Introduction
Vibrio parahaemolyticus is a common bacterium in marine ecosystems and in almost aquaculture ponds of Southeast Asian countries (FAO & WHO, 2020). Records of gastroenteritis in humans caused by V. parahaemolyticus date back to 1950, with 20 deaths in the 272 cases when humans consumed seafood in raw or partially-cooked states (Fujino et al., 1953). V. parahaemolyticus can survive in contaminated seafood and has caused outbreaks of infections in many Asian countries, including China, India, Japan, Thailand, and Vietnam; recently, infections have been reported from the USA (Meparambu Prabhakaran, 2020; Su & Liu, 2007; Vugia et al., 2009; Xu, 2015). Besides, V. parahaemolyticus is one of the main causative agents of acute hepatopancreatic necrosis disease (AHPND) in black tiger shrimp, cultured in many countries around the world (Nghia et al., 2015; Tran et al., 2013).
Haemolysin is an extracellular toxin most widely distributed among pathogenic Vibrio species (Shinoda, 1999). As a distinct gene, the thermolabile haemolysin (tlh) gene (Vptlh) has been reported in most isolated V. parahaemolyticus specimens from clinical and environmental sources (Bej et al., 1999; Mahmuda et al., 2006; Shinoda, 1991; Yamazaki et al., 2008). A specific primer pair, with a forward primer from base 904 to 927, has frequently been applied to detect Vptlh in shellfish or seafood. Their amplified product is a nucleotide sequence of 450 bp, found in 111 tested V. parahaemolyticus strains (Bej et al., 1999; Yáñez et al., 2015). However, these studies do not mention the correlation between this fragment and the Kanagawa phenomenon on Wagatsuma blood agar.
The Vptlh is the nucleotide sequence of 1.5 kb in its full length and encodes an entire protein called Vptlh, with a molecular weight of 47.5 kDa (Taniguchi et al., 1986), cause the haemolytic activity on blood agar. However, another study has reported that the Vptlh is present in two mutant cases of oligonucleotide deletions in the length of the Vptlh, one deleted bases from 1,007 to 1,586 and another from 642 and 1,586, have not produced tlh in Escherichia coli cells (Taniguchi et al., 1986). Besides, two recombinant Vptlhs in full length have been produced in recent years, however both cases have expressed in the forms of an inclusion body. Both above-mentioned studies have suggested that the refolded Vptlh is a lecithin-dependent haemolysin (ldh), and its haemolytic activity requires two substrates of phospholipase enzyme, such as phosphatidylcholine or lecithin, in the assays (Vazquez-Morado, 2021; Zhao et al., 2011). This has led to the hypothesis that the haemolytic action is dependent on the action of phospholipase. Specifically, the known overall tlh structure comprises N-terminal and C-terminal domains. However, there is no explanation about what domain responds to the haemolytic or phospholipase activities (Guerrero et al., 2017).
The Vptlh toxin is a thermolabile toxin (Shinoda, 1991; Yanagase et al., 1970). There is evidence that the haemolytic action of Vptlh is dependent on the activity of the phospholipase enzyme (Vazquez-Morado, 2021; Wang et al., 2012, Wang et al., 2015). Recent studies have discovered new compounds such as the flavonoid quercetin, the epigallocatechin gallate, and morin as alternatives to inhibit the phospholipase enzyme of Vptlh. The inhibition parameters of these compounds record respective half-maximal inhibitory concentration values of 4.5, 6.3, and 9.9 μM (Vazquez-Morado, 2021). In addition, the inhibition of metal cations to the haemolytic action to tlh of Vibrio alginolyticus (Vatlh) has been recorded. That is, the haemolytic activity of Vatlh decreased to 50% in the presence of Ca2+, Zn2+, and Mn2+ at concentrations < 1 mM (Jia et al., 2010).
A recent review has a summary and index of many chemical constituents of the Phellinus genus that have exhibited biological activity (Pingya, 2021). In the extract of this genus, various bioactive compounds have potent pharmacological properties, including preventing infection and enhancing immunity (Yang et al., 2009). Their cytotoxicity against several tumor cell lines, and some tested compounds demonstrated weak cytotoxicity (Thanh et al., 2018).
As previously mentioned, Vptlh is a refolded recombinant protein. A soluble protein is a vital prerequisite for assessing biological activity. Therefore, to prevent the loss of biological activity and examine the domain’s responsibility in the haemolytic activity of the Vptlh, we directly amplified a 450-bp VpTLH nucleotide sequence of the entire Vptlh gene encoded the C-terminal domain from a pathogenic strain of V. parahaemolyticus with a particular primer pair (Bej et al., 1999). The VpTLH product was isolated and then cloned in an expression plasmid as pET11-His-tag. Afterward, the VpC-terminal domain fused with a 6-His-tag named the His-tag-VpC-terminal domain of 47 kDa was purified successfully by affinity chromatography. The high purity was a significant result for understanding and determining the responsibility of the His-tag-VpC-terminal domain.
Materials and Methods
We used the bacterium V. parahaemolyticus causing AHPND, provided by the Research Institute for Aquaculture No. 2 (RIA2). The strain was grown in a medium supplemented with a maximum salt content of 1%–2% in thiosulfate-citrate-bile salt-sucrose medium (HiMedia, Thane, Indian). The expression vector pET-11a (with a N-terminal His-Tag) was used for overexpression of the recombinant protein, the E. coli strain NEB turbo and E. coli BL21 pLysS (DE3) cells were host cells for cloning and expression of the recombinant protein. Luria-Bertani (LB) medium (HiMedia) was used for routine culture of E. coli at 37°C. On LB agar with ampicillin antibiotic (LB/Amp, 100 µg/mL), the E. coli transformants with recombinant pET-11a-His-Tag plasmids were kept at –70°C.
A 450-bp (VpTLH) nucleotide sequence encoding the C-terminal domain of the Vptlh directly amplified from the genomic DNA of V. parahaemolyticus as a template, combined with a specific primer for polymerase chain reaction (PCR) amplification. The forward primer was designed upon bases from 904–927 of the Vptlh gene (accession number M36437.1) (Bej et al., 1999; McCarthy et al., 1999); the sequence of the forward primer was tlh-F: 5’-GGG GCA TAT GAA AGC GGA TTA TGC AGA AGC ACT G-3’ and that of the reverse primer was tlh-R: 5’-GGG GGG ATCCGC TAC TTT CTA GCA TTT TCT CTG C-3’. In these primers, the restriction sites of NdeI and BamHI enzymes are shown in bold and were synthesised by integrated DNA technology (Bej et al., 1999). For cloning, two restriction enzymes, NdeI and BamHI (Thermo Scientific, Waltham, MA, USA), were used to double-cleave both the VpTLH and an expression vector pET-11a-His-tag (Novagen, Gyeryong, Korea) before both were ligated together to create a recombinant plasmid harbouring the VpTLH by T4 ligase (Thermo Scientific). The recombinant plasmid (pET11a-His-tag-VpTLH) was transformed into competent E. coli NEB turbo by heat shock at 42°C, and the bacterial cells were plated on LB/Amp agar at 37°C overnight for screening and selecting the recombinant plasmid. From a single recombinant E. coli NEB turbo colony was chosen for continuous culturing in LB agar with 50 µg ampicillin, and the pET11a-Histag-VpTLH plasmid was extracted using an extraction kit (Monarch Plasmid Miniprep Kit, NEB). The cloned VpTLH sequence in the pET-11a-His-tag was confirmed by DNA sequencing and retransformed into competent E. coli BL21 pLysS (DE3) cells by the same method.
For expression a His-tag-VpC-terminal domain, first the E. coli BL21 (DE3) pLysS cells containing pET11a-Histag-VpTLH were grown at 37°C until cell density approached an optical density at 600 nm (OD600) of 0.6 in LB medium containing 100 μg/mL ampicillin. An expression survey of the His-tag-VpC-terminal domain was performed by inductions with various isopropyl-β-D-thiogalactopyranoside (IPTG) concentrations (0.5, 1.0, 1.5, 2.0 mM). Cells were grown continuously for an additional 20 hours at 37°C before they were harvested by centrifugation. The expressed His-tag-VpC-terminal domain was visualised by sodium dodecyl sulfate polyacrylamide gel electrophoresis (SDS-PAGE) using 12% (vol/vol) polyacrylamide gels, and the Bradford assay was used to determine the protein concentration. For purification, the induced cells on 100 mL LB medium with 100 μg/mL ampicillin were collected by centrifugation at 4,032×g for 30 minutes at room temperature. The pellet was resuspended in 5 mL lysis buffer containing 3–5 mM imidazole, followed by ultrasonication and centrifugation at 18,928×g for 30 minutes at 4°C to harvest the total lysate cell extract. Before purification, a volume buffer of 6 mL, containing 10 mM imidazole, was loaded into the His-pureTM nickel-nitrilotriacetic acid (Ni-NTA) spin column containing a 3 mL resin bed (Thermo Scientific), and the bottom plug was opened immediately to remove the buffer competently before applying the lysate cell extract into the spin column. Subsequently, the whole supernatant was applied to this column and incubated for 1 hour at room temperature in an orbital shaker to increase the binding ability of the His-tag-VpC-terminal domain on the Ni-NTA column. After that, the bottom plug was removed, and the flow-through was collected; the column was then washed twice with a solution containing 50 mM imidazole. Finally, the His-tag-VpC-terminal domain was collected with the elution containing 250–300 mM imidazole, and its purity was analysed by 12% SDS-PAGE. The purified His-tag-VpC-terminal domain was dialyzed in the following buffer: 25 mM Tris-HCl pH 7.5 and 100 mM NaCl to evaluate its activity. The final concentration of the His-tag-VpC-terminal domain was approximately 1 mg/mL.
The basidiomycetes of Phellinus igniarius (Pia) and Phellinus pini (Ppi) were harvested at the Puhuong National Park of NgheAn province in Vietnam. Firstly, 200 g of each Pia and Ppi fruiting body was extracted with 800 mL methanol solvent at room temperature (25°C) over five days and then filtrated; the solvent was removed by the vacuum rotary method to collect the raw extract (20 g). Subsequently, 20 of the extract were partitioned continuously by three solvents, containing 5 g chloroform extract, 11 g of ethylacetate extract and 4 g of water extract. We used 11 g of ethylacetate extract from fruiting bodies of Pia and Ppi for inhibition assays against the His-tag-VpC-terminal domain.
The haemolytic activity assay against sheep erythrocytes was measured as described previously (Sun et al., 2007). First, the purified His-tag-VpC-terminal domain at 50 μg/mL was added into the left well created by an Oxford cup (6 mm diameter) with the volume of 50 μL, as opposed to the right well containing 50 μL tris-buffered saline (TBS) buffer as a negative control, which was placed on a blood agar base supplemented with 3% (vol/vol) sheep blood cells. After incubation at 37°C overnight, the presence of a brown zone around the well was considered evidence of a positive reaction. In parallel, the haemolytic titters of the His-tag-VpC-terminal domain were quantified using full sheep erythrocyte suspension as a substrate; the haemolytic assay was conducted as described previously (Mazzarino et al., 2015; Wang et al., 2009), with slight modifications. First, the packed erythrocytes were extracted from sheep blood by centrifugation at 112×g for 5 minutes at 4°C. Next, the plasma was discarded, and the whole packed erythrocytes were washed three times with four volumes of a standard saline solution (phosphate buffered saline [PBS], 0.9%); the packed erythrocyte suspension was generated by resuspending 100 μL packed erythrocytes in 900 μL of PBS. The haemolytic assay was performed in a 1.5 mL tube containing 300 μL of the diluted His-tag-VpC-terminal domain in PBS (from 25, 50, 75, and 100 μg/mL), mixed with an equal volume of the 2% (vol/vol) erythrocyte suspension, and finally, TBS buffer was added to reach a final volume of 1 mL. Subsequently, all tubes were carefully homogenised before being incubated for 60 minutes at 37°C and the centrifuged at 112×g for 5 minutes at 4°C to collect the total supernatants. Haemolytic activity of the His-tag-VpC-terminal domain was calculated based on the content of released haemoglobin at a wavelength of 490 nm. A 0.2% Tween 20 solution was used as a positive control and the PBS solution as a negative control. The erythrocyte autolysis in the assay tube without the His-tag-VpC-terminal domain was recorded and subtracted for each assay. All haemolytic activity experiments were performed in triplicate, and haemolytic activity (%) was calculated using the following equation (Zhang et al., 2007):
The phospholipase activity using egg yolk emulsion as substrate has been developed (Sun et al., 2007). The purified His-tag-VpC-terminal domain at 50 μg/mL was added into the left well created by an Oxford cup (6 mm diameter) with the volume of 50 μL, as opposed to the right well containing 50 μL TBS buffer as a negative control, which was placed on a LB agar supplemented with 1% (vol/vol) egg yolk emulsion. After incubation at 37°C overnight, the presence of an opalescent zone around the well was considered evidence of a positive reaction.
Metallic cations (Na+, K+, Ni2+, Cu2+, and Zn2+) were used as the chloride forms in PBS buffer (0.9%) to observe the impacts of various concentrations of cations on haemolysis. This experiment was similar to that used for haemolytic activity, except for the addition of different metallic cations. Briefly, each assay was conducted in a 1.5-mL tube containing 300 µL of the packed erythrocyte suspension, one volume unit of different concentrations of metallic cations (5, 25, and 50 mM) and 100 µg of the His-tag-VpC-terminal domain. Each tube was supplied with PBS buffer until the total reaction volume was 1 mL. After that, the tubes were centrifuged at 11,200×g for 10 minutes at 4°C before being thoroughly homogenised and incubated at 37°C for 60 minutes. Subsequently, the relative haemolytic activity of the His-tag-VpC-terminal domain was calculated based on the released haemoglobin at a wavelength of 490 nm. The tube containing all components, except the metallic cations, was the positive control. All measurements were conducted in triplicate.
The effects of two extracts from Pia and Ppi on haemolytic was determined in a similar manner as the haemolytic activity, except for the presence of various concentrations of Pia and Ppi extracts (5, 25, and 50 mM) in 0.9% PBS. Briefly, each assay was conducted in a 1.5-mL tube containing 300 µL of the packed erythrocyte suspension, one volume unit of different concentrations (mg/mL) of Pia or Ppi (2.5 × 10–2; 5.0 × 10–2; 7.5 × 10–2; 10–1) and 100 µg of the His-tag-VpC-terminal domain. Each tube was supplied with PBS buffer until the reaction volume was 1 mL. After that, the tubes were centrifuged at 11,200×g for 10 minutes at 4°C, thoroughly homogenised and incubated at 37°C for 60 minutes. After the incubation period, the relative haemolytic activity of the His-tag-VpC-terminal domain was calculated based on the content of the released haemoglobin at a wavelength of 490 nm. The tube containing all components, except the extracts, was the positive control. All measurements were conducted in triplicate.
All statistical analyses were performed with the Statgraphics Centurion XV. The data were subjected to a one-way analysis of variance. Mean separations were performed using the Tukey’s test. Differences at p < 0.05 were considered significant.
Results
The result of the PCR process showed the presence of a relevant amplicon of 450 base pairs (bp) in 1% agarose gel. It meant that the VpTLH coding for the C-terminal domain of the Vptlh amplified successfully. The size of VpTLH was similar to that in various amplicons of previous studies (Bej et al., 1999; Mahmuda et al., 2006) (Fig. 1A). After that, a consequence of the aligned nucleotide sequences between VpTLH and the Vptlh gene (accession number M36437.1) in the gene bank identified a precisely corroborated sequence similarity from 904 to 1,377 on both samples with 100% homology identity (Fig. 1B). We successfully cloned the NEB (turbo) cells and brought the pET11a-His-tag-VpTLH plasmid involving the exact nucleotide sequence of VpTLH coding the C-terminal domain of the Vptlh.
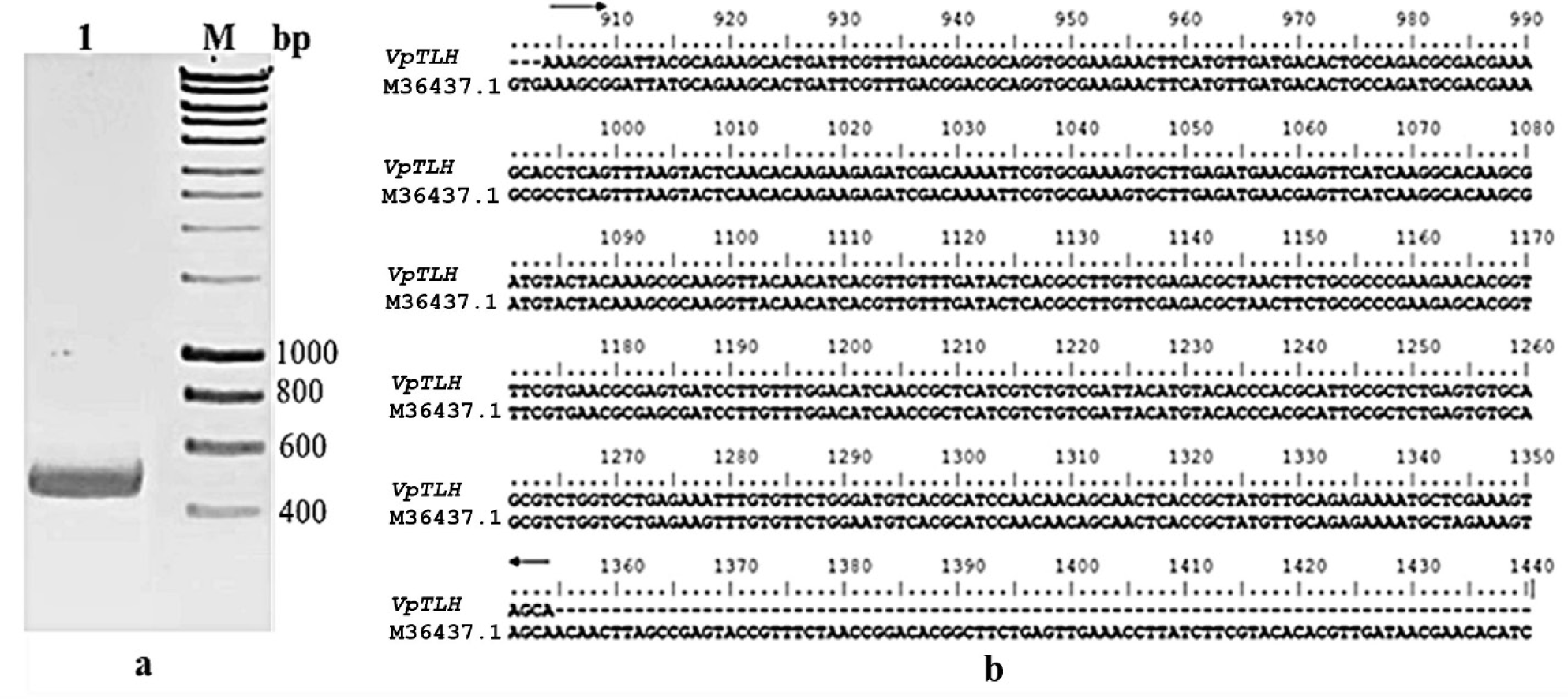
After the VpTLH sequence had been verified, the pET11a-Histag-VpTLH plasmid was transformed into BL21 (DE3) pLysS cells to check the expression of the His-tag-VpC-terminal domain. The His-tag-VpC-terminal domain was produced with the addition of a tag with an approximate molecular mass of 2 kDa. In LB medium containing 50 µg/mL ampicillin at 37°C, an expression survey of the His-tag-VpC-terminal domain in a varied range of IPTG concentration is shown in Fig. 2A. According to the length of the VpTLH sequence, the His-tag-VpC-terminal domain obtained an approximate molecular mass of 24 kDa. The impacts of the four concentrations of IPTG (mM) on the expression of His-tag-VpC-terminal domain were demonstrated by the presence of dark bands of ~45 kDa on four lanes from 1, 2, 3, and 4. But, in this study, electrophoresis results recorded appearances of dark bands of ~45 kDa on four lanes from 1, 2, 3, and 4 (SDS-PAGE), which were relevant to four induced bacteria cell samples by distinct concentrations of IPTG (0.5, 1.0, 1.5, and 2.0 mM). Once again, expression of the His-tag-VpC-terminal domain of ~45 kDa was refined strictly by the absence of the band of ~45 kDa in the U land; this is the land just contained the supernatant of bacterial culture solution at OD600 of 0.6 A without IPTG addition in the bacterial cultural media. Remarkably, equal expression levels of the His-tag-VpC-terminal domain at the three IPTG concentrations of 1.0, 1.5, and 2.0 mM were observed, whereas the lower expression of His-tag-VpC-terminal domain was found for the lowest IPTG concentration (0.5 mM). Combining these results led to the conclusion that the His-tag-VpC-terminal domain was expressed in a soluble state in the BL21 (DE3) pLysS cells under the induction of four IPTG concentrations.
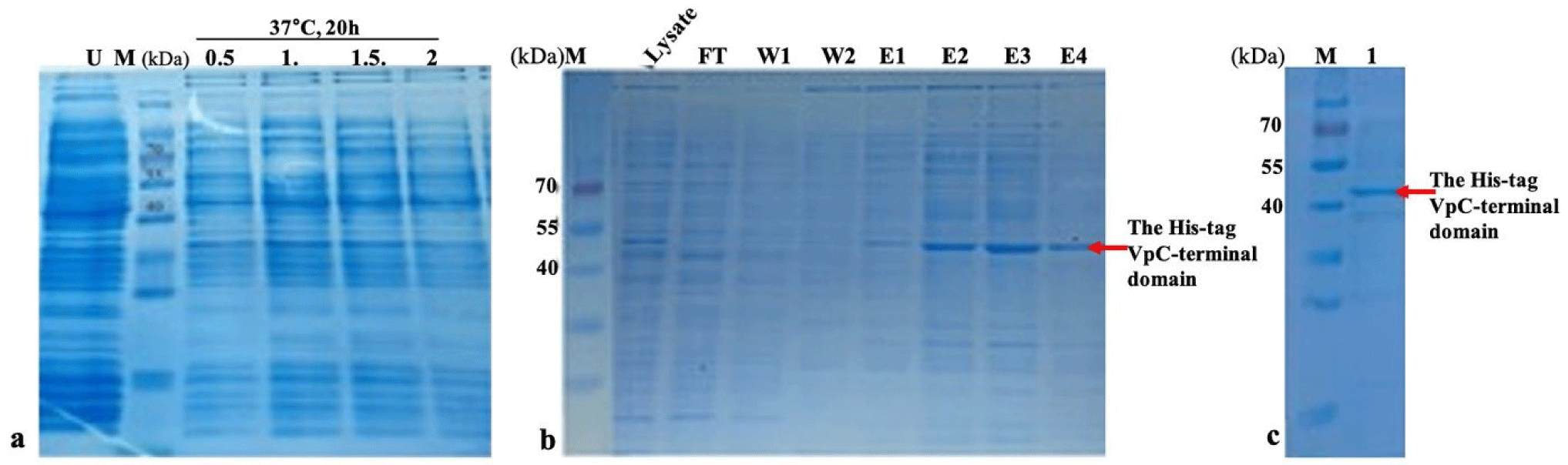
To refine the soluble state of the His-tag-VpC-terminal domain, the comp supernatant solution was applied to the Ni-NTA column to harvest and assess the molecular weight of His-tag-VpC-terminal domain. The results obtained from SDS-PAGE revealed a relevant band of ~45 kDa in the lysate sample, but this band was not visible in the flow-through and the wash solutions. Interestingly, bands of ~45 kDa in all elution solutions indicated that the His-tag-VpC-terminal domains were released from the Ni-NTA column after applying the elution solutions (Fig. 2A and 2B). In addition, after passing the dialysis step to recover the active properties, the molecular weight of the His-tag-VpC-terminal domain was confirmed again by the presence of a band at the same site of ~45 kDa on the 12% SDS-PAGE gel. This result demonstrated that the His-tag-VpC-terminal domain was expressed and purified successfully, although its apparent molecular weight was approximately two-fold higher compared to its predicted mass (around 22 kDa). Finally, the purity of the His-tag-VpC-terminal domain was over 90% (Fig. 2C).
The haemolytic activity of the purified His-tag-VpC-terminal domain against sheep erythrocytes was measured as described elsewhere (Zhang et al., 2001). Happened events in two separate wells on the blood agar used to check the haemolytic activity of the purified His-tag-VpC-terminal domain, with 50 μL of the purified His-tag VpTLH in the left well (well 1) and 50 μL of TBS buffer in the right well (well 2). After incubation for 20 hours at 37°C overnight, the appearance of a brown zone and reddish in outer well on the left side, in contrast to a well on the right side, provided evidence of a typical positive haemolytic reaction (Fig. 3A). Erythrocyte lysis in Fig. 4 initially proved the haemolytic action of the purified His-tag-VpC-terminal domain constituted by a 450-pb fragment.
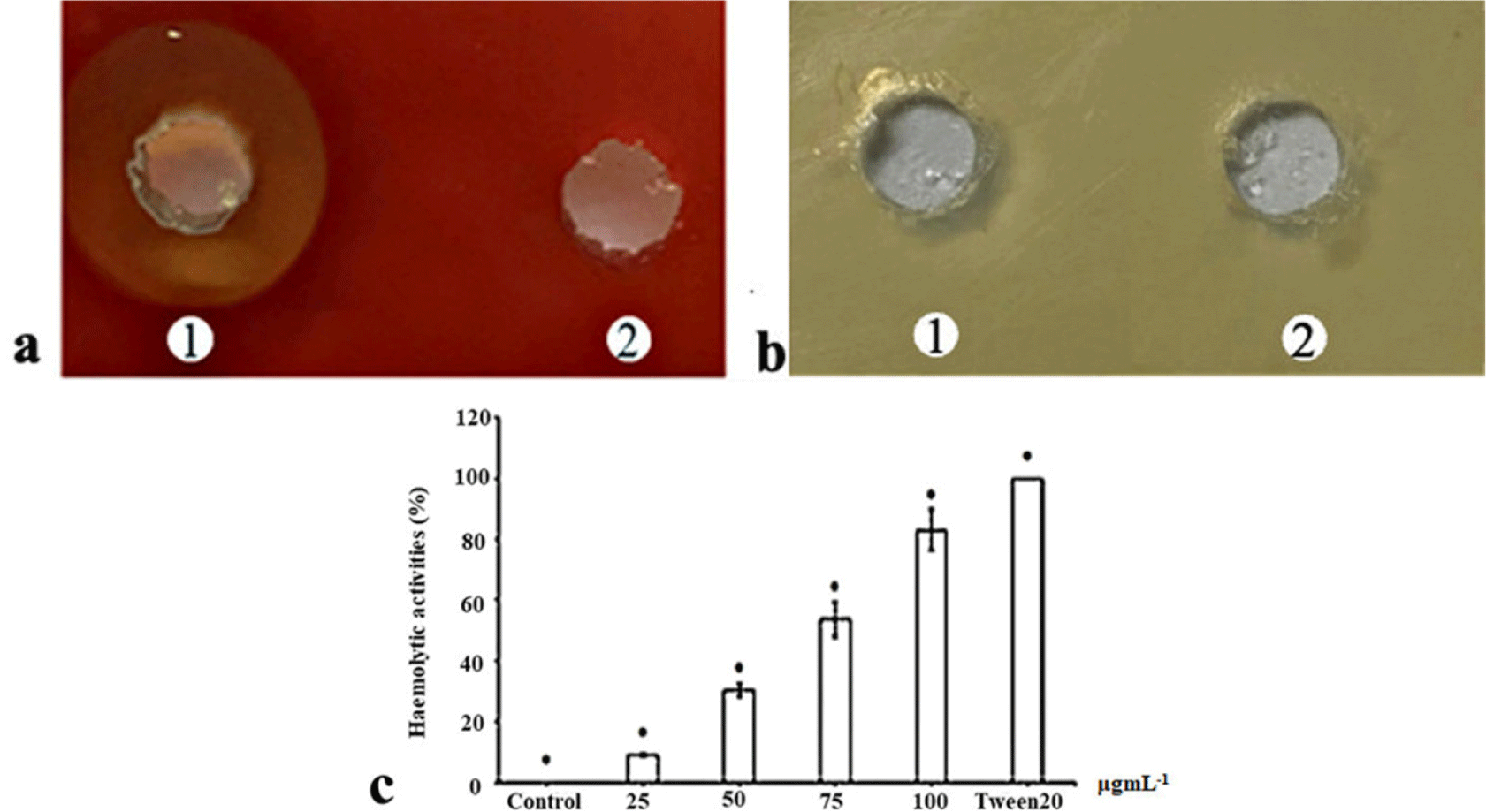
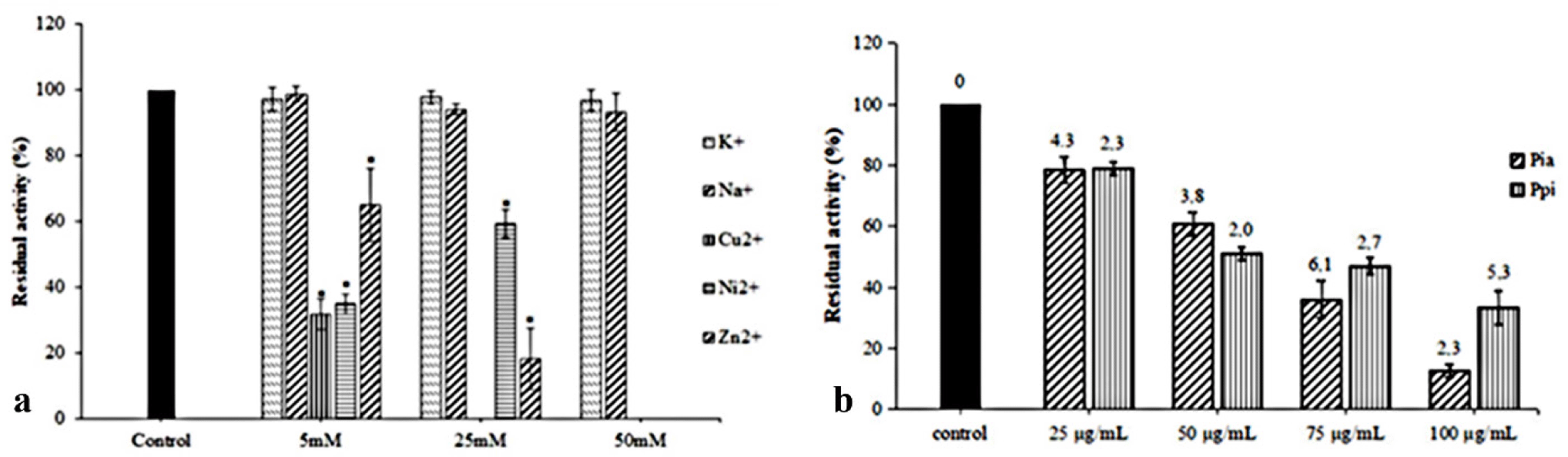
The phospholipase activity of the purified His-tag-VpC-terminal domain was examined on LB agar supplemented with 1% (vol/vol) egg yolk emulsion was an appropriate substrate for phospholipase enzyme. But enzymatic analysis in Fig. 3B revealed there was not opalescent zones around two well, indicating no phospholipase activity was detected in the above LB agar in the presence of LB agar.
The haemolytic titre was determined based on the colour change in the four assay tubes containing different concentrations of His-tag-VpC-terminal domain. All significant differences in the haemolytic activities of four His-tag-VpC-terminal domain concentrations are shown in Fig. 3C. There was a gradual decrease in the haemolytic activity against sheep erythrocytes with increasing levels of His-tag-VpC-terminal domain, suggesting that His-tag-VpC-terminal domain lysed the membrane of sheep erythrocytes without the attendance of lecithin. After subtracting by haemolytic activity 100% of Tween-20 (0.2%), the highest haemolytic activity of 85% was seen in the tube containing 100 μg of His-tag-VpC-terminal domain. Medium haemolysis from 35% to 60% was observed for the tubes containing 50 and 25 μg of the purified His-tag-VpC-terminal domain, and a minimum haemolysis of 15% was observed in the tubes with 25 μg of the purified His-tag-VpC-terminal domain. The results for the four tubes differed significantly by Tukey’s test (p < 0.05), indicating significant differences in the haemolytic activities of the purified His-tag-VpC-terminal domain in the four tested concentrations.
Monovalent or divalent cations were components of the reaction mixture and play an essential role in metalloproteins, particularly in haemolysin. Various cations can influence the activities of ldh and tlh (Jia et al., 2010; Vazquez-Morado, 2021). Therefore, five typical cations were used in this study to evaluate their impacts on the haemolytic ability of the His-tag-VpC-terminal domain. Significant differences in Fig. 4A showed monovalent ions Na+ and K+, did not interfere significantly with the relative haemolytic activities of the His-tag-VpC-terminal domain while three divalent cations Ni2+, Zn2+, and Cu2+ decreased drastic to the relative haemolytic activities of the His-tag-VpC-terminal domain. Nevertheless, two Zn2+ and Ni2+cations only begun different effects on the activities of His-tag-VpC-terminal domain at a concentration of 50 mM whereas the relative haemolytic activity of the His-tag-VpC-terminal domain could not measure in the presence of Zn2+ (Fig. 4A).
The impacts of raw extracts of Pia and Pp; on the haemolytic activity are described in Fig. 4B. The result in this experiment showed both Pia and Ppi extracts ruptured the erythrocytes at all concentration with significant differences (p < 0.05). As shown in Fig. 4B, the residual activity of His-tag-VpC-terminal domain reached an average level of 78.37 ± 4.26% to 78.37 ± 4.26% at the lower concentration (2.5 × 10–2 mg/mL) of two extracts of Pia and Ppi, whereas a higher effective inhibition was found at 10–1 mg/mL). These results suggest that extracts of Pia and Ppi can inhibit haemolytic activity of the His-tag-VpC-terminal domain (Fig. 4B).
Discussion
The haemolytic activity is followed by phospholipase activity (Shinoda, 1991; Vazquez-Morado, 2021). There is substantial evidence that the C-terminal domain maintains the conserved catalytic triad Ser153-Asp390-His393 of the SGNH hydrolysis enzyme, in which the SGNH motif is divided into four blocks. The alignment of the SGNH motif between the His-tag VpC-terminal domain, with Vptlh, Vctlh, and VhTlh, is shown in Fig. 5. As previously mentioned, the his-tag-terminal domain in the Vptlh overall structure. is involved in the conserved catalytic triad Ser153-Asp390-His393 of the SGNH hydrolysis enzyme. The docking method indicated that both residues, Asp390 and His393, did not connect to Ser153 to constitute the catalytic triad of the active site. Interestingly, as seen in Fig. 5, D390 and H393 in the catalytic triad were present in block 4 of the three haemolysins (His-tag VpC-terminal domain, Vptlh and Vhtlh), although Vctlh did not contain the D390 residue. However, based on the lysed sheep erythrocyte membrane, there was a stable haemolysin activity of the His-tag VpC-terminal domain, although without the attendance of the key residue Ser153, as reported previously studies (Jang et al., 2017; Li et al., 2013).

Most heterologous proteins are frequently expressed in an insoluble form, which results in the loss of their biological activity (Chow et al., 2006; Singh & Panda, 2005). In this study, the results of expression and purification of the His-tag-VpC-terminal domain in SDS-PAGE gel reflected the persistence of a single band with its relevant molecular mass of 47 kDa. These observations indicated that the His-tag-VpC-terminal domain of V. parahaemolyticus was successfully expressed in a soluble protein in E. coli cells, and its molecular weight was two-fold higher than its predicted weight. However, identification of the molecular weight of His-tag-VpC-terminal domain was not possible because its oligomeric conformation had not confirmed yet. Finally, the total purified His-tag-VpC-terminal domain amount in our study was approximately 280 μg for each 100 mL culture of LB medium. The individual haemolytic activity of the His-tag-VpC-terminal domain proposed a novel insight about the responsibility of the His-tag-VpC-terminal domain into the class of thermopile haemolysin in general. Furthermore, the Vptlh gene in V. parahaemolyticus has an approximately 83% sequence identity with the Vatlh gene of V. alginolyticus (Xie et al., 2005). Hence, we evaluated the haemolytic activities between the purified His-tag-VpC-terminal domain with two Vptlhs (1,233 bp and 1,586 bp) and the Vatlh from V. alginolyticus (Table 1). As shown in Table 1, the haemolytic activities of two haemolysins of Vptlh of 1,233 bp or 1,586 bp in length have refined upon on the presence of phosphatidylcholine or lecithin substrates. With the same content of 100 μg, the haemolytic action of His-tag-VpC-terminal domain was more than one-half compared to that in Vatlh (1,268 bp) and Vptlh (1,233 bp) and similar to that of 85% in Vptlh (1,586 bp). In summary, our obtained results determined the responsivity of the His-tag-VpC-terminal domain (450 bp) in the haemolytic reaction on the erythrocyte membrane as well as that of the full-length Vptlh (1,586 bp), and furthermore the haemolytic ability of the His-tag-VpC-terminal domain was independent on the persistence of lecithin.
The impacts of metallic cations on the haemolytic activities of two haemolysins, His-tag-VpC-terminal domain and Vatlh, are shown in Table 2. In which, monovalent ions Na+ and K+ did not influence differentially to the relative haemolytic activities in both haemolysins; but three remaining divalent cations Ni2+, Zn2+, and Cu2+ influenced the haemolytic activity, while only Cu2+ inhibited the action of the tlh of Vatlh and the His-tag-VpC-terminal domain. In detail, the relative haemolytic activity of the His-tag-VpC-terminal domain was decreased to 18.14% in the presence of Zn2+, whereas that of Vatlh was lost mostly because of the addition of Ni2+. A clear inhibition of the His-tag-VpC-terminal domain was observed at a concentration of 50 mM of the three divalent cations. These findings agreed about the impacts of these divalent cations to the haemolytic activity of haemolysin from V. alginolyticus in previous studies (Jia et al., 2010). Besides, effects of Pia, Ppi on the haemolytic activity of the His-tag-VpC-terminal domain and other compounds on the Vptlh showed in Table 3. Based on the inhibition ability of both Pia and Ppi extracts on the activity of the His-tag-VpC-terminal domain, we propose a mode of action for these extracts to inhibit the haemolytic activity for the His-tag-VpC-terminal domain like the Vptlh in total length (Fig. 6).
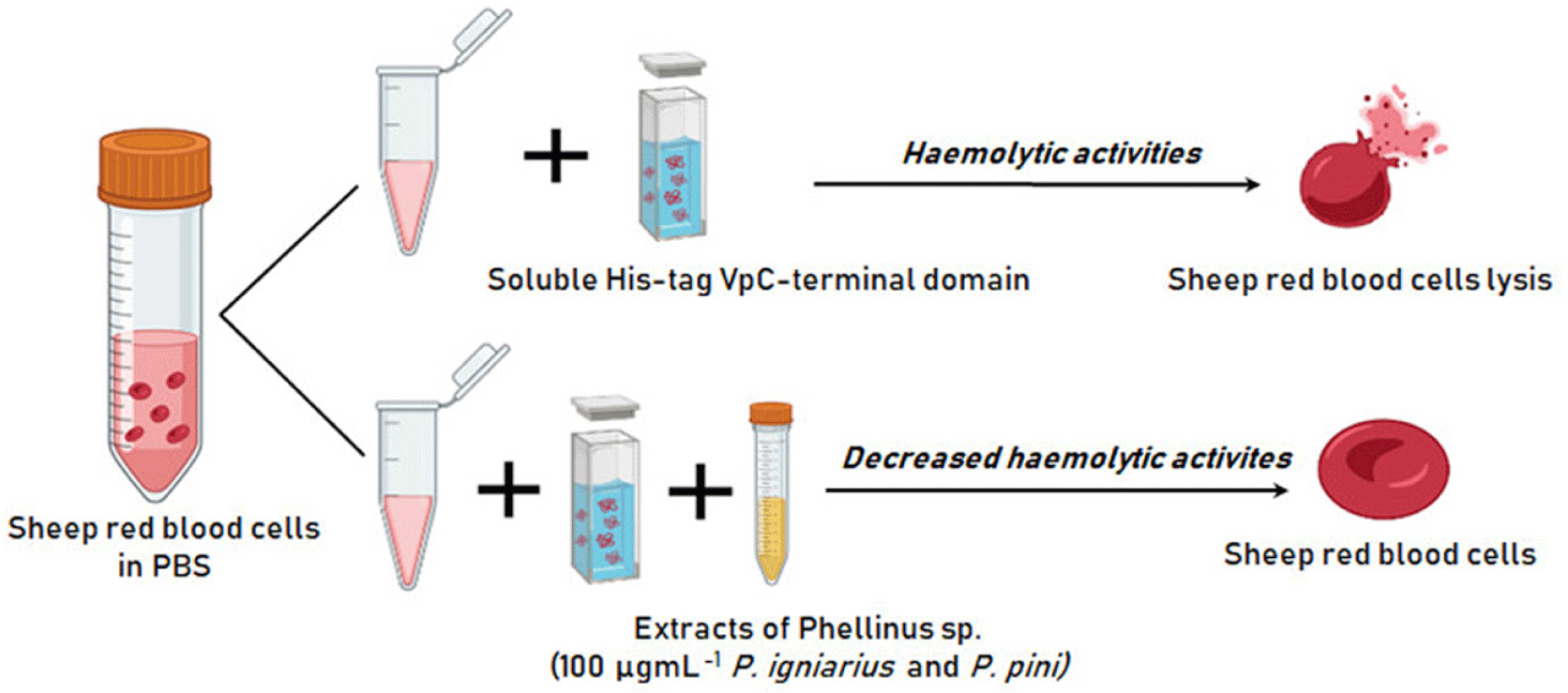
Conclusion
In summary, our study successfully produced the His-tag-VpC-terminal domain in soluble form. The content of 100 µg of the His-tag-VpC-terminal domain caused haemolytic activity of up 80% compared to that in the three remaining contents. Without adding lecithin, the His-tag-VpC-terminal domain disrupted the erythrocyte membranes in the blood agar and the PBS (0.9%) medium. Furthermore, this domain demonstrated not to involve the phospholipase activity in the LB agar supplemented with 1% (vol/vol) egg yolk emulsion. These results determined the His-tag-VpC-terminal domain played a vital responsibility in causing the haemolytic activity without the required activation by the phospholipase enzyme. Extracts of Pia and Ppi at 10–1 mg/mL inhibited the activity of the His-tag-VpC-terminal domain from 67.7% to 87.42%, respectively. The higher the concentration of an extract of Pia or Ppi used in each inhibition assay caused the higher the inhibition of the haemolytic efficiency of the His-tag-VpC-terminal domain. Consequently, the application of the His-tag-VpC-terminal domain in researching potential derivatives to inhibit the thermolabile hemolysin promises advantages more than using the thermolabile Vptlh from V. parahaemolyticus.