Introduction
Periodontitis is a common chronic multifactorial inflammatory disease in the periodontal tissues and is one of the high-prevalence chronic conditions in the world (Eke et al., 2020; Papapanou & Susin, 2017; Papapanou et al., 2018). Periodontitis usually occurs at supporting structures of the teeth such as alveolar bone, gingiva, periodontal ligament, and root cementum and is associated with dental plaque biofilm formation (Papapanou et al., 2018; Potempa et al., 2017; Teeuw et al., 2017). It is characterized by increased levels of subgingival pathogens, resulting in alveolar bone loss (ABL) and the progressive destruction of tooth-supporting structures (Ratheesh et al., 2018; Suvan et al., 2020). It is an important public health problem and is often called a silent disease because it rarely shows obvious signs and symptoms when it has progressed to its terminal stages (Papapanou & Susin, 2017). Aggregatibacter actinomycetemcomitans and Porphyromonas gingivalis are known as the major pathogens that induce periodontitis (Slots, 2017). Additionally, it is characterized by localized chronic inflammatory reactions with an increased serum cytokine level by pro-inflammatory cytokines, including tumor necrosis factor-alpha (TNF-α), interleukin (IL)-6, IL-10, and IL-17 (Ide et al., 2016; Potempa et al., 2017).
Periodontitis is treated through non-surgical and surgical treatments following the progress of the disease. In the case of non-advanced periodontitis, it is usually treated by nonsurgical methods, including scaling, root planing, and topical or oral antibiotic treatment (Costa et al., 2019; Matarese et al., 2017; Spanou et al., 2020). In contrast, patients with severe periodontitis required dental surgery such as bone grafting, flap surgery, and soft tissue grafting (Bai et al., 2018; Shrestha et al., 2018; Zufía et al., 2019). Therefore, many researchers have been developing new therapeutic approaches to investigate more effective therapy methods with bioactive substances for periodontitis and have reported that substances with antibacterial, antibiofilm, antimicrobial, anti-inflammatory, and anti-osteoclastic activities can be used as therapeutic agents for periodontitis (Kim et al., 2018; Shrestha et al., 2018; Spanou et al., 2020; Sun et al., 2019; Teughels et al., 2020).
Marine organisms, including Annelida, Chordata, Cnidaria, Phaeophyceae, and Rhodophyta, produce numerous bioactive substances since they have to face high competition for a unique living environment, such as high concentrations of salts, high pressure, and low concentration of oxygen (Batool et al., 2022; De Domenico et al., 2019; Jiang et al., 2012; Kim et al., 2016; Kim et al., 2022b; Oh et al., 2021). Additionally, many studies have investigated marine bioactive substances (MBS), including polyphenols, collagens (COL), polydeoxyribonucleotides, peptides, polysaccharides, chitins, and chitosans, and their therapeutic and pharmacological activities, such as antibacterial, antibiofilm, antimicrobial, antioxidant, and anti-inflammatory activities (Chandika et al., 2021; Heo et al., 2018; Kim et al., 2019a; Kim et al., 2020; Kim et al., 2021b; Kim et al., 2022a; Nguyen et al., 2016). For their huge biological activities, MBS has been increasing attention as a therapeutic and pharmaceutic agent for human diseases, including atopic dermatitis, idiopathic pulmonary fibrosis, osteoporosis, and periodontitis.
Based on these facts, this review summarizes the current state of MBS against periodontitis treatment, including the therapeutic effects and application forms of MBS. To facilitate their discussion, these breakthroughs will be classified as in vitro, in vivo, and clinical studies. Finally, we will discuss the challenges and recent trends in MBS research for periodontitis treatment.
Materials and Methods
We conducted a publication analysis on MBS against periodontitis with the use of the keywords “Marine” & “Periodontitis” via Google Scholar, PubMed, and Web of Science. We examined all language articles (2,580 articles) published from January 2019 to July 2022 (Fig. 1). In this research, we searched to find articles, which were conducted in vitro, in vivo (animal model), and clinical experiments, on the treatment of MBS against periodontitis. We reviewed the in vitro, in vivo, and clinical articles on the potential of MBS against periodontitis treatment throughout the article content (in the abstract, methods and materials, results, and discussion sections).
We excluded 1,496 several articles, such as symposium abstracts, reviews, book chapters, and dissertations, to avoid repetitive article counting. However, we examined the content of articles that were mentioned in the review articles and included them. Moreover, 1,035 studies simply described MBS or periodontitis in the introduction or discussion part. Therefore, we considered that 2,531 studies are irrelevant to the therapeutic effect and application forms of MBS against periodontitis and excluded them.
Results
Fig. 2 illustrates the number of studies on MBS for periodontitis published each year. We found that the number of studies on the therapeutic effect of MBS for periodontitis is increasing from 2019 to 2022. This trend indicated that characterizing the therapeutic efficacy and application of MBS, as a novel and safe treatment against periodontitis, is increasing interest.
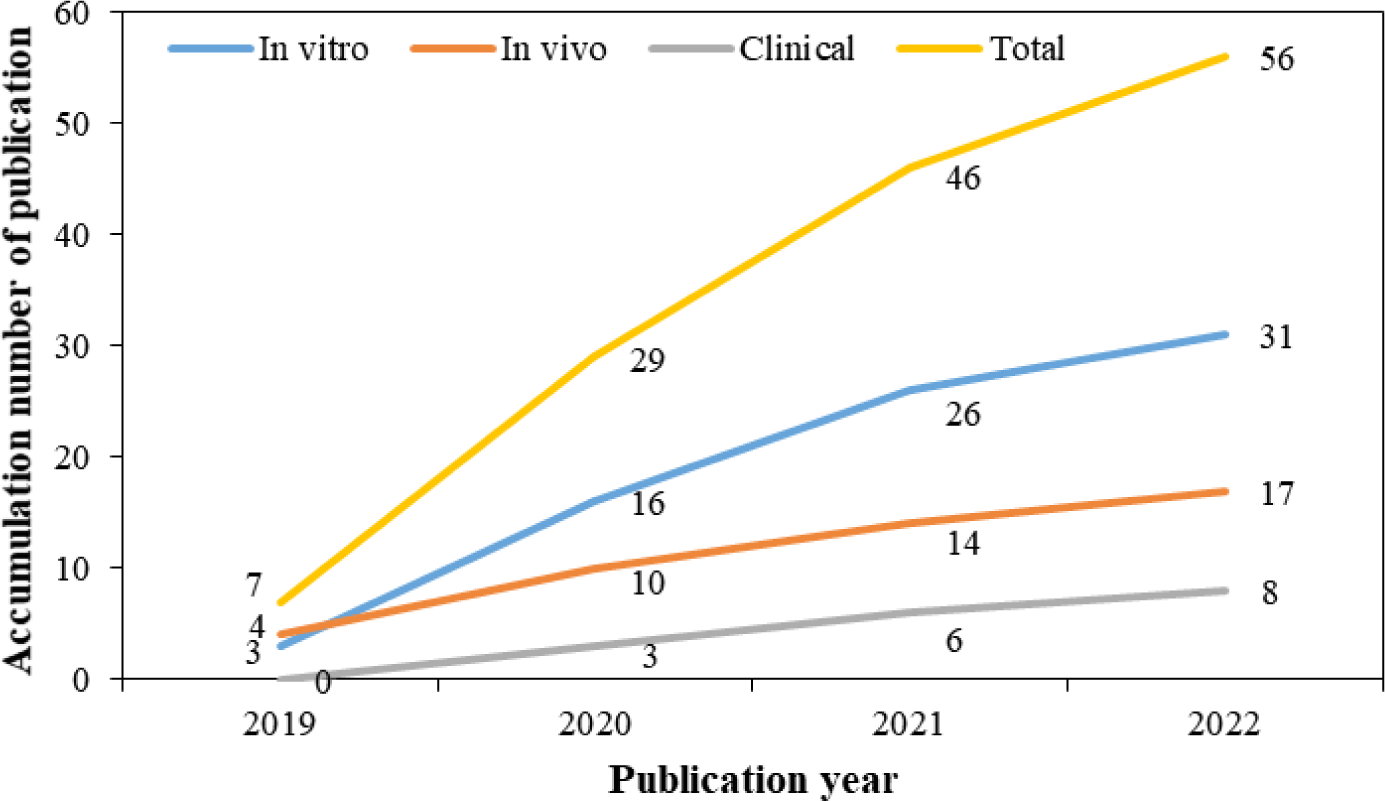
All studies (n = 56) were analyzed to identify the prevailing sources of MBS extraction (Fig. 3A) and twenty-three species, except unknown sources, were identified. Although extraction sources of most MBS did not know due to researchers purchasing MBS from material companies, MBS was mainly extracted from three classes (Phaeophyceae, Chordata, and Annelida) in the literature provided information on extraction sources.
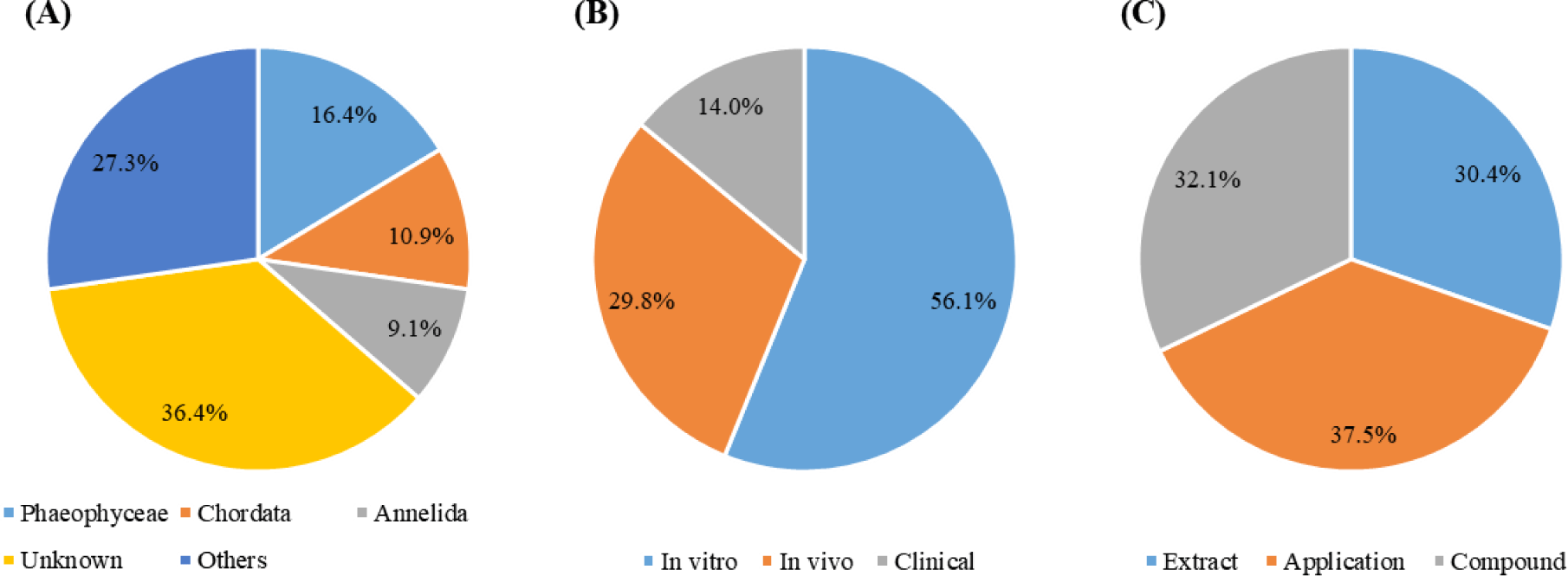
Especially, Phaeophyceae (Brown algae) includes numerous genera, such as Ecklonia, Agarum, Sargassum, and Turbinaria, and contains potential bioactive substances, because it produces secondary metabolites, including alginic acid, fucoidan, polyphenol, alkaloids, and fatty acids (Tapia-Martinez et al., 2019). MBS extracted from Phaeophycea show antibacterial, anticoagulant, antithrombotic, antitumor, antiviral, and anti-inflammatory properties (Achmad et al., 2020b; Leal et al., 2018; Ren et al., 2019). Additionally, several biopolymers and bioactive substances, such as chitosan, COL, and fish oil, are extracted from Chordata and exhibit antioxidant, antimicrobial, and anti-inflammatory activities and biocompatibility (Asari et al., 2021; Oh et al., 2021; Satpathy et al., 2021). Arenicola marina inhabits intertidal soft sediments from the Arctic to the Mediterranean and contains peptides and extracellular hemoglobin with antimicrobial and anti-hepatotoxicity activities (De Cubber et al., 2019; Le Daré et al., 2021; Umnyakova et al., 2018). Based on the pharmacological properties of the marine resources, many studies analyzed in this review investigated the potential of MBS for periodontitis treatment.
As mentioned in the method part, the experiments discussed herein will be classified as in vitro studies, in vivo studies, and clinical studies. Most experiments were conducted using in vitro models (31 studies), followed closely by in vivo studies (17 studies), suggesting that clinical studies (8 studies) are rarely conducted (Fig. 3B).
Two studies have developed jellyfish COL-based scaffolds (Arslan & Kantarcioğlu, 2019; Rachmawati et al., 2021). One of these studies, a 2019 study fabricated Salvadora persica aqueous extract-loaded Rhizostoma pulmo COL (JC) scaffold and evaluated their effect on human periodontal ligament fibroblasts (HPLF) (Arslan & Kantarcioğlu, 2019). All fabricated scaffolds showed no cytotoxic effect and, in particular, the S. persica extract-unloaded JC scaffold enhanced cell proliferation 14 days after incubation. Other studies revealed that Aurelia aurita-derived COL scaffolds did not show cytotoxicity up to 1,000 μg/mL on human mesenchymal stem cells (hMSC) (Rachmawati et al., 2021).
Previous studies have confirmed that bioactive substances extracted from Phaeophyceae have therapeutic potential against periodontitis (Han et al., 2021; Jung et al., 2021; Kim et al., 2019b; Kim et al., 2021a; Magesh et al., 2020; Alden et al., 2022). Two of these studies investigated whether Ecklonia cava ethanol (EtOH) extract (ECE) has a therapeutic effect on periodontitis (Jung et al., 2021; Kim et al., 2019b). ECE presented no cytotoxicity in P. gingivalis lipopolysaccharide (Pg LPS)-stimulated RAW 264.7 and inhibited Pg LPS-induced production of inflammatory mediators, including nitric oxide (NO) and prostaglandin E2 (PGE2), and cyclooxygenase-2 (COX-2) activity (Kim et al., 2019b). Additionally, ECE decreased mRNA expression of inducible nitric oxygen synthase (iNOS), TNF-α, IL-1β, and IL-6 by activating the Nrf-2/heme oxygenase-1 (HO-1) pathway and suppressing the phosphorylation of nuclear factor kappa-light-chain-enhancer of activated B cells (NF-κB) pathway. The other study confirmed that ECE inhibited the production of PGE2 and reactive oxygen species (ROS) without cytotoxicity on human gingival fibroblast (HGF) (Jung et al., 2021). Another study investigated that Agarum clathratum, which has antioxidant and anti-microbial properties, exhibits potential for periodontitis treatment on Pg LPS-stimulated HGF (Han et al., 2021). A. clathratum methanol (MeOH) extract inhibited mRNA and protein expression of IL-1β without cytotoxicity on Pg LPS-stimulated HGF. A 2021 study isolated epiloliolide (ELL), a monoterpene lactone derived from the degradation of carotenoids, from Sargassum horneri and demonstrated that ELL promotes cell proliferation and migration in Pg LPS-stimulated human periodontal-derived ligament cell (HPDL) (Kim et al., 2021a). Additionally, ELL showed anti-inflammatory activity by suppressing protein expression of COX-2, iNOS, and NOD-like receptor pyrin domain-containing protein 3 (NLRP3) and mRNA expression and release of TNF-α, IL-1β, and IL-6. ELL induced cell mineralization and enhanced mRNA expression of alkaline phosphatase (ALP), osteopontin (OPN), and runt-related transcription factor 2 (RUNX-2) by activating the phosphorylation of the Protein kinase A (PKA)/cAMP response element-binding protein (CREB) pathway. Another study confirmed Turbinaria conoides petroleum ether (PE) extract has antibacterial activity on Streptococcus sp. and Actinomycetes sp. (Alden et al., 2022). The other study evaluated the antibacterial effect of Sargassum wightii PE extract on Streptococcus mutans (Magesh et al., 2020).
Rhodophyta is a groups of seaweeds with pharmacological properties and contains numerous natural compounds (Cotas et al., 2020). Therefore, two studies have investigated that extract and/or compounds from Rhodophyta have therapeutic potential for periodontitis (Cherian et al., 2019; Sharan & Vennila, 2021). A study obtained Kappaphycus alvarezii MeOH extract (KAM) and isolated marine bromophenol from KAM (KAB) (Cherian et al., 2019). KAB and KAM showed antibacterial activity on P. gingivalis and decreased Arginine specific gingipain (Rgp) activity and hemagglutination. Additionally, KAB and KAM suppressed the mRNA expression of peptidyl arginine deiminase (PPAD), Rgp, and hemagglutinin A (HgA). The other study obtained K. alvarezii extracts using different polarity solvents, including EtOH (KAE), ethyl acetate (KAEA), and chloroform (KAC) (Sharan & Vennila, 2021). After that, the authors evaluated the pharmacological effect of K. alvarezii extracts. All extracts showed high antioxidant activity and antimicrobial activity on Bacillus subtilis, Escherichia coli, Klebsiella pneumoniae, Pseudomonas aeruginosa, and Candida albicans. Additionally, KAC showed high compatibility on Vero (Normal fibroblast cell line derived from African green monkey) and KAE decreased cell viability of KB-3-1 (Human epidermoid oral carcinoma cell line), which is an oral cancer cell line.
A 2020 study investigated the pharmacological activity of several EtOH extracts of Phaeophyceae and Rhodophyta on periodontitis (Yoon et al., 2020). In this study, EtOH extracts were obtained from 3 Phaeophyceae (Distromium decumbens [DDEE], Myelophycus simplex [MSEE], and Scytosiphon canaliculatus [SCEE]) and 3 Rhodophyta (Asparagopsis taxiformis [ATEE], Galaxaura fastigiata [GFEE], and Hypnea japonicay [HJEE]) and then evaluated their anti-inflammatory effect on Pg LPS-stimulated HGF. All extracts did not show cytotoxicity on Pg LPS-stimulated HGF. GFEE and SCEE suppressed only NO production, however, ATEE and HJEE inhibited the protein expression of iNOS, as well as NO production.
Three studies developed Chordata-derived biomaterial-based applications for periodontitis treatment (Achmad et al., 2020a; Elango et al., 2020; Zhou et al., 2020). One study confirmed whether Chanoschanos scale chitosan-based gel has high antibacterial activity on A. actinomycetemcomitans and P. gingivalis, similar to a positive control (Metronidazole) (Achmad et al., 2020a). A 2020 study has developed a tilapia COL/sodium alginate/titanium oxide (TiO2) 3D matrix (CB3D) (Elang et al., 2020). CB3D enhanced the level of ALP and osteocalcin (OCN) without cytotoxicity on HPLF. Additionally, CB3D increased the mRNA expression of COL, ALP, OCN, and RUNX-2 and protein expression of COL, OCN, and RUNX-2. These results indicate that CB3D promoted cell mineralization in HPLF. The other study developed a hydrogel based on tilapia COL and poly (vinyl alcohol) (PVA) (Zhou et al., 2020). When the increasing ratio of tilapia COL in hydrogel, hydrogel enhanced the cell viability, proliferation, and adhesion in HPLF and HGF. A 2020 study has fabricated tilapia COL/chitosan film, loaded with resveratrol or celastrol, as a dental implant for periodontitis (Wang et al., 2020b). Tilapia COL/chitosan film regardless of cross-linking using N-ethyl-N’-(3-(dimethylamino)propyl)carbodiimide/N-hydroxysuccinimide shows the highest cell proliferation and promotes the differentiation of bone marrow-derived macrophage (BMM) to tartrate-resistant acid phosphatase (TRAP)-positive cell.
Marine sponges belonging to the genus Agelas constitute several marine pyrrole-imidazole alkaloids with various biological properties (Rane et al., 2014). Therefore, one study investigated whether alkaloids isolated from the Agelas dilatata has therapeutic potential for periodontitis (Pech-Puch et al., 2020). In this study, eight alkaloids were isolated from A. dilatata and evaluated for antibacterial activity on P. aeruginosa. Bromoageliferin, an alkaloid isolated A. dilatata, has the highest antibacterial effect on P. aeruginosa (minimum inhibitory concentration = 8 μg/mL) and inhibited biofilm formation by P. aeruginosa.
A study confirmed that biseokeaniamide A, isolated Okeania sp., exhibits anti-inflammatory activity on LPS-stimulated RAW 264.7 (Ohno et al., 2020). Biseokeaniamide A inhibited NO production and IL-1β expression in LPS-stimulated RAW 264.7. Moreover, Biseokeaniamide A decreased protein expression iNOS by suppressing the phosphorylation of nuclear factor of kappa light polypeptide gene enhancer in B-cells inhibitor, alpha (IκBα).
A 2020 study purified a cold-adapted dextranase (Cadex2870) from Catenovulum sp. and evaluated its biological activity for periodontitis treatment (Deng et al., 2020). Cadex2870 showed anti-biofilm activity on S. mutans and scavenging activity on the formed plaque biofilm of S. mutans.
HEMARINA-M101 (M101) is natural extracellular hemoglobin derived from the A. marina and is considered an extracellular oxygen carrier having high oxygen binding capacity (Batool et al., 2020). For these desirable properties of M101, M101 is used in anti-inflammatory and anti-fibrotic applications (Batool et al., 2020; Batool et al., 2022; Özçelik et al., 2021). One study investigated whether M101 has antibacterial and anti-inflammatory activity using Pg LPS-stimulated and P. gingivalis-infected human oral epithelial cell (HOEC) (Batool et al., 2020). M101 showed antibacterial activity on P. gingivalis. Additionally, M101 inhibited mRNA and protein expression of TNF-α and suppressed protein expression of IL-1β, IL-8, regulated upon activation, normal T cell expressed and presumably secreted (RANTES), and interferon gamma-induced protein (IP)-10 without cytotoxicity on inflammatory reaction-induced HOEC. Additionally, M101 inhibited mRNA expression of NF-κB and receptor activator of nuclear factors κB ligand (RANKL), enhanced the release of tissue inhibitors of matrix metalloproteinases (TIMP)-2, macrophage colony-stimulating factor human (M-CSF), and intercellular adhesion molecule 1 (ICAM-1), mRNA expression of Resolvin-E1 receptor, and protein expression of platelet-derived growth factor-BB (PDGF-BB), transforming growth factor (TGF)-β1, IL-10, IL-2, IL-4, IL-11, and IL-15. Moreover, another study investigated that M101 has therapeutic potential against P. gingivalis-infected or hypoxia-induced HOEC (Batool et al., 2022). M101 showed antioxidant and anti-hypoxic activities. Additionally, M101 increased cell migration and suppressed mRNA expression of glucose transporters (GLUT-1), GLUT-3, hypoxia-inducible factor (HIF)-1α, and matrix metalloproteinase (MMP)-9. To apply M101 for periodontitis treatment, a 2021 study fabricated hyaluronic acid-based hydrogel containing M101 (Xn2%-HA1%-M101) (Özçelik et al., 2021). The fabricated hydrogel showed antibacterial activity on P. gingivalis without cytotoxicity on HOEC.
One study investigated whether liposomes extracted from the skin of Dosidicus gigas have a potential for periodontitis treatment (Kao et al., 2021). In the RANKL/M-CSF-stimulated RAW 264.7 model, squid skin liposomes show an anti-inflammatory effect by suppressing the PGE2 level. Additionally, the squid skin inhibited osteoclast maturation by inhibiting TRAP activity and mRNA expression of NFATc1, c-Src, receptor activator of nuclear factor κB (RANK), Cathepsin K, TRAP, and dendritic cell-specific transmembrane protein (DC-STAMP).
Nine studies did not indicate information on extraction sources due to purchasing from material companies (Campana et al., 2020; Guo et al., 2021; Johnson et al., 2020; Li et al., 2021; Özdoğan et al., 2021; Ribeiro-Vidal et al., 2020; Tang et al., 2022; Xu et al., 2020; Zhou et al., 2021). One of these studies fabricated doxycycline-containing chitosan/carboxymethyl chitosan nanoparticles (Dox:CS/CMCS-NPs) and evaluated several biological activities, including antibacterial, anti-inflammatory, and cell proliferative activities (Xu et al., 2020). The fabricated nanoparticles exhibited an antibacterial effect on P. gingivalis and suppressed mRNA and protein expression of IL-1β without cytotoxicity on HGF stimulated by a combination treatment of Pg LPS and adenosine triphosphate (ATP). Additionally, Dox:CS/CMCS-NPs inhibited mRNA and protein expression of NLRP3 and apoptosis-associated speck-like protein containing a caspase recruitment domain (ASC) and mRNA expression and activation of Caspase-1. A 2020 study investigated that surfactin-loaded ĸ-carrageenan oligosaccharide-entangled cellulose nanofibers have therapeutic potential against periodontitis (Johnson et al., 2020). The fabricated nanofibers showed compatibility on RAW 264.7 and exhibited antibacterial activity on P. gingivalis and anti-biofilm activities on P. gingivalis and S. mutans. Moreover, nanofibers suppressed the metabolic activity of P. gingivalis and S. mutans, enhanced the malondialdehyde (MDA) level, and induced cell death of P. gingivalis and S. mutans. Another study on the biological activity of eicosapentaenoic acid (EPA) and docosahexaenoic acid (DHA) demonstrated that EPA and DHA have antibacterial activity on Actinomyces naeslundii, Fusobacterium nucleatum, Streptococcus oralis, Veillonella parvula, P. gingivalis, and A. actinomycetemcomitans and anti-biofilm activity on Streptococcus sp. and Aggregatibacter sp. (Ribeiro-Vidal et al., 2020). Another study also investigated the therapeutic effect of EPA using Pg LPS (normal and heat-inactivated form)-stimulated HGF (Zhou et al., 2021). In this study, they confirmed that EPA has an anti-inflammatory effect by suppressing mRNA expression of IL-1β and IL-6 and protein expression of IL-6 on Pg LPS-stimulated HGF. Moreover, EPA inhibited mRNA and protein expression of HO-1.
A 2020 study demonstrated that 2,2-bis(6-bromo-1H-indol-3-yl) ethanamine and its fluorinated analog, one of the marine bisindole alkaloids and its analog, have antibacterial, antimicrobial, and anti-biofilm activities on S. mutans. Additionally, both compounds showed a viable but non-culturable cell (VBNC) inducing effect and decreased oxacillin resistance against S. mutans. Another study developed gel formulations of atorvastatin solid dispersions with various mixture ratios (chitosan, lactic acid, pluronic F-68, and polyethylene glycols) (Özdoğan et al., 2021). All gel formations suppressed the release of IL-1β, IL-6, IL-8, IL-10, TGF-β1, TGF-β2, and TGF-β3. A 2021 study fabricated ginsenoside Rg1 and amelogenin-loaded injectable hydrogel, composed of glycol chitosan and aldehyde-modified hyaluronic acid, and evaluated its cytotoxicity (Guo et al., 2021). This study confirmed that the fabricated hydrogel did not show cytotoxicity on human bone marrow mesenchymal cells (HBMSC). Another 2021 study developed minocycline-loaded mouthwash containing natural polysaccharides, including chitosan and cyclodextrin (Li et al., 2021). The developed mouthwash showed the antibacterial and anti-biofilm effects on P. gingivalis. The other study investigated whether fucoidan-derived carbon dots (FDCD) have the biological potential for periodontitis treatment (Tang et al., 2022). FDCD showed antibacterial and anti-biofilm activity against Enterococcus faecalis and induced bacterial cell leakage. FDCD has non-cytotoxicity on MC3T3-E1 and RAW 264.7. Additionally, FDCD promoted ROS production in bacterial cells and increased macrophage migration.
Stichopus herrmanni has pharmacological effects, such as antibacterial, antifungal, and wound healing effects (Sarhadizadeh et al., 2014; Wahyuningtyas et al., 2019). Based on these activities, three studies investigated the therapeutic potential of S. herrmanni for periodontitis (Adam et al., 2022; Mulawarmanti et al., 2019a; Mulawarmanti et al., 2019b). One of these studies confirmed using the femoral defect guinea pig model that S. herrmanni-extracted bone grafts induced OCN expression and increased the number of osteoblast at the implanted site (Adam et al., 2022). Others investigated that sodium carboxymethylcellulose (CMC-Na) gel containing S. herrmanni powder has a therapeutic activity on periodontitis diabetes rat model and periodontitis mice, respectively (Mulawarmanti et al., 2019a; Mulawarmanti et al., 2019b). In a periodontitis diabetes rat model induced by P. gingivalis administration, fabricated CMC-Na gel inhibited the expression of IL-1β and IL-10 (Mulawarmanti et al., 2019a). In a periodontitis mouse model induced by P. gingivalis administration, fabricated gel promoted osteoprotegerin (OPG) expression. Additionally, the fabricated gel increased osteoblast number and decreased osteoclast number at the alveolar mandible (Mulawarmanti et al., 2019b).
One study extracted sulfated polysaccharides from Gracilaria caudate, one of Rhodophyta, and evaluated their preventive effect on tissue damage caused by periodontitis (da Silva et al., 2019). G. caudate-derived sulfated polysaccharides suppressed gingival bleeding index (GBI), probing pocket depth (PPD), and myeloperoxidase (MPO) activity in gingiva and decreased MDA concentration and glutathione (GSH) levels in the liver in a ligature-induced periodontitis rat model. Additionally, sulfated polysaccharides prevent the histopathological alteration, which is induced by periodontitis.
Two studies demonstrated that ECE exhibits a therapeutic effect on periodontitis (Jung et al., 2021; Kim et al., 2019b). In a ligature-induced periodontitis rat model, ECE decreased several clinical symptoms, including ABL, bone resorption, the distance between the cementoenamel junction (CEJ) and the crest of the alveolar bone (ABC), gingival index (GI), periodontal bone height, and tooth mobility (TM). Additionally, ECE inhibited the proliferation and erosion of periodontal epithelial cells and suppressed the mRNA expression of IL-1β, MMP-2, MMP-9, and RANKL/OPG.
A 2020 study has isolated bromoageliferin from A. dilatata and assessed its efficacy against periodontitis-related microbial, such as Acinetobacter baumannii, K. pneumoniae, and P. aeruginosa (Pech-Puch et al., 2020). Bromoageliferin showed an anti-survival effect on P. aeruginosa in the P. aeruginosa-infected Galleria mellonella model.
Two studies using M101 as a therapeutic agent for periodontitis have been conducted (Batool et al., 2020; Batool et al., 2022). In one of these studies, M101 decreased clinical abscess size, inflammatory cell infiltration, inflammatory score, and TRAP-positive cell number and promoted the quantity and quality of bone in a P. gingivalis-induced subcutaneous calvarial abscess mice model (Batool et al., 2022). In P. gingivalis soaked ligature-induced periodontitis mouse model, M101 showed augmentation in fibrous connective tissue attachment (FA) and suppressed epithelial attachment (EA) (Batool et al., 2020). Additionally, M101 enhanced the attachment level (AL) and bone contour and quality. In histological analysis, M101 suppressed the expression of HIF-1α, NF-κB, and MMP-9 and the number of TRAP-positive cells at the periodontitis-induced site.
A 2020 study investigated whether fish oil has the potential for periodontitis treatment in a hypercholesterolemic rat model with ligature-induced periodontitis (Antona et al., 2020). Fish oil prevents bone loss and promotes bone trabeculae, the substitution of saturated fat, periodontal bone support (PBS), and a percentage of lower bone volume (LBV) and total lower volume (TLV).
Nannochloropsis oculata, one of the green microalgae, has been explored for functional food, nutraceutical, pharmacological agents, and aquaculture feed application (Andriopoulos et al., 2022; Hussein et al., 2020; Samarakoon et al., 2013). Therefore, Revianti et al. investigated whether N. oculata extract shows a pharmacological effect on periodontitis using an A. actinomycetemcomitans-infected periodontitis rat model (Revianti et al., 2020). Oral irrigation with N. oculata extracts decreased TNF-α expression and enhanced expression of OPG and IL-10 in periodontal ligament tissue.
One study demonstrated that Spirulina maxima extract has the potential for periodontitis treatment (Kang et al., 2021). S. maxima extract suppressed MPO activity, decreased TRAP-positive cell number in the destroyed space of the bone surface, and shorten the distance between CEJ and ABC in P. gingivalis-inoculated periodontitis rat model. Additionally, S. maxima extract increased OPG/RANKL expression ratio and osteoblast number in the destroyed space of the bone surface and inhibited mRNA expression of pro-inflammatory cytokines (TNF-α, IL-1β, IL-4, IL-6, and NF-κB), MMP-8, and MMP-13.
Two studies assessed the therapeutic effect of omega-3 polyunsaturated fatty acids on periodontitis using a ligature-induced periodontitis rat model (Jalal et al., 2020a; Jalal et al., 2020b). Omega-3 polyunsaturated fatty acids inhibited serum levels of IL-1β, IL-6, and TNF-α, decreased inflammatory cell infiltration and periodontal damage score, and enhanced bone formation at incisor periodontal tissue.
Three studies used chitosan as a base material for drug delivery systems for periodontitis treatment (Gani et al., 2022; Guo et al., 2021; Li et al., 2021). Ginsenoside Rg1 is a component of the natural extract of ginseng and is reported to its anti-inflammatory activities (Gao et al., 2020). Amelogenin is the main component of enamel matrix proteins and exhibits a regenerative effect on periodontal tissue (Liao et al., 2020). To deliver two drugs (Ginsenoside Rg1 and amelogenin) to periodontal tissue, one study fabricated Ginsenoside Rg1 and amelogenin-loaded injectable hydrogel composed of glycol chitosan and aldehyde-modified hyaluronic acid (1% HA-CHO + 1% GC + FeCl3) and evaluated the periodontium regenerative activity using rat model induced by periodontium defect (Guo et al., 2021). 1% HA-CHO + 1% GC + FeCl3 hydrogels nearly completed periodontium regeneration in the periodontium defect site and decreased clinical symptoms, such as the distance between CEJ and ABC, bone mineral density (BMD), bone volume fraction, trabecular thickness (Tb. Th), infiltration of inflammatory cells, and optical density of IL-1, TNF-α, and TGF-β. Another study developed a chitosan/cyclodextrin drug delivery system loaded with minocycline, which can inhibit the biofilm formation of Streptococcus gordonii and P. gingivalis (Elnagdy et al., 2021; Li et al., 2021). The developed drug delivery system alleviated several histological changes, including GI, TM, the distance between CEG and ABC, proliferation and erosion of periodontal epithelial cells, and bone resorption. Additionally, the system suppressed the mRNA expression of IL-1β, MMP-2, MMP-9, and RANKL/OPG ratio. The other study investigated whether hydroxyapatite-loaded chitosan gel as a bone graft has a therapeutic effect on periodontitis in a femoral defect rat model (Gani et al., 2022). Chitosan gel enhanced Bone morphogenetic protein (BMP)-2 expression and inhibited IL-1 expression at defect sites.
Few clinical studies (n = 8) have revealed that several MBS can be prevent and treat periodontitis (Deshpande et al., 2021, dos Santos et al., 2020; Hussain et al., 2022; Kujur et al., 2020; Murgia & Aspriello, 2021; Poornima et al., 2021; Pușcașu et al., 2022; Stańdo et al., 2020).
It was conducted two studies that used Chordata as an extraction source of base material or pharmacological agents (Deshpande et al., 2021; Pușcașu et al., 2022). Forty patients with chronic periodontitis were examined to investigate the therapeutic effects of the grafts with nanocrystalline hydroxyapatite in the absence and presence of fish COL (Deshpande et al., 2021). Through clinical parameter analysis, they confirmed that both grafts reduced plaque index (PI), GI, PPD, clinical attachment level (CAL), and radiographic defect depth, but no statistically significant difference between the two groups was observed. Another study demonstrated the potential of marine fish extracts (Alflutop®), which is known as extracts of Engraulis encrassicholusponticus, Sprattus sprattus sprattus, and Rizeafca nordmanni, for periodontitis treatment (Pușcașu et al., 2022). 44 patients with periodontitis received Alflutop® treatment after scaling, root planning, and debridement (SRP) and 44 patients with periodontitis received only SRP. Alflutop® treatment after SRP decreased GI of the Score 2 patients and alleviated the papilla bleeding index (PBI) and pathological TM of the Score 1 patients without side effects. The degree of TM and probing depth (PD) of all patients was decreased by Alflutop® treatment.
Almost clinical studies have evaluated the therapeutic effect of commercial products, such as omega-3 fatty acids, chitosan-based varnish, chitosan brush, COL-loaded composite, and Alfalife (omega-3 fatty acids) (dos Santos et al., 2020; Hussain et al., 2022; Kujur et al., 2020; Murgia & Aspriello, 2021, Poornima et al., 2021; Stańdo et al., 2020).
Four studies investigated the therapeutic effect of omega-3 fatty acids on patients with periodontitis (dos Santos et al., 2020; Kujur et al., 2020; Murgia & Aspriello, 2021; Stańdo et al., 2020). The results of three studies indicated that omega-3 fatty acids showed no adverse events and no changes in systemic parameters, including body mass index, blood pressure, levels of glycated hemoglobin A1c and triglycerides, in patients with periodontitis (dos Santos et al., 2020; Kujur et al., 2020; Stańdo et al., 2020). Additionally, omega-3 fatty acids decreased several indexes, such as Bleeding on probing (BoP), CAL, GI, PD, PI, PPD, and gingival recession (REC), the concentration of inflammatory mediators (IL-1β, IL-6, IL-8, IL-17, and IFN-γ), and release of chemokine (C-C motif) ligand (CCL)21, CCL26, CCL27, chemokine (C-X-C motif) ligand (CXCL)10, and CXCL16. In contrast, the treatment of omega-3 fatty acids increased the concentration of anti-inflammatory cytokine (IL-10), fibroblast growth factor (FGF)-2, and granulocyte-colony stimulating factor (G-CSF) and enhanced the release of CC4, CCL5, CCL15, CCL25, and chemokine (C-X3-C motif) ligand (CX3CL) 1. Another study investigated that Alfalife, a products containing omega-3 and omega-6 dietary supplements, has an anti-periodontitis effect in patients with periodontitis (Murgia & Aspriello, 2021). Alfalife decreased BoP, CAL, and PD. Based on these results, omega-3 fatty acids have a therapeutic potential for periodontitis through an anti-inflammatory effect.
Chitosan is a natural alkaline polysaccharide obtained from chitin via deacetylation and has antibacterial, anti-cancer, and antiviral activities, biocompatibility, and biodegradability (Ke et al., 2021; Wang et al., 2020a). Therefore, many studies have used chitosan as base materials for biomedical applications, such as drug delivery systems, hydrogels, nanofibers, and scaffolds (Cui et al., 2021; Peers et al., 2020; Rajabi et al., 2021; Sadeghianmaryan et al., 2020). For these reasons, two studies investigated the therapeutic effect of chitosan brush in patients undergoing fixed orthodontic therapy and in periodontitis patients (Hussain et al., 2022; Poornima et al., 2021). In patients undergoing fixed orthodontic therapy, chitosan brush showed no adverse events and decreased plaque bacterial count (Poornima et al., 2021). In patients with stage III and IV, grade B periodontitis, patients underwent no adverse events and decreased bleeding on probing (BoP) and PPD (Hussain et al., 2022).
Publication year | Kind of sample | Sample | Kind of source | Organisms | Stimulator | Cell | Outcomes | Ref |
---|---|---|---|---|---|---|---|---|
2019 | Application | S. persica extract-loaded COL hybrid constructs | Cnidaria | Rhizostoma pulmo | - | HPLF | ↑Cell proliferation | Arslan & Kantarcioğlu, 2019 |
2019 | Extract | ECE | Phaeophyceae | Ecklonia cava | Pg LPS | RAW 264.7 |
Non cytotoxicity ↓ NO and PGE2 production ↓ COX-2 activity ↓ mRNA expression of IL-1β, IL-6, iNOS, and TNF-α ↑ Activation of Nrf-2/HO-1 pathway ↓ Phosphorylation of NF-κB pathway |
Kim et al., 2019b |
2019 | Compound | Marine bromophenol & extract | Rhodophyta | Kappaphycus alvarezii | - | - |
Antibacterial effect on Porphyromonas gingivalis ↓ Rgp activity ↓ Hemagglutination ↓ mRNA expression of HgA, PPAD, and Rgp |
Cherian et al., 2019 |
2020 | Application | Chitosan-loaded gel | Chordata | Chanos chanos | - | - | Antibacterial effect on P. gingivalis and Aggregatibacter actinomycetemcomitan | Achmad et al., 2020a |
2020 | Application | COL-sodium alginate-TiO2 matrix | Chordata | Tilapia | - | HPLF |
Non cytotoxicity ↑Level of ALP and OCN ↑Mineralization ↑mRNA expression of ALP, COL, OCN, and RUNX-2 ↑Protein expression of COL, OCN, and RUNX-2 |
Elango et al., 2020 |
2020 | Application | Dox:CS/CMCS-NPs | Unknown | Unknown | Pg LPS & ATP | HGF |
Antibacterial effect on P. gingivalis Non cytotoxicity ↓ mRNA and protein expression of ASC and NLRP3 ↓ mRNA and protein expression of IL-1β ↓ mRNA expression and activation of Caspase-1 |
Xu et al., 2020 |
2020 | Application | PVA/COL hydrogel | Chordata | Tilapia | - | HPLF & HGF |
↑Cell viability and proliferation when increased COL ratio in both cell lines ↑Cell adhesion when increased COL ratio in both cell lines |
Zhou et al., 2020 |
2020 | Application | Resveratrol and Celastrol-loaded fish COL/chitosan film |
Combination (Chordata & unknown) |
Tilapia & unknown | - | HPLF & BMM |
↑Proliferation of HPLF ↑Differentiation to TRAP-positive cell from BMM |
Wang et al., 2020a |
2020 | Application | Surfactin-loaded ĸ-carrageenan oligosaccharides entangled cellulose nanofibers | Unknown | Unknown | - | RAW 264.7 |
Non cytotoxicity Antibacterial effect on P. gingivalis Anti-biofilm effect of P. gingivalis and Streptococcus mutans ↓ Metabolic activity of P. gingivalis and S. mutans ↑MDA level on P. gingivalis and S. mutans ↑Cell death of P. gingivalis and S. mutans |
Johnson et al., 2020 |
2020 | Compound | Alkaloids | Porifera | Agelas dilatata | - | - |
Antibacterial effect on P. aeruginosa Anti-biofilm effect on P. aeruginosa |
Pech-Puch et al., 2020 |
2020 | Compound | Biseokeaniamide A | Cyanobacteria | Okeania sp. | LPS | RAW 264.7 |
Non cytotoxicity ↓ NO production ↓ Expression of IL-1β ↓ Protein expression of iNOS ↓ Protein expression of IκBα |
Ohno et al., 2020 |
2020 | Compound | Cadex2870 | Pseudomonadota | Catenovulum sp. | - | - |
Anti-biofilm effect on S. mutans Scavenging effect on the formed plaque biofilm of S. mutans |
Deng et al., 2020 |
2020 | Compound | DHA & EPA | Unkonwn | Unkonwn | - | - |
Antibacterial effect on Streptococcus oralis, Actinomyces naeslundii, Veillonella parvula, Fusobacterium nucleatum, P. gingivalis, and Aggregatibacter actinomycetemcomitans Anti-biofilm effect on Streptococcus sp. and Aggregatibacter sp. |
Ribeiro-Vidal et al., 2020 |
2020 | Compound | M101 | Annelida | Arenicola marina | Pg LPS stimulation or P. gingivalis infection | HOEC |
Antibacterial effect on P. gingivalis Non cytotoxicity ↓ mRNA expression of NF-κB, TNF-α, and RANKL ↓ Protein expression of TNF-α ↓ Protein expression of IL-1β, IL-8, IP-10, and RANTES ↑Protein expression of IL-10, IL-2, IL-4, IL-11, IL-15, PDGF-BB, and TGF-β1 ↑Release of ICAM-1, M-CSF, and TIMP-2 ↑mRNA expression of Resolvin-E1 receptor |
Batool et al., 2020 |
2020 | Compound | 2,2-bis(6-bromo-1H-indol-3-yl) ethanamine & its fluorinated analogue | Unknown | Unknown | - | - |
Antimicrobial activity on S. mutans Antibacterial activity against S. mutans in plasma Anti-biofilm effect against S. mutans Inducted VBNC ↓ Oxacillin resistance in S. mutans |
Campana et al., 2020 |
2020 | Extract |
Algae EtOH extracts (ATEE, DDEE, GFEE, HJEE, MSEE, & SCEE) |
Rhodophyta & Phaeophyceae | ATEE, DDEE, GFEE, HJEE, MSEE & SCEE | Pg LPS | HGF |
Non cytotoxicity ↓ NO production (ATEE, HJEE, GFEE, and SCEE) ↓ Protein expression of iNOS (ATEE and HJEE) |
Yoon et al., 2020 |
2021 | Application | Atorvastatin solid-loaded gel formulation | Unknown | Unknown | TNF-α | HGF | ↓ Release of IL-1β, IL-6, IL-8, IL-10, TGF-β1, TGF-β2, and TGF-β3 | Özdoğan et al., 2021 |
2021 | Application | Ginsenoside Rg1 and amelogenin-loaded injectable glycol chitosan/aldehyde-modified hyaluronic acid hydrogel | Unknown | Unknown | - | HBMSC | Non cytotoxicity | Guo et al., 2021 |
2021 | Application | Minocycline-loaded chitosan/cyclodextrin mouthwash | Unknown | Unknown | - | - | Antibacterial effect on P. gingivalis Anti-biofilm effect on P. gingivalis | Li et al., 2021 |
2021 | Application | Xn2%-HA1%-M101 gels | Annelida | A. marina | - | HOEC |
Non cytotoxicity Antibacterial effect on P. gingivalis |
Özçelik et al., 2021 |
2021 | Extract | Agarum clathratum MeOH extract | Phaeophyceae | A. clathratum | Pg LPS | THP-1 |
Non cytotoxicity ↓ mRNA and protein expression of IL-1β |
Han et al., 2021 |
2021 | Extract | ECE | Phaeophyceae | E. cava | LPS | HGF |
Non cytotoxicity ↓ PGE2 production ↓ ROS production ↓ Phosphorylation of MAPK pathway ↓ mRNA expression of COX-2, IL-6, MMP-2, and MMP-8 ↓ mRNA expression of MIP-1α and SDF-1 |
Jung et al., 2021 |
2021 | Extract | KAE, KAEA, KAC | Rhodophyta | K. alvarezii | - | Vero & KB-3-1 |
High antioxidant activity Antimicrobial activity on Bacillus subtilis, Escherichia coli, Klebsiella pneumonia, Pseudomonas aeruginosa and Candida albicans High cell viability on Vero (KAC) High cytotoxicity on KB-3-1 (KAE) |
Sharan & Vennila, 2021 |
2021 | Extract | Squid skin liposome | Mollusca | Dosidicus gigas | RANKL/M-CSF | RAW 264.7 |
Non cytotoxicity ↓ PGE2 level ↓ Osteoclast maturation ↓ Bone resorption pit area ↓ TRAP activity ↓ mRNA expression of NFATc1, c-Src, Cathepsin K, DC-STAMP RANK, and TRAP |
Kao et al., 2021 |
2021 | Compound | EPA | Unknown | Unknown | Pg LPS or heat-inactivated Pg LPS | HGF |
Non cytotoxicity ↓ mRNA expression of IL-1β and IL-6 ↓ Protein expression of IL-6 ↑mRNA and protein expression of HO-1 |
Ke-cong et al., 2021 |
2021 | Compound | Epiloliolide | Phaeophyceae | Sargassum horneri | Pg LPS | HPDL |
↑Proliferation, migration, mineralization ↓ Protein expression of NLRP3, COX-2, and iNOS ↑Phosphorylation of PKA/CREB pathway ↑mRNA expression of ALP, OPN, and RUNX-2 ↓ mRNA expression of IL-1β, IL-6, and TNF-α ↓ Release of IL-1β, IL-6, and TNF-α |
Kim et al., 2021a |
2022 | Application | FDCD | Unknown | Unknown | - | MC3T3-E1 & RAW 264.7 |
Antibacterial effect on Enterococcus faecalis Anti-biofilm effect on E. faecalis Induction of cell leakage Non cytotoxicity ↑ROS production inside bacterial cell ↑Macrophage migration |
Tang et al., 2022 |
2022 | Application | Jellyfish COL scaffolds | Cnidaria | Aurelia aurita | - | hMSC | ↑Cell proliferation | Rachmawati et al., 2021 |
2022 | Compound | M101 | Annelida | A. marina | P. gingivalis at MOI 100 or inducing hypoxia with CoCl2 | HOEC |
Antioxidant effect Anti-hypoxic effect ↑Cell migration ↓ mRNA expression of GLUT-1, GLUT-3, HIF-1α, and MMP-9 |
Batool et al., 2022 |
2022 | Extract | Turbinariaconoides PE extract | Phaeophyceae | T. conoides | - | - | Antibacterial effect on Streptococcus sp. and Actinomycetes sp. | Magesh et al., 2022 |
2022 | Extract | Sargassum Wightii PE extract | Phaeophyceae | S. Wightii | - | - | Antibacterial effect on S. mutans | Magesh et al., 2020 |
HPLF, human periodontal ligament fibroblast; ECE, Ecklonia cava ethanol extract; Pg LPS, Porphyromonas gingivalis lipopolysaccharide; NO, nitric oxide; PGE2, prostaglandin E2; COX-2, cyclooxygenase-2; IL, interleukin; iNOS, inducible nitric oxygen synthase; TNF-α, tumor necrosis factor alpha; HO-1, heme oxygenase-1; Rgp, Arginine specific gingipain; HgA, hemagglutinin A; PPAD, peptidyl arginine deiminase; ALP, alkaline phosphatase; OCN, osteocalcin; COL, collagen; RUNX-2, runt-related transcription factor 2; Dox:CS/CMCS-NPs, doxycycline-containing chitosan/carboxymethyl chitosan nanoparticles; ATP, adenosine triphosphate; HGF, human gingival fibroblast; ASC, apoptosis-associated speck-like protein containing a caspase recruitment domain; NLRP3, NOD-like receptor pyrin domain-containing protein 3; PVA, poly (vinyl alcohol); BMM, bone marrow-derived macrophages; TRAP, tartrate resistant acid phosphatase; MDA, malondialdehyde; IκBα, nuclear factor of kappa light polypeptide gene enhancer in B-cells inhibitor, alpha; DHA, docosahexaenoic acid; EPA, eicosapentaenoic acid; HOEC, human oral epithelial cells; NF-κB, nuclear factor kappa-light-chain-enhancer of activated B cells; RANKL, receptor activator of nuclear factors κB ligand; RANTES, regulated upon activation, normal T cell expressed and presumably secreted; PDGF-BB, platelet-derived growth factor-BB; TGF, transforming growth factor; ICAM-1, intercellular adhesion molecule 1; M-CSF, macrophage colony-stimulating factor; TIMP, tissue inhibitors of matrix metalloproteinases; VBNC, viable but non-culturable cells; EtOH, ethanol; ATEE, Asparagopsis taxiformis; DDEE, Distromium decumbens; GFEE, Galaxaura fastigiata; HJEE, Hypnea japonicay; MSEE, Myelophycus simplex; SCEE, Scytosiphon canaliculatus; HBMSC, human bone marrow mesenchymal cell; MeOH, methanol; THP-1, human monocytic leukemia cell; ROS, reactive oxygen species; MMP, matrix metalloproteinase; MIP-1α, macrophage inflammatory protein-1 alpha; SDF, stromal cell-derived factor; KAE, Kappaphycus alvarezii extracts ethanol extract; KAEA, Kappaphycus alvarezii extracts ethyl acetate extract; KAC, Kappaphycus alvarezii extracts chloroform extract; DC-STAMP, dendritic cell specific transmembrane protein; HPDL, human periodontal derived ligament cells; PKA, protein kinase A; CREB, cAMP response element-binding protein; OPN, osteopontin; FDCD, fucoidan-derived carbon dots; hMSC, human mesenchymal stem cell; GLUT, glucose transporters; HIF, hypoxia inducible factor; PE, petroleum ether.
Publication year | Kind of sample | Sample | Kind of source | Organisms | Model | Outcomes | Ref |
---|---|---|---|---|---|---|---|
2019 | Application | CMC-Na gel containing Stichopus herrmanni powder | Echinodermata | S. herrmanni | Periodontitis diabetes rat model induced by Porphyromonas gingivalis administration | ↓ Expression of IL-1β and IL-10 | Mulawarmanti et al., 2019a |
2019 | Application | CMC-Na gel containing S. herrmanni powder | Echinodermata | S. herrmanni | Periodontitis mice model induced by P. gingivalis administration |
↑OPG expression ↑Number of osteoblast ↓ Number of osteoclast |
Mulawarmanti et al., 2019b |
2019 | Extract | Sulfated polysaccharides | Rhodophyta | Gracilaria caudata | Ligature-induced periodontitis rat model |
↓ GBI and PPD ↓ MPO activity ↓ MDA concentration and GSH level in liver ↓ Histopathological alteration in liver tissue |
da Silva et al., 2019 |
2019 | Extract | ECE | Phaeophyceae | Ecklonia cava | Ligature-induced periodontitis rat model |
↓ GI and TM ↓ Distance between CEJ and ABC ↓ Proliferation and erosion of periodontal epithelial cell ↓ Bone resorption ↓ mRNA expression of IL-1β, MMP-2, MMP-9, and RANKL/OPG |
Kim et al., 2019b |
2020 | Compound | Bromoageliferin | Porifera | Agelas dilatata | Pseudomonas aeruginosa-infected Galleria mellonella model | Anti-survival effect | Pech-Puch et al., 2020 |
2020 | Compound | M101 | Annelida | Arenicola marina | P. gingivalis-induced subcutaneous calvarial abscess mice model |
↓ Clinical abscess size ↓ Inflammatory cell infiltration ↓ Inflammatory score ↑Quantity and quality of bone ↓ TRAP-positive cell number |
Batool et al., 2020 |
2020 | Compound | Omega-3 polyunsaturated fatty acid | Unknown | Unknown | Ligature-induced periodontitis rat model | ↓ Serum level of IL-1β, IL-6, and TNF-α | Jalal et al., 2020a |
2020 | Compound | Omega -3 polyunsaturated fatty acid | Unknown | Unknown | Ligature-induced periodontitis rat model |
↑Bone formation ↓ Inflammatory cell infiltration ↓ Periodontal damage score |
Jalal et al., 2020b |
2020 | Extract | Fish oil | Chordata | Unknown | Hypercholesterolemic rat model with ligature-induced periodontitis |
↓ Bone loss ↑Bone trabeculae ↑LBV/TLV% ↑Substitution of saturated fat ↑PBS |
Antona et al., 2020 |
2020 | Extract | Nannochloropsis oculata extract | Ochrophyta | N. oculata | A. actinomycetemcomitans infected periodontitis rat model |
↓ TNF-α expression ↑Expression of OPG and IL-10 |
Revianti et al., 2020 |
2021 | Application | Ginsenoside Rg1 and amelogenin-loaded injectable glycol chitosan/aldehyde-modified hyaluronic acid hydrogel | Unknown | Unknown | Periodontitis rat model induced by periodontium defect |
Nearly completed periodontium regeneration ↓ Distance between CEJ and ABC ↓ BMD, bone volume fraction (BV/TV), and Tb. Th ↓ Infiltration of inflammatory cells ↓ Optical density of IL-1, TGF-β, and TNF-α |
Guo et al., 2021 |
2021 | Application | Minocycline-loaded chitosan/cyclodextrin mouthwash | Unknown | Unknown | Ligature-induced periodontitis rat model |
Antiplaque biofilm activity ↓ GBI and ABL ↓ Distance between CEJ and ABC |
Li et al., 2021 |
2021 | Extract | ECE | Phaeophyceae | E. cava | Ligature-induced periodontitis rat model |
↓ ABL and bone height ↓ mRNA expression of RANKL/OPG |
Jung et al., 2021 |
2021 | Extract | Spirulina maxima extract | Cyanophyta | S. maxima | P. gingivalis inoculated periodontitis rat model |
↓ MPO activity ↓ TRAP-positive cell number ↑OPG/RANKL expression ratio ↓ Distance between CEJ and ABC ↑Osteoblast number ↓ mRNA expression of IL-1β, IL-4, IL-6, NF-κB, and TNF-α ↓ mRNA expression of MMP-8 and MMP-13 ↑Protein expression OPN, OSX, and Type I COL ↑Activation of BMP-2/Smad pathway |
Kang et al., 2021 |
2022 | Application | Hydroxyapatite-loaded chitosan gel | Unknown | Unknown | Femoral defect rat model |
↑BMP-2 expression ↓ IL-1 expression |
Gani et al., 2022 |
2022 | Compound | M101 | Annelida | A. marina | P. gingivalis soaked ligature-induced periodontitis mice model |
Augmentation in the FA ↓ EA ↑AL and bone contour and quality ↓ Expression of HIF-1α, NF-κB, and MMP-9 ↓ Number of TRAP positive cell |
Batool et al., 2022 |
2022 | Extract | S. herrmanni extract | Echinodermata | S. herrmanni | Femoral defect guinea pig model |
↑OCN expression ↑Number of osteoblast |
Adam et al., 2022 |
CMC-Na, sodium carboxymethylcellulose; OPG, osteoprotegerin; ECE, Ecklonia cava ethanol extract; GBI, gingival bleeding index; PPD, probing pocket depth; MPO, myeloperoxidase; MDA, malondialdehyde; GSH, glutathione; GI, gingival index; TM, tooth mobility; CEJ, cementoenamel junction; ABC, the crest of the alveolar bone; IL, interleukin; MMP, matrix metalloproteinase; RANKL, receptor activator of nuclear factors κB ligand; TRAP, tartrate resistant acid phosphatase; TNF-α, tumor necrosis factor alpha; LBV, lower bone volume; TLV, total lower volume; PBS, periodontal bone support; BMD, bone mineral density; BV, bone volume; TV, tissue volume; Tb. Th, trabecular thickness; TGF, transforming growth factor; ABL, alveolar bone loss; OPN, osteopontin; OSX, osterix; COL, collagen; BMP, bone morphogenetic protein; FA, fibrous connective tissue attachment; EA, epithelial attachment; AL, attachment level; HIF, hypoxia inducible factor; NF-κB, nuclear factor kappa-light-chain-enhancer of activated B cells.
Publication year | Kind of sample | Sample | Kind of source | Organisms | Patient | Outcomes | Ref |
---|---|---|---|---|---|---|---|
2020 | Compound | Omega-3 fatty acids | Unknown | Unknown |
Periodontitis patient (n = 1, female) |
No changes in systemic parameters ↓ BoP, CAL, PD, and PI ↓ Concentration of IFN-γ, IL-1β, and IL-6 in gingival crevicular fluid |
dos Santos et al., 2020 |
2020 | Compound | Omega-3 fatty acids | Unknown | Unknown |
Periodontitis patients (n = 30, both) |
No adverse events ↓ BoP, CAL, PD, and REC ↓ Concentration of IL-8 and IL-17 ↑Concentration of IL-10 ↑Release of CCL4, CCL5, CCL15, CCL25 and CX3CL1 ↓ Release of CCL21, CCL26, CCL27, CXCL1, CXCL10 and CXCL16 ↑Concentration of FGF2 and G-CSF |
Stańdo et al., 2020 |
2020 | Compound | Omega-3 fatty acids | Unknown | Unknown | Patients with chronic generalised moderate periodontitis (n = 76, both) | ↓ PPD, CAL, and GI | Kujur et al., 2020 |
2021 | Application | Chitosan brush | Unknown | Unknown | Patients undergoing fixed orthodontic therapy (n = 20, both) |
No adverse events ↓ Plaque bacterial count |
Poornima et al., 2021 |
2021 | Application | COL-loaded composite | Chordata | Unknown | Periodontitis patients (n = 40, both) |
↓ PI, GI, PPD, and CAL ↓ Radiographic defect depth |
Deshpande et al., 2021 |
2021 | Compound | Alfalife | Unknown | Unknown | Periodontitis patients (n = 24, both) | ↓ BoP, CAL, and PD | Murgia & Aspriello, 2021 |
2022 | Application | Chitosan brush | Unknown | Unknown | Patients with stage III and IV, grade B periodontitis (n = 78, both) |
No adverse event ↓ BoP and PPD |
Hussain et al., 2022 |
2022 | Extract | Alflutop® treatment after SRP | Chordata | Engraulis encrassicholus ponticus, Sprattus sprattus sprattus, & Rizeafca nordmanni | Periodontitis patients (n = 88, both) |
No side effects ↓ GI of the score 2 patients ↓ PBI of the score 1 patients ↓ Pathological TM of the score 1 patients ↓ Degree of TM and PD |
Pușcașu et al., 2022 |
BoP, bleeding on probing; CAL, clinical attachment level; PD, probing depth; PI, plaque index; IL, interleukin; REC, gingival recession; CCL, chemokine (C-C motif) ligand; CX3CL, chemokine (C-X3-C motif) ligand; CXCL, chemokine (C-X-C motif) ligand; FGF, fibroblast growth factor; G-CSF, granulocyte-colony stimulating factor; PPD, probing pocket depth; GI, gingival index; SRP, scaling, root planning, and debridement; PBI, papilla bleeding index; TM, tooth mobility.
Discussion and Conclusion
All studies (56 articles), which were published from January 2019 to July 2022 were analyzed and summarized literature content about the therapeutic effects and application forms of MBS for periodontitis.
Analysis result of the published year indicated that publication numbers steadily is increasing from January 2019. Most studies used commercial MBS obtained from material companies. However, as illustrated in Fig. 3A, MBS against periodontitis treatment, which in described information of marine organisms, was mainly extracted from Phaeophyceae, Chordata, and Annelida. Additionally, MBS was investigated in the form of extracts, compounds, and applications (e.g., brush, film, hydrogel, mouthwash, nanofiber, and nanoparticle) at a similar percentage during the analyzed period (Fig. 3C).
Biomedical engineering is the application of engineering principles and problem-solving techniques to medical and biological research for healthcare that provides new strategies for the diagnosis, prevention, and treatment of various human diseases (Kim & Jung, 2019; Kim et al., 2021a; Oh et al., 2021). The main goal of biomedical engineering is the development of new therapies and technologies to help, recover, and replace damaged tissue and organs without side effects (Chandika et al., 2021; Lee et al., 2020; Oh et al., 2020). Although numerous biomedical applications incorporating MBS have been investigated, most studies investigated the regenerative effect of MBS on skin and bone (Chandika et al., 2021; Kim & Jung, 2019; Kim et al., 2021a; Lee et al., 2020; Oh et al., 2020; Oh et al., 2021). In this review, only 18 studies developed several biomedical applications, including film, hydrogel, nanofiber, and nanoparticles (Achmad et al., 2020a; Arslan & Kantarcioğlu, 2019; Deshpande et al., 2021; Elango et al., 2020; Gani et al., 2022; Guo et al., 2021; Hussain et al., 2022; Johnson et al., 2020; Mulawarmanti et al., 2019a; Mulawarmanti et al., 2019b; Özçelik et al., 2021; Özdoğan et al., 2021; Poornima et al., 2021; Rachmawati et al., 2021; Tang et al., 2022; Wang et al., 2020a; Xu et al., 2020; Zhou et al., 2020). The biomedical application for periodontitis is steadily investigated from 2019 to 2022. Therefore, we suggest that biomedical engineering incorporating MBS for periodontitis has a high potential as a therapeutic strategy.
Although MBS has high therapeutic potential on periodontitis, several limitations and weaknesses remain in terms of extraction source, underlying mechanism from in vitro and in vivo, and few results in clinical safety and efficacy. Firstly, although the ocean covers more than 70% of the earth embedding numerous marine organisms with biological agents, only a few marine organisms were used as extraction sources of bioactive substances for periodontitis treatment (Kim et al., 2019a). Next, most studies used commercial products of MBS, not extracted MBS. Thus, it is hard to confirm the specific information of extraction sources and active compounds. The underlying mechanisms from in vitro and in vivo of MBS as therapeutic agents for periodontitis were demonstrated by only seven articles (12.5% of the number of articles about MBS for periodontitis treatment) (Batool et al., 2020; Batool et al., 2022; Kang et al., 2021; Kao et al., 2021; Kim et al., 2019b; Kim et al., 2021a; Ohno et al., 2020). Finally, few clinical studies (8 articles) were conducted to confirm the safety and therapeutic efficacy of MBS.
Therefore, additional research is needed to develop more numerous extraction sources such as starfish, algae, and unstudied marine organisms, and isolate single compounds to apply medical devices. Clinical studies on the safety and therapeutic efficacy of MBS for periodontitis treatment are needed to demonstrate the therapeutic potential and safety for periodontitis patients. Finally, biomedical applications incorporating MBS for periodontitis must be constantly developed to enhance the therapeutic efficacy of MBS for periodontitis.