Introduction
At present, many aquaculture models are being increasingly developed, including models of intensive shrimp farming in the Mekong Delta. Stocking densities of 50–70 and 80–100 shrimp/m2 are often widely applied in conventional intensive shrimp ponds to raise black tiger shrimp and whiteleg shrimp, respectively, which are two economically important species throughout Asia. According to the report of the Directorate of Fisheries, Viet Nam (Nova Consumer, 2020), the shrimp farming area reached 742,483 ha in 2020, equaling 104.2% compared to 2019. Of this total area, the black tiger shrimp farming area was 629,065 ha, while that of whiteleg shrimp covered 113,418 ha. Harvest output reached 900,000 tons, up 12% over the same period in 2019. Export turnover reached US$ 3.7 billion, up 11% compared to the same period in 2019.
In order to raise shrimp with high productivity, the control of the phytoplankton community in shrimp ponds requires close attention. Algae play an important role in supplying oxygen to shrimp ponds and contributing to forming a “veil” to prevent light from reaching the bottom, which helps reduce stress in shrimp and limits the growth of benthic algae. In addition, algae are the first link in the food chain in the cycle of matter and energy metabolism in water bodies (Ut & Oanh, 2013). Moreover, algae are a rich source of nutrients for shrimp and fish, and they contain high amounts of chlorophyll, beta-carotene, and vitamins (B1, B3, B6, B13, and vitamin E). Guerrero-galván et al. (1998) suggested that the high biomass of algae is controlled by optimal aquatic environmental conditions such as high light intensity and high temperature. Phytoplankton populations also play an important role in nitrogen absorption and prevent nitrogen accumulation to toxic levels in shrimp ponds. Effective uptake of ammonium and nitrate by blooming diatoms and phytoflagellates likely prevented nutrient concentrations from reaching toxic levels and thereby generated an eco-friendly aquaculture water discharge into the adjacent ecosystem (Fernandes et al., 2019). According to Arifin et al. (2017), an increase in phytoplankton productivity is due to an increase in the nutrient content of the cultivation pond. Shrimp feed and waste products are sources of nutrients that support the growth of algae. However, algal overgrowth in shrimp ponds will affect the quality of the aquatic environment, thereby adversely affecting the shrimp. Moreover, the succession of the phytoplankton community during shrimp cultivation follows a process in which phytoplankton succeed from initial establishment driven by abiotic and biotic factors; this process might affect the function of the aquaculture ecosystem and the health of reared shrimp to some extent (Yang et al., 2020). Therefore, this study was conducted to investigate the species composition and density of algae in brackish shrimp ponds as a basis for algal management as well as water quality management, which will contribute to the stable development of shrimp farming in the future.
Materials and Methods
The study was carried out in three black tiger shrimp ponds and three whiteleg shrimp ponds in Bac Lieu province, Vietnam (Fig. 1). Qualitative and quantitative samples of algae were collected once a week for the first month after stocking shrimp. From the second month on, algae samples were collected every 2 weeks until the shrimp were harvested. Black tiger shrimp ponds were sampled a total of 10 times, and whiteleg shrimp ponds were sampled 8 times. Black tiger shrimp and whiteleg shrimp were harvested at 96 days and 70 days, respectively. The sampling cycle is shown in detail in Table 1.
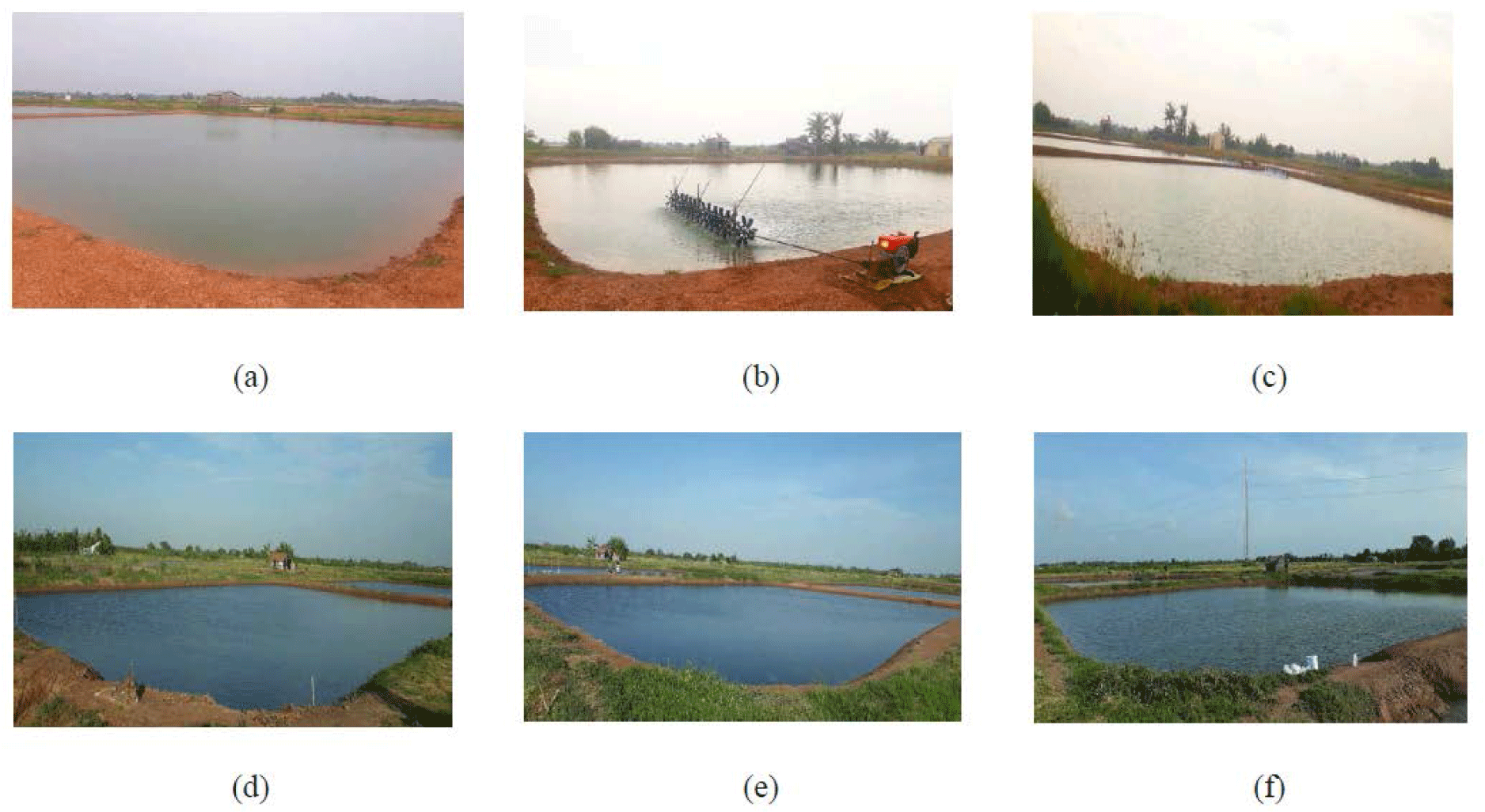
Times | Black tiger shrimp (day) | Whiteleg shrimp (day) |
---|---|---|
1 | 1 | 1 |
2 | 7 | 7 |
3 | 14 | 14 |
4 | 21 | 21 |
5 | 28 | 28 |
6 | 42 | 42 |
7 | 56 | 56 |
8 | 70 | 70 |
9 | 84 | |
10 | 96 |
Black tiger shrimp ponds were stocked at a density of 35 shrimp/m2 at post larvae 15 (average body weight of 0.02 g), and the area of each pond was 1,000 m2. The whiteleg shrimp ponds had an area of 1,200 m2 and were stocked at a density of 60 shrimp/m2 at post larvae 12 (average body weight of 0.013 g). The shrimp ponds were located close to each other and had the same water supply. Water taken from the supply channel was diverted into a settling pond. After treatment, the water was moved into shrimp ponds and was then colored. Prior to stocking shrimp, we used calcium lime (25 kg/1,000 m3) and dolomite lime (25 kg/1,000 m3) for 2 days to help stabilize the water environment and create colored water in the shrimp ponds.
Shrimp were fed Grobest Feed (Vietnam) in the amount recommended by the manufacturer. At the beginning of the study period, after stocking for one week, shrimp were fed at a rate of 10%–12% of their weight; then they were fed at a rate of 7%–9% until the one-month period, and after this period until harvest, shrimp were fed at a ratio of 1%–3%. A mixture of probiotics (Viprotech 0.5 L + CP-Bioplus 0.5 L + sugar molasses 1 L + 50 L pond water) was incubated anaerobically for 24 hours and then applied once a week in the afternoon to stabilize the growth of algae. The culture protocol did not include a change of water until 2 months after the shrimp were stocked; after this time, additional water was provided at a rate of 10%. Water was supplied once a week from the treated settling pond.
The water quality parameters determined in this study, including temperature, pH, and salinity, were measured directly in the shrimp ponds using a Hana HI98128 multi-parameter. Water samples to determine other parameters including total ammonia nitrogen (TAN), nitrate (NO3–), phosphate (PO43–), total nitrogen (TN), total phosphorus (TP), and chlorophyll-a were collected and stored in a 1-L plastic bottle at 4°C. All parameters were analyzed according to APHA (2017). Water quality parameters were collected for a period of 1 day after stocking shrimp, then collected periodically once a week for the first month. From the 2nd month on, these factors were taken every 2 weeks until the end of the farming process.
Qualitative samples of algae were collected by plankton net with a mesh size of 20 µm at different locations in the shrimp ponds. After collecting a sample, it was placed in a 200-mL plastic bottle. Quantitative samples of algae were collected by the sedimentation method using a small plastic cup at many different points in the shrimp ponds and placed into 20-L plastic buckets, stirring the samples. A quantitative sample (1 L) was then taken. Phytoplankton samples were fixed with formalin at a concentration of 2%. The sampling cycle of algae was conducted similarly to that of water parameters.
The species composition of algae was determined based on taxonomic literature to identify the species of algae present in the shrimp ponds. Classification documents used in this study included works by An (1993), Bellinger & Sigee (2015), Omura et al. (2012), Shirota (1966), Tien (1996), Tien & Hanh (1997). Algal abundance was determined using a Sedgwick-Rafter counting chamber according to the method of Boyd & Tucker (1992). Phytoplankton density was calculated using the following formula:
Where X is the density of phytoplankton (ind./L), T is the number of individuals counted, Vcon. is the volume of the concentrated sample (mL), A is the area of one cell (1 mm2), N is the number of cells counted, and Vsam. is the volume of the collected sample (mL).
Where: Pi = ni / N (ni is the individual number of species i, and N is the total amount of algae present in the sample).
The Pearson correlation between water quality parameters and dominant algae species density in shrimp ponds was analyzed using SPSS 22.0 software. Additionally, canonical correlation analysis (CCA) was also used to analyze the relationship between environmental variables and phytoplankton phyla abundance and species composition by R 3.6 and R Studio software.
Results and Discussion
We recorded a total of 75 species of algae belonging to 5 phyla in black tiger shrimp ponds; of these, diatoms had the highest species number (29 species, 39%), followed by green algae (19 species, 22%). The remaining algal phyla, including blue-green algae, euglenoids, and dinoflagellates, ranged from 7–12 species (9%–16%) (Fig. 2). In whiteleg shrimp ponds, 64 species of phytoplankton were identified, including diatoms (30 species, 47%), dinoflagellates (11 species, 17%), green algae (9 species, 14%), blue-green algae (9 species, 14%), and euglenoids (5 species, 8%) (Fig. 3). The difference in algal species composition between black tiger shrimp and whiteleg shrimp ponds was due to the difference in salinity, which fluctuated from 4–6 ppt in black tiger shrimp ponds, and from 8–12 ppt in vannamei ponds. According to Lien et al. (2022), the average density of Cylindrotheca closterium decreased significantly (about 56 times) when the salinity increased in the culture period. Similarly, other algae species such as Nitzschia sp., Amphiprora alata, and Pleurosigma sp. also tended to be negatively correlated (p < 0.05) with salinity in super-intensive whiteleg shrimp ponds. In this study, freshwater algal phyla present in black tiger shrimp ponds, such as green algae and blue-green algae, had a higher species number than in whiteleg shrimp ponds. Some algal genera often appear in both black tiger shrimp and whiteleg shrimp ponds, such as Gyrosigma, Campylodiscus, and Cyclotella (Bacillariophyta). According to Oanh et al. (2014), in a study on the relationship between shrimp health and phytoplankton population fluctuations in intensive whiteleg shrimp ponds, the algal composition was much higher than in our study. Oanh et al. (2014) found 119 algae species in total in whiteleg shrimp ponds in Soc Trang and Ca Mau provinces, Vietnam. In contrast, Shaari et al. (2011) revealed that a total of 19 microalgae species were found in the black tiger shrimp pond in Pulau Pinnang, Malaysia, with diatoms contributing up to 72% of the species, followed by Chlorophyta (11%) and Cyanophyta (11%).
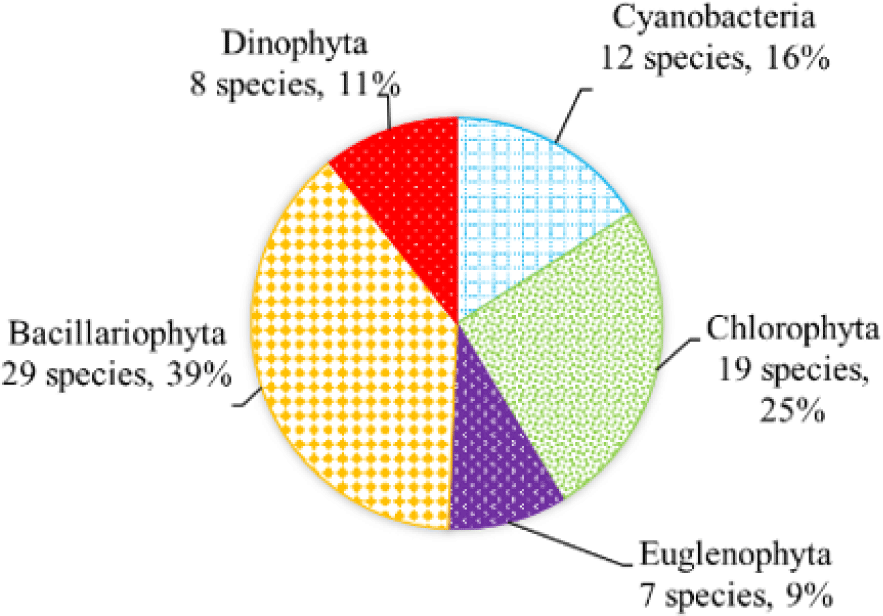
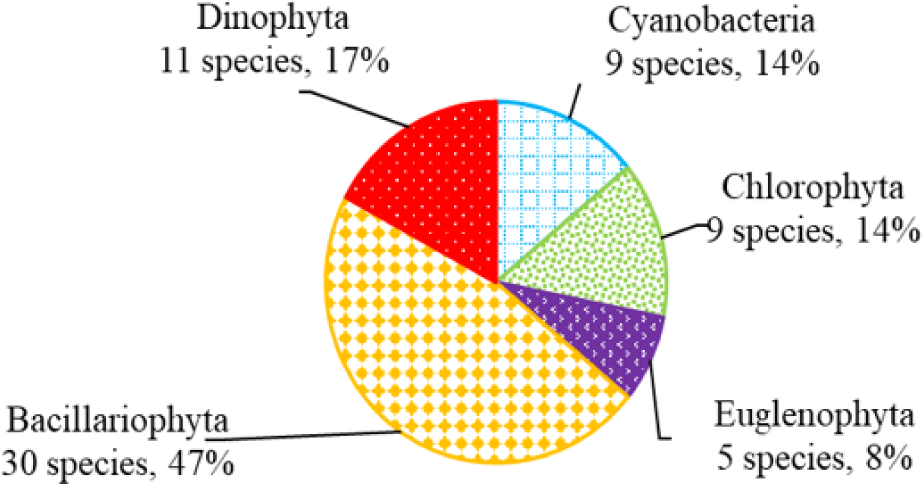
We identified 18 species that were present in all black tiger shrimp and whiteleg shrimp ponds, including Campylodiscus encheneis, Gyrosigma acuminatum, Tropidoneis sp., Synedra ulna, Thalassiosira sp., Nitzschia sigma var. intercendens, Synedra sp., Cyclotella striata, Chaetoceros contortus, Campylodiscus daemelianus (Bacillariophyta), Oscillatoria limosa, Phormidium sp., Microcystis aeruginosa, Microcystis smithii (Cyanobacteria), Euglena sp. (Euglenophyta), Peridinium sp., Alexandrium sp., Pyrocystis robusta (Dinophyta). In addition, 57 species only appeared in black tiger shrimp ponds, and 46 species were only identified in whiteleg shrimp ponds (Fig. 4). Some common species found in brackish shrimp ponds included O. limosa, M. aeruginosa (Cyanobacteria); Nannochloropsis sp. (Chlorophyta); Euglena gracilis (Euglenophyta); Thalassiosira sp., C. contortus (Bacillariophyta); Alexandrium sp., and Peridinium sp. (Dinophyta).
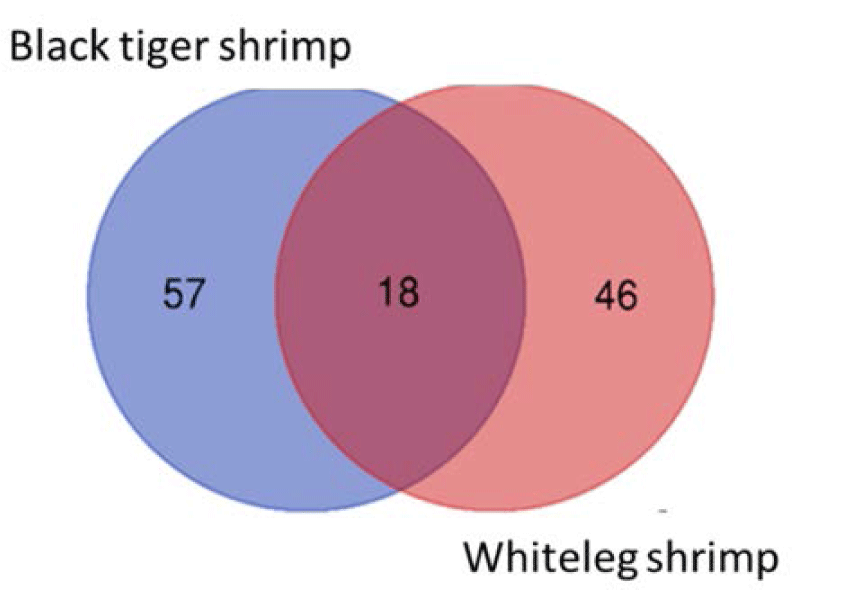
The number of algal species in the black tiger shrimp and whiteleg shrimp ponds varied relatively widely, ranging from 34 to 50 species (Fig. 5). For black tiger shrimp ponds, species composition was highest at the beginning of the shrimp crop (before 21 days), ranging from 41 to 50 species. Diatoms and green algae had high species numbers, varying from 14–23 and 12–14, respectively. Algal communities tended to decrease in the period from 28–70 days. At these stages, the species numbers of diatoms and green algae were still quite high, ranging from 11–13 and 6–9, respectively. The algal species composition at the end of the cultivation crop (84–96 days) tended to be higher than in the middle of the culture cycle, ranging from 39–42 species. In general, cyanobacteria and diatoms had an increased species composition at the end of the crop, while green algae and euglenoids tended to decrease in this phase.
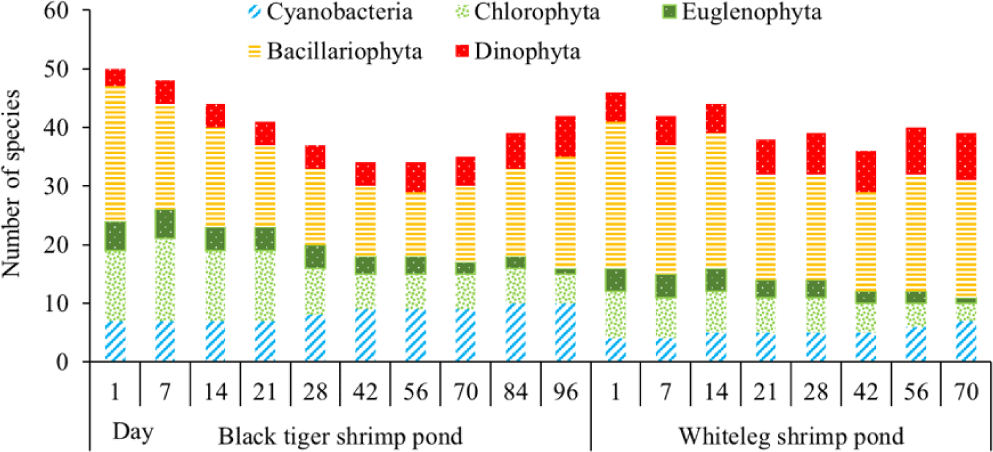
In the whiteleg shrimp ponds, the algal composition did not change much over the sampling periods, ranging from 36–46 species. The change in algal composition over time was similar to that in black tiger shrimp ponds. In the first phase of the farming crop (before 14 days), the algal species composition was very high, ranging from 42 to 46 species. Diatoms still accounted for the highest proportion. The total number of algal species at 21–56 days tended to decrease slightly, ranging from 36–40 species. Blue-green algae and dinoflagellates showed a slight increase in species composition at the end of the culture crop. In contrast, the species composition of euglenoids and green algae decreased at the end of the crop cycle (Fig. 5).
Diatoms had a higher species composition than other phyla due to their ability to survive in both freshwater and brackish-/saltwater environments. They had a more diverse species composition in the brackish-/saltwater environment. The aquatic environment in intensive shrimp culture ponds with fewer changes of water contained a high density of diatoms. Some algal species commonly appear in brackish water bodies, including Thalassionema, Cyclotella, and Campylodiscus (Ryther & Officer, 1981), and are used as a food source for consumers at higher trophic levels (Boyd, 1990).
In this study, the composition of green algae was also relatively high due to the fact that, at the beginning of the growing season, the water environment had relatively low salinity, so it was suitable for their growth. Freshwater algae tend to gradually disappear as salinity increases. In addition, the algal species composition varied with salinity changes because most algae are stenohaline and are shocked by osmotic pressure regulation (Bisson & Kirst, 1995). Euglenoids had a relatively low species composition, ranging from 5–7 species belonging to genera like Euglena, Phacus, and Trachelomonas. Blue-green algae were present at most of the sampling stages, but the species composition was less diverse because they are often distributed mainly in freshwater bodies (Tuyen, 2003). For shrimp farmers, blue-green algae are considered a harmful algal group. Therefore, during most of the pond-preparation stages, farmers usually find many ways to limit the growth of blue-green algae in shrimp ponds. According to Thanh (2022), blue-green algae appeared in high density in whiteleg shrimp ponds from the middle to the end of the culture cycle. The composition of the dinoflagellate species in shrimp ponds was quite low and occurred with a low frequency; they included Alexandrium sp., Peridinium sp., Gymnodinium sp. and Ceratium intermedium. These species belong to a group of algae that are widely distributed in freshwater, brackish, and saltwater environments. During the sampling periods, the salinity in shrimp ponds in this study was below 15 ppt, which is considered suitable for the growth and development of the above species.
Algal density in brackish shrimp ponds fluctuated quite strongly, ranging from 497,091–2,237,758 ind./L, with an average density of 1,530,605 ± 570,730 ind./L (Fig. 6). Mean densities of blue-green algae, green algae, euglenoids, diatoms, and dinoflagellates varied from 77,077–731,556 ind./L, 70,043–1,478,083 ind./L, 13,089–91,083 ind./L, 94.815–1,397,487 ind./L, and 62,652–609,993 ind./L, respectively. The cyanobacterial density tended to increase at the end of the crop cycle, while the green algae and euglenoids reached high values at the beginning of the culture process in both black tiger shrimp and whiteleg shrimp ponds. Particularly, diatoms in black tiger shrimp ponds increased at the end of the crop. In contrast, in vannamei ponds, the density of diatoms was the highest at the beginning of the study. The dinoflagellate abundance reached the highest level at 28 days in black tiger shrimp ponds, while in vannamei ponds, their density increased at the end of the crop (Figs. 7–11).
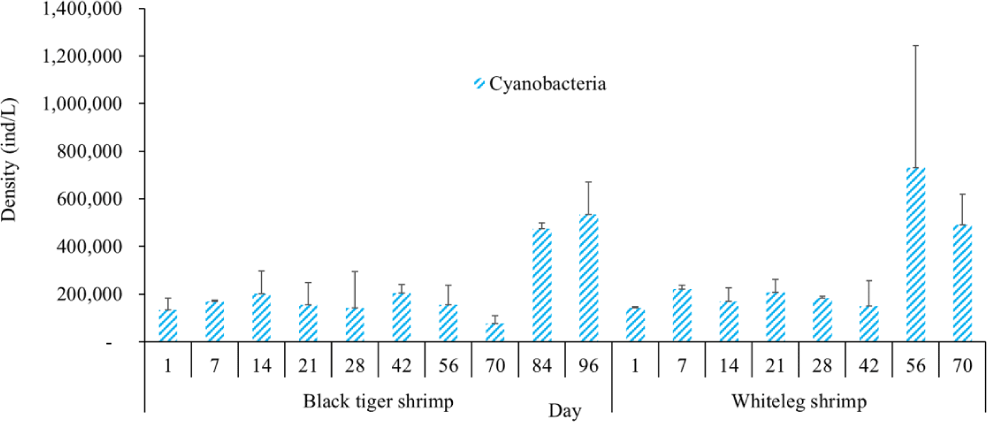
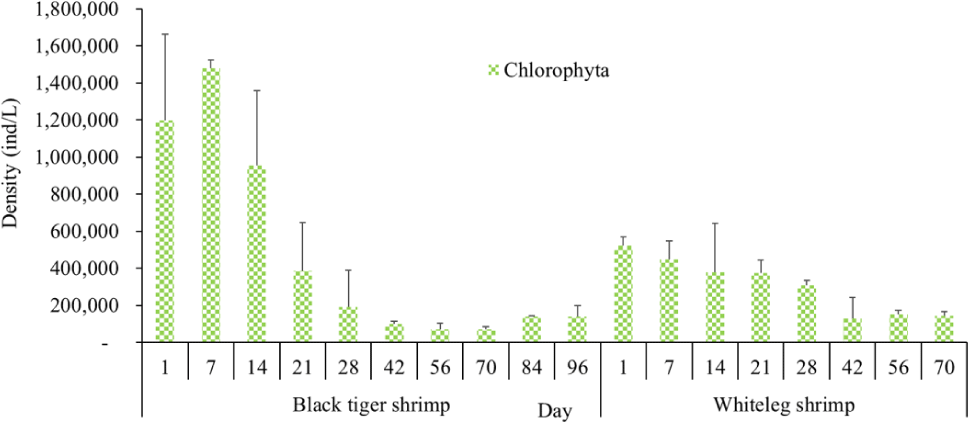
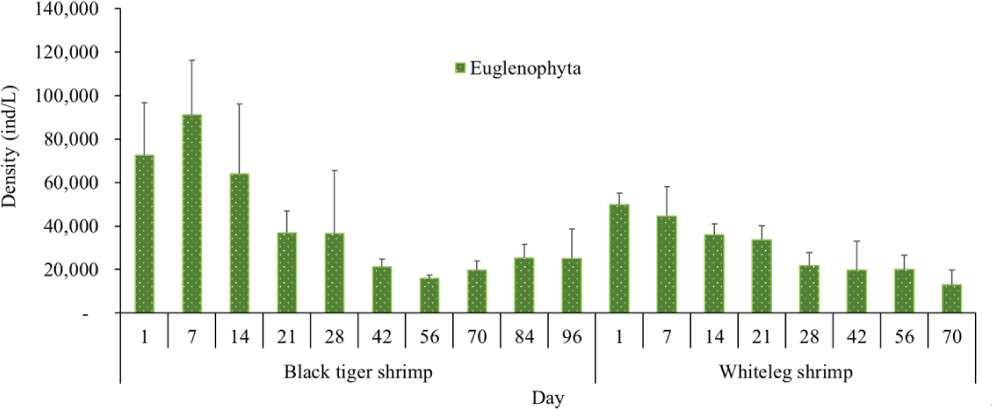
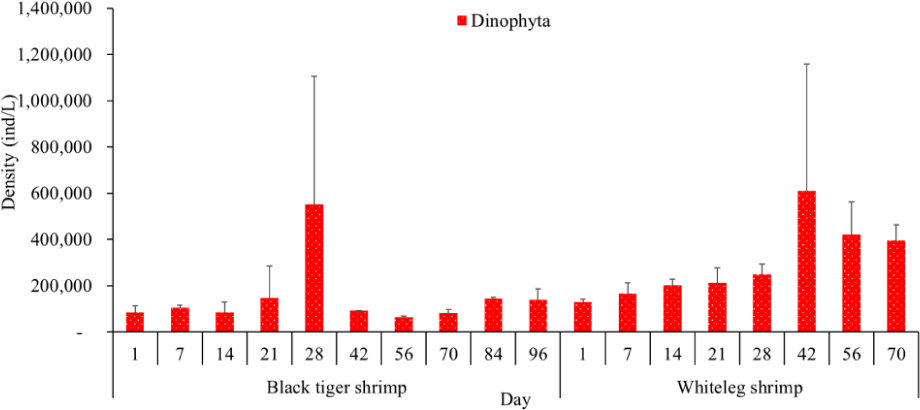
For black tiger shrimp ponds, the algal density was highest at 1–14 days, ranging from 1,580,467–2,229,500 ind./L, with an average density of 655.8511 ± 172.993 ind./L. At this stage, the water had a salinity of 4 ppt, with a predominance of Nannochloropsis algae. Species in this genus are spherical to ovoid in shape, with a very small size (2–4 µm). These algae are able to adapt to wide temperature fluctuations and grow well at 20°C–30°C (Hoff & Snell, 1999). In addition, the chlorophycean bloom was made up mostly of Nannochloropsis sp. in commercial shrimp ponds using green-water technology (Cremen et al., 2007).
Algal density decreased sharply in the period from 21–70 days, ranging from 497,091–1,122,588 ind./L, with an average density of 16,626 ± 952.344 ind./L. Nannochloropsis sp. was still growing with the highest density at 21 days. According to Hoff & Snell (1999), Nannochloropsis sp. can survive in a wide range of salinities from 0–36 ppt. Thus, in the present study, with salinities from 4–6 ppt, it was able grow well. In the 28-day period, the algal density increased with the predominance of Alexandrium sp., coinciding with the high levels of NO3– (0.1 mg/L) and PO43– (0.09 mg/L) in the shrimp ponds, which created favorable conditions for the development of dinoflagellates. The density of Alexandrium algae, which are also harmful and can affect shrimp growth, is often high in the dry season (Thang, 2007). Shrimp development can be negatively influenced even at very low cell densities of Alexandrium. At the end of the crop, algal density tended to increase, ranging from 1,913,808 to 1,938,530 ind./L, with an average density of 11,276 ± 151,398 ind./L. At this time, G. acuminatum and M. aeruginosa had a higher abundance than other algae because of the high nutrient content at the end of the crop cycle. The average TN and TP contents in these stages varied from 1.91–2.43 mg/L and 1.46–1.62 mg/L, respectively. In intensive shrimp ponds, sources of nutrients for phytoplankton growth could be metabolic waste and uneaten feed (Keawtawee et al., 2012). Many species of algae can produce toxins that adversely affect shrimp growth and lead to economic losses, including Microcystis. In summary, green algae (mainly Nannochloropsis sp.) predominated in the early stages after shrimp stocking, while cyanobacteria and diatoms increased in density towards the end of the culture cycle (Figs. 7 and 8, Fig. 10). This result is similar to that of Cremen et al. (2007), who studied changes in species composition and density of algae in commercial shrimp ponds in a tropical region.
For whiteleg shrimp ponds, the algal density was quite high at the beginning of the culture cycle, ranging from 1,788,905 to 2,237,758 ind./L, with an average density of 126,034 ± 219,916 ind./L. At these stages, diatoms were most abundant, with a predominance of the algae Chaetoceros and Thalassiosira (Bacillariophyta), followed by the green algae Nannochloropsis (Chlorophyta). Research by Lien et al. (2022) showed that most sample stages in super-intensive whiteleg shrimp ponds were dominated by algae Thalassiosira sp., with densities ranging from 280,125 to 7,693,417 ind./L, whereas algae Chaetoceros sp. was only observed during commercial shrimp farming times, with densities varying from 1,944 to 15,083 ind./L. In addition, Thalassiosira play an important role in aquaculture, as they are highly nutritious and suitable algae for rearing of shrimp larvae (Tam et al., 2020). The genera Thalassiosira, Chaetoceros, and Nannochloropsis are raised as natural food for rotifers, bivalve larvae, and shrimp larvae.
Algal density tended to decline remarkably in the period from 21–28 days, ranging from 1,401,134–1,538,542 ind./L, with an average density of 218,375 ± 141,249 ind./L. Two algal species, including G. acuminatum, and Nannochloropsis sp., also had a high density at this stage. Microalgae of the genus Nannochloropsis are already exploited in aquaculture for their high content of polyunsaturated fatty acids, carotenoids, polyphenols, and vitamins. These molecules can contribute to delaying aging processes and preventing the onset of many metabolic disorders triggered by unhealthy lifestyles and diets with an excess of saturated fatty acids (Zanella & Vianello, 2020). Additionally, according to research by Yusoff et al. (2002), diatoms often dominate in low-nutrient conditions.
The amount of algae tended to increase from the middle to the end of the farming cycle (42–70 days), varying from 1,555,786 to 1,924,056 ind./L, with a mean abundance of 293,715 ± 959,969 ind./L (Fig. 6). Especially at these stages, there was an increase in the density of cyanobacteria and dinoflagellates, with the predominance of M. aeruginosa (Cyanobacteria) and Alexandrium sp. (Dinophyta). Some species of the genus Alexandrium can produce paralytic toxins (Cembella et al., 1987), while some other species of Alexandrium, in addition to producing paralytic toxins, also produce other toxins with hemolytic activities (Simonsen et al., 1995) that can kill fish (Mortensen, 1985; Ogata & Kodama, 1986). When suitable environmental conditions exist, such as high temperatures, strong light, and high nutrient content, Microcystis will grow at high densities. Hoogenhout & Amesz (1965) reported that the growth rate of blue-green algae is always slower than that of other groups of algae, so Cyanobacteria usually predominate at the end of the culture cycle. Cyanobacteria were significantly higher in control compared to treated ponds during the final phase of the shrimp culture cycle (Yusoff et al., 2002).
Algal density showed less change over the sampling period in vannamei ponds than in black tiger shrimp ponds. In the whiteleg ponds, green algae and diatoms usually predominated at the beginning of the crop, while dinophytes and cyanobacteria had high densities at the end of the farming cycle. Euglenophyta appeared frequently during the study phases, but at a low density. The common genera that appeared in the shrimp ponds were Trachelomonas, Euglena, and Phacus, Trachelomonas species were found at low densities because these algae are adapted to freshwater environments. Research by Tien et al. (2021) identified 25 species of Euglenophyta belonging to 3 genera, including Trachelomonas, Euglena, and Phacus, in brackish-water shrimp ponds in An Bien district, Kien Giang province, Vietnam. Many species of Euglenophyta belonging to the genus Euglena, which are indicator organisms for organic pollution in shrimp-farming ecosystems, were also identified in this study.
The Shannon-Wiener diversity index, Pielou’s evenness index, and the Simpson dominance index in black tiger shrimp and whiteleg shrimp ponds varied from 1.98–2.75 and 2.62–2.91; 0.59–0.81 and 0.09–0.14; and 0.12–0.27 and 0.76–0.86, respectively (Figs. 12 and 13). The results of these indices showed that the composition of phytoplankton species in shrimp ponds was quite diverse. The H’ index in black tiger shrimp ponds decreased at 28 days and at the end of the growing cycle. For vannamei ponds, the diversity of algal species increased at 21 days and at the end of farming cycle. The J’ index was higher in black tiger shrimp ponds than in vannamei ponds, indicating a more even distribution of algal density in black tiger shrimp ponds. These differences can be explained by the fact that in whiteleg shrimp ponds, there were many algal species with high abundance. Similarly, the Simpson dominance index was lower in black tiger shrimp ponds than in whiteleg shrimp ponds. The D index had higher values, which correspond to lower diversity (Gerami et al., 2016). This result once again confirmed that the diversity of algae was lower in the vannamei ponds than in the black tiger shrimp ponds. In addition, according to Marques et al. (2009), the water quality in the brackish shrimp ponds in this study is mostly classified as poor to moderate.
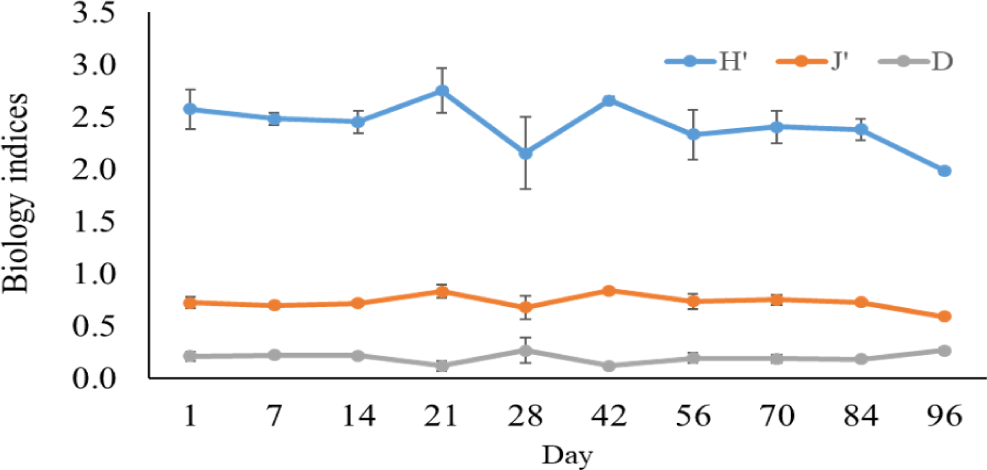
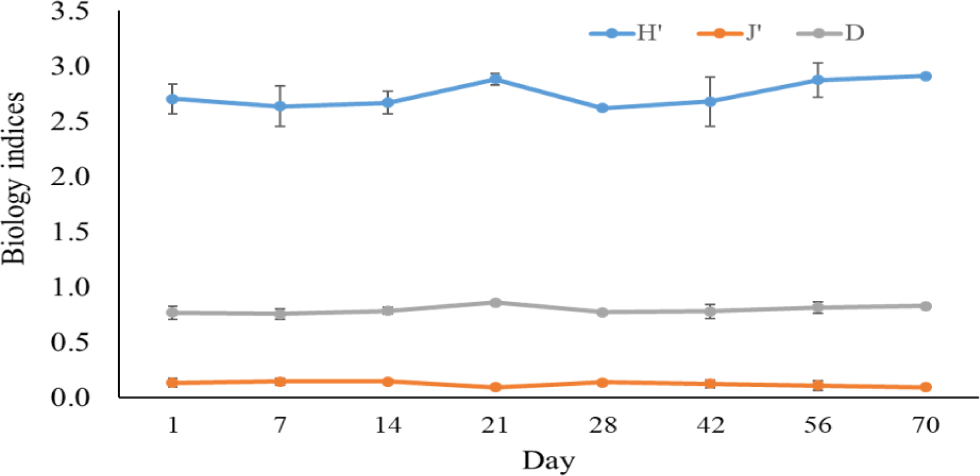
Correlations between environmental factors and dominant algae species density in brackish shrimp ponds are presented in Table 2. In general, temperature had a significant positive correlation (p < 0.01) with the density of A. granulata. The pH value was negatively correlated (p < 0 .01) with the density of Nannochloropsis sp., but positively correlated (p < 0.05) with the abundance of G. acuminatum. In addition, salinity had a significantly positive correlation (p < 0.05) with the dominance of M. aeruginosa, Thalassiosira sp., and C. contortus. However, salinity was negatively correlated with the density of Nannochloropsis sp. Salinity is an environmental factor affecting the species composition as well as the abundance of phytoplankton in aquatic ecosystems. When salinity changes, the cell volume and ability of microalgae to regulate osmotic pressure will also change. Additionally, microalgal species are often adapted to a wide range of chemical parameters, but the most important elements are nitrogen and phosphorus, which are essential for algal reproduction and growth (Cremen et al., 2007). In this study, the nutrient contents in water, including TAN, NO3–, and PO43–, had a significantly positive correlation (p < 0.05) with the blue-green alga M. aeruginosa. The TN content was positively correlated with C. contortus, and TP was negatively correlated (p < 0.05) with Nannochloropsis sp. In addition, G. acuminatum was strongly correlated (p < 0.01) with TAN concentration. Alexandrium sp. had no close relationship (p > 0.05) with most of the water quality parameters in this study.
The multivariable relationship between water quality parameters and species composition and density of algal phyla provided by CCA plot (Fig. 14). The density of diatoms was positively correlated with pH, TAN, and TN concentrations. With higher temperatures, the chlorophyll-a content increased. In addition, the species composition of Cyanobacteria, Bacillariophyta, and Dinophyta, as well as the densities of Dinophyta and Cyanobacteria, were affected by the concentrations of NO3–, PO43–, TP, and salinity. Thus, the diversity and abundance of microalgae often fluctuated quite strongly and depended on the water quality parameters of the shrimp ponds, such as temperature, pH, salinity, and the availability of nutrients. Similarly, according to Shaari et al. (2011), environmental factors such as nutrient levels are able to affect the abundance of phytoplankton during shrimp cultivation.
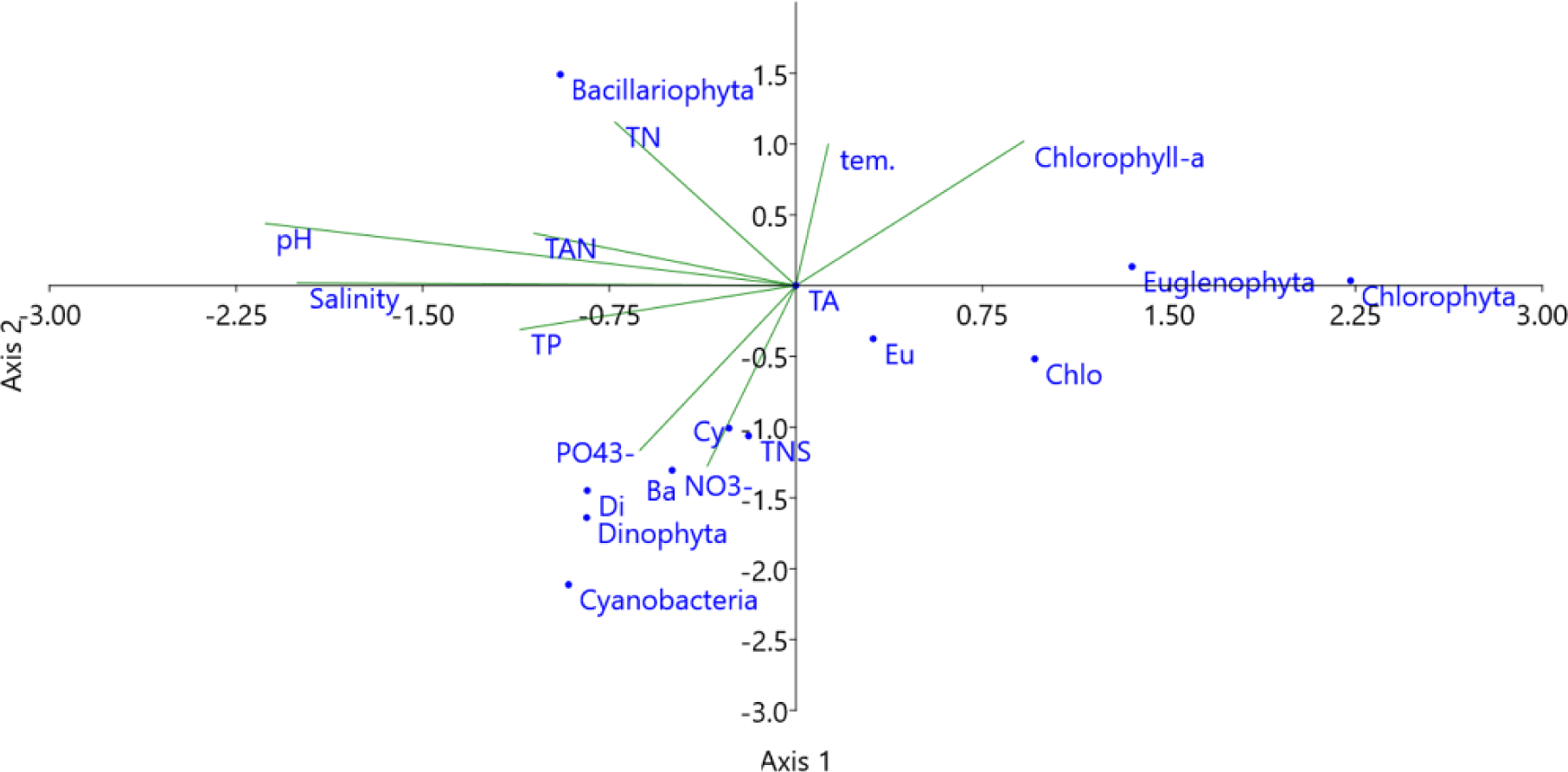
The average shrimp yield in black tiger shrimp and whiteleg shrimp ponds was 2,153 ± 211 kg/ha and 2,694 ± 188 kg/ha, respectively (Table 3). In general, euglenoids had lower densities in whiteleg ponds than in the black tiger shrimp ponds; in contrast, diatoms grew at higher densities during the study stages in whiteleg ponds, contributing to the increase in shrimp productivity.
No. | Pond | Stocking density (ind.m–2) | Mean production (kg/ha) |
---|---|---|---|
1 | Black tiger shrimp | 35 | 2,153 ± 211 |
2 | Whiteleg shrimp | 60 | 2,694 ± 188 |
In conclusion, we recorded five phyla of algae in brackish shrimp ponds. Diatoms had the richest species composition with 29–30 species, followed by green algae with 9–19 species. The remaining phyla, i.e., blue-green algae, euglenoids, and dinoflagellates, had 7–12 species. Diatoms and green algae reached high densities at the beginning stages of the farming cycle, while cyanobacteria increased in abundance towards the end of the cycle, with a predominance of diatoms and dinoflagellates. An increase in the density of cyanobacteria and dinoflagellates at the end of the growing season can significantly affect shrimp growth and yield. The concentrations of PO43– and TP should be limited in order to minimize growth in both species composition and density of cyanobacteria and dinoflagellates and to promote the development of beneficial algae in shrimp ponds. Additionally, the water exchange for shrimp ponds should be done regularly, especially at the end of the farming process, to reduce blue-green algae and dinoflagellate development. These findings contribute greatly to the management of the aquatic environment, especially the management of algae, in brackish-water shrimp ponds. The relationship between pond management and its impact on algal and water quality fluctuations in shrimp farming processes should be studied further.