Introduction
Oxidative stress is related with the cause of several diseases and decreasing oxidative stress is also essential role to maintain human health (Pizzino et al., 2017). It is normally caused by the accumulation of reactive oxygen species (ROS) including superoxide, hydrogen peroxide, and free radicals. In particular, free radicals are very reactive and unstable (Dong et al., 2022; Halliwell, 2022; Yang & Lian, 2020). These radicals can trigger structural instability to receive and donate electrons in normal molecules. The accumulation of free radicals induces a disparity between the ROS and anti-oxidant level. They damage the cellular macromolecules, lipids, gene expression pathways, and nucleic acid (Schieber et al., 2014; Simioni et al., 2018; Tryfidou et al., 2020). Consequently, several harmful effects according to accumulation of ROS level are highly related to various diseases including diabetes, asthma, atherosclerosis, pulmonary diseases, neurodegenerative diseases, and caner (Batty et al., 2022; Kim et al., 2015; Nakamura & Takada, 2021; Qu et al., 2017; Taniguchi et al., 2021; Volpe et al., 2018). Antioxidants, which are known free radical scavengers, are important constituents to protect the oxidation. It plays critical role in removing or altering the oxidative stress related to diverse free radicals. Halophytes are known to produce the various biological secondary metabolites due to salt-tolerance condition (Patel et al., 2022; Ravi et al., 2020). Therefore, halophytes are rich sources of natural antioxidant. The halophytes are comprised with about 72 species in South Korea (Lee et al., 2019). Calystegia soldanella is a perennial plant which generally grows with a procumbent stem (50–100 cm long) and reniform-shaped leaves. They are distributed with temperate regions (South Korea, Japan, and Europe). The root of C. soldanella have been treated as edible and medicinal herbs to cure the dropsy, antipyretic, and diuretic effects (Eom et al., 2021; Lee et al., 2017). In previous papers, the chemical constituents of C. soldanella have been known to possess diverse type chemical skeletons (resin glycosides, flavonoids, caffeic ester, and courmaric acid esters) and diverse bioactivities including anti-diabetic, anti-cancer, anti-viral, and anti-inflammatory activities (Lee et al., 2014; Murai et al., 2015; Ono et al., 2014; Tori et al., 2010). In the current study, we reported the chemical characterization of flavonoid and quinic acid derivatives (1−8) from the aerial parts of C. soldanella with antioxidant activities.
Materials and Methods
The nuclear magnetic resonance (NMR) spectra were generated using Varian VNMRS 500 MHz FT-NMR spectrometer (Varian, Palo Alto, CA, USA). The high-performance liquid chromatography (HPLC; Waters, Miliford, MA, USA) with a PDA detector and C18 column (Cosmosil, Kyoto, Japan) was analyzed using extract and isolated compounds. MPLC (Biotage, Uppsala, Sweden) and preparative-HPLC (Shimadzu, Kyoto, Japan) were performed to isolation and purification. The HPLC of elution solvents were used for the HPLC grade (ACN, Water, MeOH, J. T. Baker®, Phillipsburg, NJ, USA) and Mass elution solvents were used by mass grade (ACN, MeOH, Supelco, Bellefonte, PA, USA). The HR-ESI-MS spectra were measured on a SCIEX X500R Q-TOF LC-MS/MS spectrometer (SCIEX, Framingham, MA, USA).
Aerial parts of C. soldanella were collected from Ujeong-eup, Hwaseong-si, Gyeonggi-do, Korea in September 2019. A voucher specimen (NP-0682) was kept in the National Biodiversity institute of Korea.
The aerial parts of C. soldanella (150 g) were cut and extracted three times with 70% EtOH at room temperature for 8 h. The 70% EtOH extract was successively partitioned with n-hexane, chloroform, ethyl acetate, and n-butanol. The most active portion (n-BuOH-soluble layer) was chromatographed by MPLC eluting with an increasing MeOH in water to give seven fractions (S1–S7). The mixed fraction was further followed by Sephadex LH-20 column chromatography (eluent: MeOH) to yield two compounds 5 (27.6 mg), 6 (2.7 mg), and nine subfractions (S1–S9). The subfraction 5 was finally isolated using preparative-HPLC with increasing solvents (2:8/MeOH:Water → 5:5/MeOH:Water) to obtain four compounds 1 (9.6 mg), 2 (1.2 mg), 3 (2.9 mg), and 4 (14.5 mg). The subfraction 8 was further divided with preparative-HPLC eluted with increasing solvents (3:7/MeOH:Water → 65:35/MeOH:Water) in water to obtain two compounds 7 (5.0 mg) and 8 (3.7 mg).
Chlorogenic acid (1): HR-ESI-MS (negative) m/z 353.0878 [M-H]–, calcd for C16H17O9 353.0873; 1H NMR (500 MHz, CD3OD): δ7.56 (1H, d, J = 15.9 Hz, H-7’), 7.05 (1H, d, J = 1.8 Hz, H-2’), 6.95 (1H, dd, J = 8.2, 1.8 Hz, H-6’), 6.77 (1H, d, J = 8.2 Hz, H-5’), 6.27 (1H, d, J = 15.9 Hz, H-8’), 5.34 (1H, m, H-3), 4.17 (1H, m, H-5), 3.73 (1H, m, H-4), 2.20/2.06 (2H, m, H-2), 2.18/2.05 (2H, m, H-6); 13C NMR (125 MHz, CD3OD): δ177.5 (C-7), 168.8 (C-9’), 149.7 (C-4’), 147.2 (C-7’), 146.9 (C-3’), 127.9 (C-1’), 123.1 (C-6’), 116.5 (C-5’), 115.3 (C-8’), 115.2 (C-2’), 76.4 (C-1), 73.7 (C-4), 72.1 (C-3), 71.6 (C-5), 39.0 (C-6), 38.4 (C-2) (Supplementary Figs. S1-S3).
Cryptochlorogenic acid (2): HR-ESI-MS (negative) m/z 353.0880 [M-H]–, calcd for C16H17O9 353.0873; 1H NMR (500 MHz, CD3OD): δ7.63 (1H, d, J = 15.9 Hz, H-7’), 7.07 (1H, d, J =1.7 Hz, H-2’), 6.97 (1H, dd, J = 8.1, 1.7 Hz, H-6’), 6.78 (1H, d, J = 8.1 Hz, H-5’), 6.37 (1H, d, J = 15.9 Hz, H-8’), 4.86 (1H, m, overlap, H-3), 4.30 (1H, m, H-4), 4.21 (1H, m, H-2), 2.14-2.02 (4H, m, H-1 and 5); 13C NMR (125 MHz, CD3OD): δ177.4 (C-7), 169.0 (C-9’), 149.7 (C-4’), 147.2 (C-7’), 146.9 (C-3’), 128.0 (C-1’), 123.1 (C-6’), 116.6 (C-5’), 115.5 (C-8’), 115.2 (C-2’), 78.9 (C-3), 75.9 (C-6), 69.3 (C-4), 66.4 (C-2), 39.2 (C-5), 38.7 (C-1) (Supplementary Figs. S4-S7).
5-O-(E)-p-coumaroylquinic acid (3): HR-ESI-MS (negative) m/z 337.0924 [M-H]–, calcd for C16H17O8 337.0923; 1H NMR (500 MHz, CD3OD): δ7.63 (1H, d, J = 15.9 Hz, H-7’), 7.46 (2H, d, J = 8.5 Hz, H-2’ and H-6’), 6.80 (2H, d, J = 8.5 Hz, H-3’ and H-5’), 6.34 (1H, d, J = 15.9 Hz, H-8’), 5.34 (1H, m, H-3), 4.16 (1H, m, H-5), 3.72 (1H, dd, J = 8.5, 2.6 Hz), 2.22-2.02 (4H, m, H-2 and H-6); 13C NMR (125 MHz, CD3OD): δ179.2 (C-7) 168.8 (C-9’), 161.4 (C-4’), 146.7 (C-7’), 131.3 (C-2’ and 6’), 127.3 (C-1’), 116.9 (C-3’ and 5’), 115.5 (C-8’), 75.8 (C-1), 73.9 (C-4), 72.3 (C-3), 71.9 (C-5), 39.3 (C-2), 38.5 (C-6) (Supplementary Figs. S8-S11).
Rutin (4): HR-ESI-MS (negative) m/z 609.1449 [M-H]–, calcd for C27H29O16 609.1456; 1H NMR (500 MHz, CD3OD): δ7.67 (1H, d, J = 1.9 Hz, H-2’), 7.63 (1H, dd, J = 8.4, 2.0 Hz, H-6’), 6.87 (1H, d, J = 8.4 Hz, H-5’), 6.38 (1H, d, J = 1.8 Hz, H-8), 6.20 (1H, d, J = 1.8 Hz, H-6), 5.11 (1H, d, J = 7.6 Hz, H-1’’), 4.52 (1H, d, J = 1.0 Hz, H-1’’’), 3.81/3.39 (2H, m, H-5’’), 3.64 (1H, m, H-2’’’), 3.55 (1H, m, H-3’’’), 3.47 (1H, m, H-2’’), 3.45 (1H, m, H-5’’’), 3.44 (1H, m, H-3’’), 3.33 (1H, m, H-5’’), 3.30 (1H, m, H-4’’’), 3.29 (1H, m, H-4’’), 1.12 (3H, d, J = 6.2 Hz, H-6’’’); 13C NMR (125 MHz, CD3OD): δ179.5 (C-4), 166.1 (C-7), 163.0 (C-5), 159.4 (C-9), 158.6 (C-2), 149.9 (C-4’), 145.9 (C-3’), 135.7 (C-3), 123.6 (C-6’), 123.2 (C-1’), 117.8 (C-2’), 116.1 (C-5’), 105.7 (C-10), 104.8 (C-1’’), 102.5 (C-1’’’), 100.0 (C-6), 94.9 (C-8), 78.2 (C-3’’), 77.3 (C-5’’), 75.8 (C-2’’), 74.0 (C-4’’’), 72.3 (H-3’’’), 72.2 (H-2’’’), 71.5 (H-4’’), 69.8 (H-5’’’), 68.6 (H-6’’), 18.0 (H-6’’’) (Supplementary Figs. S12-S14).
Isoquercetin (5): HR-ESI-MS (negative) m/z 463.0873 [M-H]–, calcd for C21H19O12 463.0877; 1H NMR (500 MHz, CD3OD): δ7.71 (1H, brs, H-2’), 7.57 (1H, d, J = 7.3 Hz, H-6’), 6.86 (1H, d, J = 7.3 Hz, H-5’), 6.36 (1H, brs, H-8), 6.17 (1H, brs, H-6), 5.25 (1H, d, J = 6.6 Hz), 3.72/3.59 (2H, m, H-6’’), 3.46 (1H, m, H-2’’), 3.44 (1H, m, H-3’’), 3.35 (1H, m, H-4’’), 3.25 (1H, m, H-5’’); 13C NMR (125 MHz, CD3OD): δ179.5 (C-4), 166.1 (C-7), 163.0 (C-5), 159.0 (C-2), 158.5 (C-9), 149.5 (C-4’), 145.9 (C-3’), 135.7 (C-3), 123.2 (C-6’), 123.1 (C-1’), 117.6 (C-2’), 116.0 (C-5’), 105.7 (C-10), 104.4 (C-1’’), 100.0 (C-6), 94.8 (C-8), 78.4 (C-5’’), 78.1 (C-3’’), 75.8 (C-2’’), 71.5 (C-4’’), 62.6 (C-6’’) (Supplementary Figs. S15-S17).
Nicotiflorin (6): HR-ESI-MS (negative) m/z 593.1501 [M-H]–, calcd for C27H29O15 593.1507; 1H NMR (500 MHz, CD3OD): δ8.07 (2H, d, J = 8.7 Hz, H-2’ and H-6’), 6.89 (2H, d, J = 8.7 Hz, H-3’ and H-5’), 6.40 (1H, brs, H-8), 6.21 (1H, brs, H-6), 5.13 (1H, d, J = 7.4 Hz, H-1’’), 4.51 (1H, brs, H-1’’’), 3.81/3.38 (2H, m, H-6’’), 3.64 (1H, m, H-2’’’), 3.52 (1H, m, H-3’’’), 3.45 (1H, m, H-5’’’), 3.44 (1H, m, H-2’’), 3.42 (1H, m, H-3’’), 3.33 (1H, m, H-5’’), 3.29 (1H, m, H-4’’’), 3.27 (1H, m, H-4’’), 1.12 (3H, d, J = 6.2 Hz); 13C NMR (125 MHz, CD3OD): δ179.5 (C-4), 166.2 (C-7), 163.1 (C-5), 161.6 (C-4’), 159.5 (C-9), 158.6 (C-2), 135.6 (C-3), 132.5 (C-2’ and C-6’), 122.8 (C-1’), 116.2 (C-3’ and C-5’), 105.7 (C-10), 104.7 (C-1’’), 102.5 (C-1’’’), 100.1 (C-6), 95.0 (C-8), 78.2 (C-3’’), 77.3 (C-5’’), 75.9 (C-2’’), 74.0 (C-4’’’), 72.4 (C-3’’’), 72.2 (C-2’’’), 71.5 (C-4’’), 69.8 (C-5’’’), 68.7 (C-6’’), 18.0 (C-6’’’) (Supplementary Figs. S18-S20).
Isochlorogenic acid A (7): HR-ESI-MS (negative) m/z 515.1188 [M-H]–, calcd for C25H23O12 515.1190; 1H NMR (500 MHz, CD3OD): δ7.62 (1H, d, J = 16.0 Hz, H-3’), 7.58 (1H, d, J = 16.0 Hz, H-3’’), 7.07 (2H, s, H-9’ and H-9’’), 6.96 (2H, m, H-5’ and H-5’’), 6.78 (2H, d, J = 8.0 Hz, H-6’ and H-6’’), 6.36 (1H, d, J = 16.0 Hz, H-2’), 6.27 (1H, d, J = 16.0 Hz, H-2’’), 5.42 (1H, m, H-3), 5.40 (1H, m, H-5), 3.97 (1H, m, H-4), 2.32/2.15 (2H, m, H-2), 2.23/2.19 (2H, m, H-6); 13C NMR (125 MHz, CD3OD): δ177.8 (C-7), 169.0 (C-1’), 168.5 (C-1’’), 149.7 (C-7’), 149.6 (C-7’’), 147.3 (C-3’), 147.1 (C-3’’), 146.9 (C-8’ and C-8’’), 128.0 (C-4’), 127.9 (C-4’’), 123.2 (C-5’), 123.1 (C-5’’), 116.5 (C-6’ and C-6’’), 115.7 (C-2’), 115.3 (C-2’’), 115.2 (C-9’ and C-9’’), 74.9 (C-1), 72.8 (C-3), 72.2 (C-5), 70.9 (C-4), 38.0 (C-2), 36.2 (C-6) (Supplementary Figs. S21-S23).
Isochlorogenic acid C (8): HR-ESI-MS (negative) m/z 515.1184 [M-H]–, calcd for C25H23O12 515.1190; 1H NMR (500 MHz, CD3OD): δ7.60 (1H, d, J = 15.8 Hz, H-3’), 7.51 (1H, d, J = 15.8 Hz, H-3’’), 7.01 (2H, d, J = 1.8 Hz, H-9’ and H-9’’), 6.91 (2H, brt, H-5’ and H-5’’), 6.74 (2H, d, J = 1.8 Hz, H-6’ and H-6’’), 6.29 (1H, d, J = 15.8 Hz, H-2’), 6.19 (1H, d, J = 15.8 Hz, H-2’’), 5.62 (1H, m, H-5), 5.12 (1H, dd, J = 9.0, 2.8 Hz, H-4), 4.37 (1H, m, H-3), 2.22-2.05 (4H, m, H-2 and H-6); 13C NMR (125 MHz, CD3OD): δ177.3 (C-7), 168.6 (C-1’), 168.3 (C-1’’), 149.8 (C-7’ and C-7’’), 147.8 (C-3’), 147.7 (C-3’’), 146.9 (C-8’ and C-8’’), 127.8 (C-4’), 127.7 (C-4’’), 123.2 (C-5’ and C-5’’), 116.5 (C-6’ and C-6’’), 115.2 (C-9’ and C-9’’), 114.8 (C-2’ and C-2’’), 76.4 (C-1), 76.0 (C-4), 69.6 (C-3), 69.2 (C-5), 39.6 (C-6), 38.5(C-2) (Supplementary Figs. S24-S26).
The separation of main peaks was carried out by UPLC-ESI-Q-TOF mass spectrometry using a BEH C18 column (I.D 1.7 μm, 2.1 × 100 mm). The injection amount (10 μL) of samples was injected and eluted with the gradient solvent conditions of line A (water in 0.1% formic acid) and line C (ACN) with a flow rate at 0.4 mL/min. The gradient solvent was performed using the following protocol: (0–2 min/90:10, A:C), (2–35 min/90:10, A:C → 0:100, A:C), (35–38 min/0:100, A:C), (38–38.5 min/90:10, A:C), and (38.5–45 min/90:10, A:C). The mass spectrometer was operated in negative mode using the mass ranging from 100 to 2,000 Da, spray voltage at −4,500 V, declustering potential at −80 V, and collision energy at −25.
2,2’-azinobis(3-ethylbenzothiazoline-6-sulfonic acid) (ABTS) and 2,2-diphenyl-1-picrylhydrazyl (DPPH) radical scavenging activities were determined according to slightly modified methods. Simply, ABTS solution (7 mM) including potassium persulfate (2.45 mM) was dissolved in water with dark condition. The sample (10 μL) and ABTS solution (190 μL) were mixed and the absorbance was recorded at 734 nm. The dissolved DPPH solution and the sample were mixed and performed at ultraviolet (UV) absorbance at 517 nm (Kim et al., 2022, 2020).
The total polyphenol content (TPC) in C. soldanella was measured using the Folin-Denis colorimetric method according to some modifications. Simply, samples (20 μL) were mixed with Folin-Denis agent (100 μL) for 1 min at 40°C. The mixtures were contained to a Na2CO3 solution and reacted for 15 min at 40°C. The reaction solutions were performed by the microplate reader using UV absorbance at 765 nm. The total flavonoid content (TFC) was recorded using an aluminum chloride colorimetric assay with slight modifications. The calibration curve was prepared by stock solution (5 mg/mL) and then diluted to concentrations ranging from 31.25−500 μg/mL. Briefly, 50 μL of the diluted samples were separately mixed with 150 μL (MeOH), 10 μL (10% aluminum chloride), 10 μL (1 M potassium acetate), and 280 μL (distilled water). After mixing, the solutions were incubated at room temperature for 30 min and measured at 415 nm (Hassan & Al Aqil, 2013; Sant’Ana et al., 2014).
Intracellular ROS formation was investigated using a 2’-7’-dichlorodihydrofluorescein diacetate (DCFH-DA) assay. Briefly, Raw264.7 cells were cultured in Dulbecco’s Modified Eagle Medium (DMEM). Cells (3 × 105 cells/mL) were conducted with compound 8 for 1 h and then lipopolysaccharide (LPS; 1 μg/mL) was treated each well plate for 24 h at 37°C. Then, the cells were washed and added serum-free DMEM including DCFH-DA (10 μM) in incubation (37°C and 30 min). The mixed solutions were washed and recorded using a flow cytometer (BD Bioscience, San Jose, CA, USA) (Oh et al., 2023).
Results and Discussions
The investigation of anti-oxidative metabolites from 70% EtOH extract of C. soldanella resulted in the isolation of eight compounds (1–8) including three flavonoid derivatives: rutin (4), isoquercertin (5), and nicotiflorin (6) and five quinic acid derivatives: chlorogenic acid (1), crytochlorogenic acid (2), 5-O-(E)-p-coumaroylquinic acid (3), isochlorogenic acid A (7), and isochlorogenic acid C (8), respectively (Fig. 1). The chemical structures were determined by spectroscopic analysis (HR-ESI-MS and NMR spectra) with comparison of reported in the literature (Ganbaatar et al., 2019; Lee et al., 2010; Ma et al., 2022; Pan et al., 2019; Tao et al., 2012).
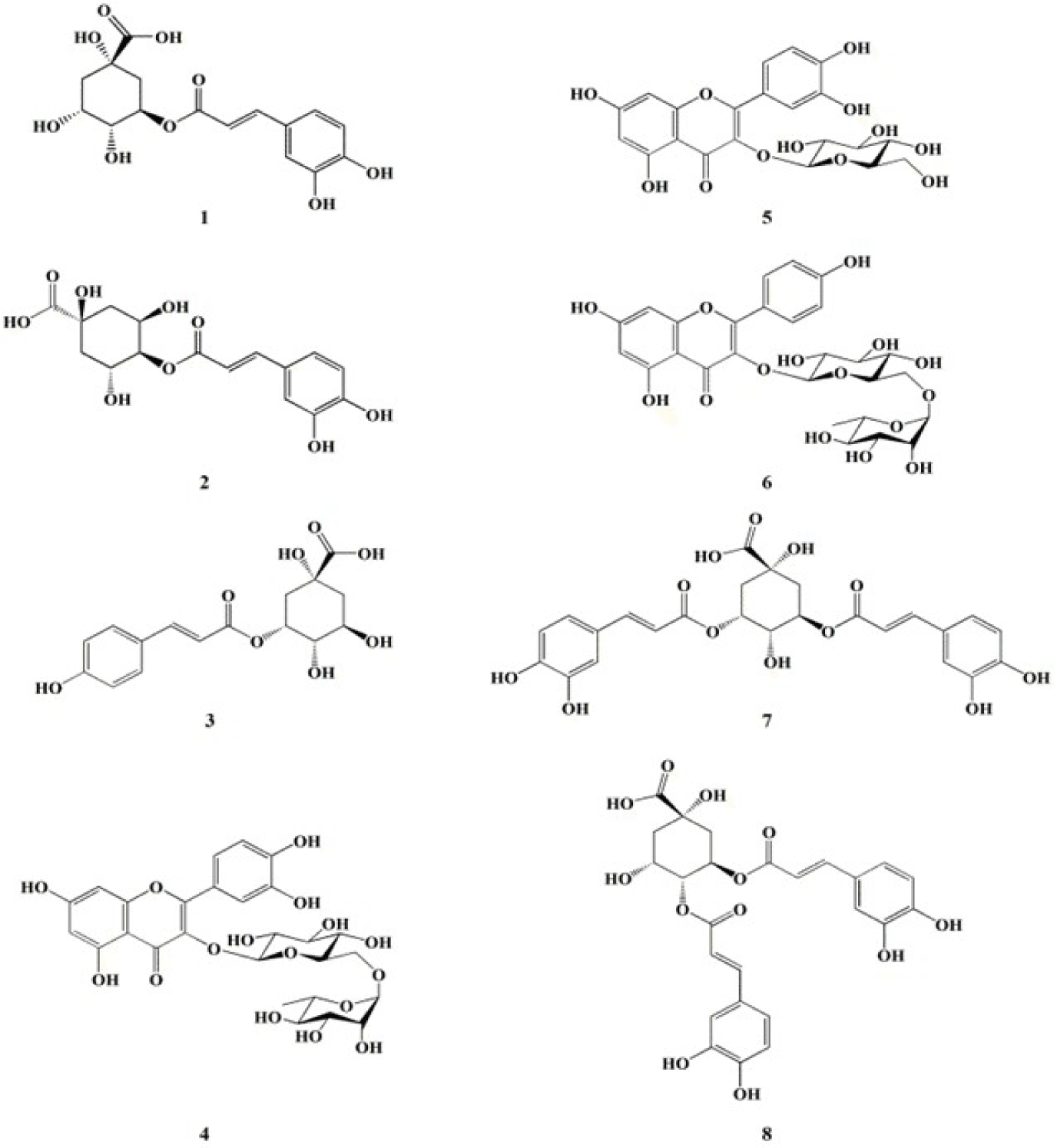
To confirm main components, the 70% EtOH extract (sample concentration: 1 mg/mL) of C. soldanella was analyzed using HR-ESI-Q-TOF mass spectrometry. The peaks (1–8) showed molecular ions based on negative mode of masses consistent with those of isolated compounds (Precursor ions: m/z 1: 353.0878; 2: 353.0880; 3: 337.0924; 4: 609.1449; 5: 463.0873; 6: 593.1501; 7: 515.1188; 8: 515.1184 [M-H]–). The retention time, molecular formula, error, and characterization of peaks (1–8) are shown in (Table 1; Fig. 2).
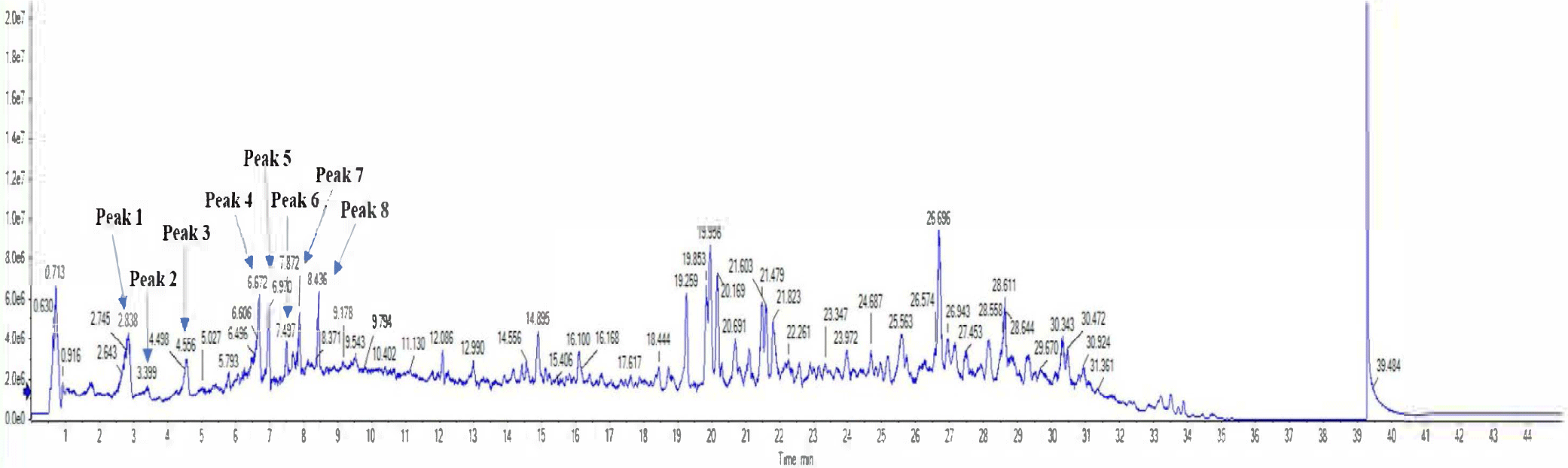
Chemical antioxidant assays (ABTS and DPPH radicals scavenging assays, TPC, and TFC protocols) are broadly used for the primary screening of antioxidant activities. Furthermore, these assays are simple and inexpensive. Polyphenols and flavonoids are divided into various moieties related with attachment of phenol rings. These derivatives exhibit potent antioxidant activities. The results are shown (Table 2). The extract of C. soldanella in TPC and TFC revealed that the values are 13.7 ± 0.9 mg GAE/g and 16.2 ± 0.3 mg QE/g, respectively. Furthermore, the amounts of partitioned portions (n-hexane, EtOAc, and n-BuOH- soluble layers) were varied, ranging from 28.6 ± 0.2 to 30.6 ± 0.5 mg GAE/g and from 44.5 ± 3.1 to 72.4 ± 2.9 mg QE/g in TPC and TFC assays. The remaining water-soluble portion exhibited lower values (8.1 ± 0.2 mg GAE/g in TPC, and 10.3 ± 0.1 mg QE/g in TFC) compared with partitioned portions. In addition, the 70% EtOH extract of C. soldanella were evaluated for its scavenging activity against ABTS and DPPH radicals. The 70% EtOH extract of C. soldanella displayed moderate anti-oxidant activities with IC50 values of 167.5 ± 9.4 (ABTS radical) and 379.8 ± 9.8 μg/mL (DPPH radical), respectively. The most active portion (n-BuOH-soluble layer) in ABTS and DPPH radical scavenging assay showed the IC50 values of 69.1 ± 10.0 and 120.6 ± 4.9 μg/mL, respectively. However, other separated portions (n-Hexane, EtOAc, and water-soluble portions) showed no antioxidant activity in the concentrate with an IC50 value of 150 μg/mL (Table 3). Therefore, the main components of the n-BuOH-soluble layer of C. soldanella were isolated and purified to identify the active anti-oxidative compounds.
To search anti-oxidative compounds from the n-BuOH-soluble portion of C. soldanella, Compounds (1−8) were isolated through purification and isolation, which is followed by column chromatography techniques. The anti-oxidant activities of compounds (1−8) showed IC50 values ranging from 12.4 μM to > 200 μM and 37.9 μM to > 200 μM, respectively. The most active compound 8 (isochlorogenic acid C) exhibited the IC50 values of 12.4 ± 0.1 μM (ABTS radical) and 37.9 ± 0.5 μM (DPPH radical). Compounds 7 and 8 displayed higher activities than ascorbic acid (IC50 values: 24.4 ± 0.4 μM/ABTS radical and 74.4 ± 0.7 μM/DPPH radical). Furthermore, compound 5 (isoquercetin) also showed potent antioxidant activities (ABTS and DPPH radicals) with IC50 values of 27.4 ± 0.6 and 47.0 ± 1.9 μM, successively (Table 3). In particular, we confirmed that two main classes were flavonoid and quinic acid derivatives from the extract of C. soldanella. Increasingly, compound 5 showed higher antioxidant effect than compound 6, indicating that is contributed to the existence of the hydroxyl unit at C ring in the flavone moiety. Furthermore, the attachment of an additional sugar moiety at glucose unit in isoquercetin slightly decreased the antioxidative activity compared with that of compound 5. Therefore, the attachment of the hydroxyl unit at C ring in flavone moiety plays a role for antioxidant activity. In addition, the presence of coumaroyl moiety in compound 3 considerably decreased its antioxidant activity compared with caffeoyl moiety in compounds 1 and 2. Furthermore, the additional attachment of a caffeoyl moiety (compounds 7 and 8) at quinic acid moiety significantly enhance the antioxidant activity than compounds 1 and 2. Therefore, these results considered that the existence of a caffeoyl moiety and the attachment between quinic acid and an additional caffeoyl moiety play important role for the antioxidant activity.
DCFH-DA is one of the most commonly used dyes for the direct detection of intracellular ROS levels. Therefore, we used flow cytometric analysis to measure the effect of compound 8 on the intracellular ROS levels in LPS-stimulated RAW 246.7 cells. As shown in Fig. 3, compound 8 did not show the cytotoxicity at the concentration of 50, 100, and 200 μM. The results displayed that the LPS-treated group shifted rightward compared with LPS non-treated group. However, the compound 8 and ascorbic acid treated groups were shifted at leftward compared with the LPS-treated group. In particular, compound 8 decreased intracellular ROS levels at the concentrate of 50, 100, and 200 μM. Additionally, we presented DCF-DA fluorescence as fold changes in mean fluorescence intensity (MFI) compared to the non-treated group. The compound 8−treated groups significantly decreased MFI compared to the non-treated group, Therefore, compound 8 showed the antioxidant effects against intracellular ROS production.
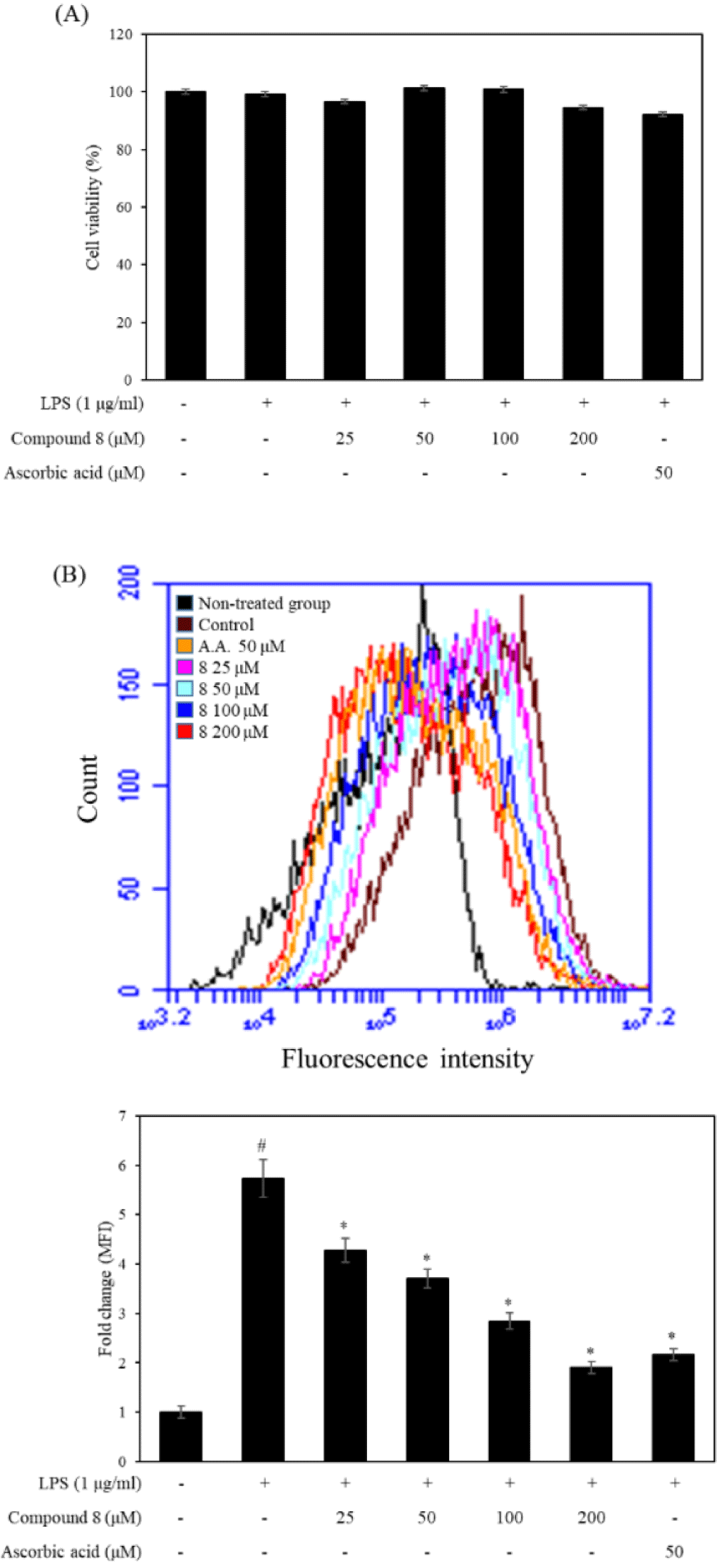
Conclusion
In the current study, we demonstrated the main components with their antioxidative activities from 70% EtOH extract of C. soldanella. We found that the n-BuOH-soluble layer have potent anti-oxidant effects against TPC, TFC, ABT, and DPPH radicals scavenging assays. Compounds (1−8) were acquired from the n-BuOH-soluble layer of C. soldanella through isolation process and identified as chlorogenic acid (1), cryptochlorogenic acid (2), 5-O-(E)-p-coumaroylquinic acid (3), rutin (4), isoquercetin (5), nicotiflorin (6), isochlorogenic acid A (7), and isochlorogenic acid C (8). The isolated compounds (1–8) were detected by HR-ESI-MS spectrum of the 70% EtOH extract of C. soldanella. Furthermore, two main classes including flavonoid derivatives and quinic acid derivatives from the 70% EtOH extracts of C. soldanella were characterized and evaluated for their antioxidant activities. The most active compound 8 exhibited the IC50 values of 12.4 ± 0.1 and 37.9 ± 0.5 μM in the ABTS and DPPH radical scavenging assays. Compound 5 (isoquercetin) also showed strong antioxidant activities with IC50 values of 27.4 ± 0.6 (ABTS radical) and 47.0 ± 1.9 μM (DPPH radical). Furthermore, the presence of a hydroxyl unit at C ring in flavone moiety indicated a role in the structure-activity relationship. The presence of a caffeoyl moiety and the attachment between quinic acid and an additional caffeoyl moiety considerably enhanced the antioxidant activity. We also evaluated for inhibitory activity on intracellular ROS production and confirmed the anti-oxidant effects of compound 8. These results can provide valuable information as natural antioxidants from C. soldanella.
Supplementary materials
Supplementary materials are only available online from: https://doi.org/10.47853/FAS.2024.e61.