced with soybean oil in gilthead seabream diet. In their study, final body weight was significantly reduced with high dietary soybean oil level. Compared to present study, Martínez-Llorens et al. (2007) prepared all diets to contain similar levels of protein sources indicating that oil source was only the reason for significant changes in muscle fatty acid levels. However, the experimental diets in the present study were formulated with different levels of protein sources, and fish oil were added to compensate the reducing marine originated lipid levels due to FM replacement. Therefore, discrepancies with the previous study might be attributed to the ingredient composition of diets formulated in the present study. We suggest that muscle fatty acid levels should be investigated in red seabream fed different dietary lipid and protein sources in future studies.
In summary, FM level in red seabream diet was successfully reduced to 40% with the ingredient mixture. Growth performance was adversely affected with further FM replacement down to 20%. Therefore, further studies should be conducted to determine proper FM replacement level in between 40%–20% and to improve efficiency of protein source mixture. However, feed utilization, immune parameters and muscle composition were not significantly influenced by the protein source mixture indicating that FM level in diets can be reduced to 20% with the ingredient mixture without adverse effects on these parameters.