Introduction
In recent years marine organisms have attracted a lot of attention and have been commercially produced for industrial use. Its various potentials can be utilized in various industrial applications for human benefit (Baptista et al., 2020; Puspita et al., 2020). Marine organisms such as algae, sponges, and corals produce various compounds with diverse bioactivity and are potential sources of new therapeutic agents. For example, seaweed has been used as a therapeutic and traditional medicine in several countries such as China and Korea (Kang et al., 2016; Sanjeewa & Jeon, 2018).
Marine organisms can survive in complex communities and competitive environments by producing various secondary metabolites in response to environmental stresses such as competition, predators, and tidal variations (Abu-Ghannam & Rajauria, 2013). Some of these compounds function as antimicrobials to interfere with the growth of competitive microorganisms (Kasmiati et al., 2018; Pérez et al., 2016; Silva et al., 2020). Compared to other marine organisms, seaweed can play an important role in many ways. Macroalgae or seaweeds have developed physiological adaptations including synthesizing active compounds as a defense against epiphytes and other organisms fouling (Águila–Ramírez et al., 2012; Bhadury & Wright, 2004).
Several studies suggest that in crude extracts, fractionated parts and/or pure compounds of green, brown, and red seaweeds exhibit antioxidant activity (Corsetto et al., 2020), anticoagulant (Wijesinghe et al., 2011), antiviral (Cheung et al., 2014), antifungal (De Corato et al., 2017), anticancer (Gutiérrez-Rodríguez et al., 2018), cytotoxic (Gunasekaran et al., 2017), anti-inflammatory (Kazłowska et al., 2010), antibacterial (Agbaje-Daniels et al., 2017), and toxicity (Kim & Choi, 2017).
Recently, seaweed has been increasingly studied because of its potential to produce new compounds with various biological activities that are important for solving food and health problems (Brown et al., 2014). One of the challenges in recent years, microorganisms have developed a new strategy so that they are resistant to the action of antibiotics known as multiple drug-resistant bacterial strains (Hampton et al., 2020). Common antibiotics have lost most of their efficacy. Thus, it is urgently needed to continue to find new antimicrobial drugs that can address the never-ending problem of antimicrobial resistance. Antimicrobial resistance has become an important threat to human and animal health and the environment (Reed et al., 2019). The increasing resistance of pathogens to antibiotics has inspired experts to intensively explore and develop cheaper and more effective natural antimicrobial agents with better potency. It is a priority in the field of public health to find new antimicrobials with fewer side effects than antibiotics, good bio-resource availability, and minimal toxicity (Thanigaivel et al., 2015).
Diverse studies have proven that seaweed is a source of strong antibacterial agents such as Ulva rigida (Ismail et al., 2018; Sirakov et al., 2019); Caulerpa racemosa and Caulerpa lentilifera (Nagappan & Vairappan, 2014). Thus, it is also possible to examine the possibility of synergizing antimicrobial compounds from marine organisms with currently available drugs (Cheung et al., 2014). In addition to the promising antibacterial activity of seaweed, it is also necessary to explore the activity toxicity, because it is very important for a compound to be non-toxic and have a promising effect at the same time.
In Indonesia, one of the marine algae species that is currently considered cheap and underutilized is red seaweed of the genus Halymenia. Even though this type of algae has wide potential for use, especially its bioactive compounds. Halymenia is a supply of natural pigments and also contains bioactive components which have been broadly reported, particularly within the last 5 years, specifically as antioxidants, antibacterial, antimalarial, and antiviral (Deepak et al., 2019; Malik et al., 2020; Vinosha et al., 2019; Vinosha et al., 2020). Halymenia sp. grows wild in Indonesian waters which so far have not been widely used. The results of the latest research presented by Sanger et al. (2019) and Khatulistiani et al. (2020) showed that Halymenia sp. from the waters of North Sulawesi and Banten have potential as antidiabetic and antioxidant agents.
Halymenia durvillei is found in abundance in the waters around Makassar city, especially on Kayangan island. So far, studies on its potential bioactivity are still limited. Therefore, this study needs to be carried out to determine the antibacterial activity and toxicity of H. durvillei found on Kayangan island, South Sulawesi.
Materials and Methods
The main ingredients used were H. durvillei seaweed, methanol and hexane PA grade (Merck, Darmstadt, Germany), and dimethyl sulfoxide (DMSO; Merck). The test bacteria Escherichia coli, Salmonella typhi, and Pseudomonas aeruginosa had been obtained from the Faculty of Medicine, Hasanuddin University, while Aeromonas hydrophila, and Vibrio harveyi had been received from the Center for Brackish Water Aquaculture Fisheries (BPBAP) Takalar. Artemia salina egg test animals had been acquired at the Marine Biology Laboratory, Hasanuddin University. The growth media for bacteria are nutrient broth (NB; Oxoid, Basingstoke, UK), nutrient agar (NA; Oxoid), and tryptone soya agar (TSA; Oxoid). 6 mm Diameter paper disk, Whatman No. 1 filter paper (Merck), and the commercial antibiotic ciprofloxacin. Seawater was taken from the waters around Kayangan island. The equipment used was a blender (Philips HR 2221, Philips, Amsterdam, Netherlands), autoclave, and oven.
H. durvillei red seaweed (Fig. 1) was collected from Kayangan island waters in June 2020. The sampling location was about 3 km west of Makassar city. Samples were taken at a depth of 1 to 1.5 meters using snorkel equipment and then washed with seawater to remove adhering dirt such as sand and shellfish. The samples had been placed into sample plastics and stored in a coolbox containing ice as a cooling medium to keep the freshness of the seaweed for the duration of transportation to the laboratory. Moreover, the samples were washed with running water to clean seawater and remaining dirt, sooner rinsed with distilled water, after which drained and weighed to determine the wet weight of the seaweed. The samples were dried for 10 days then mashed using a blender after which sieved to attain seaweed flour. Seaweed flour was weighed and packed in an airtight container and stored at room temperature before use. The yield was expressed in the weight percentage of seaweed flour produced to the weight of wet seaweed.
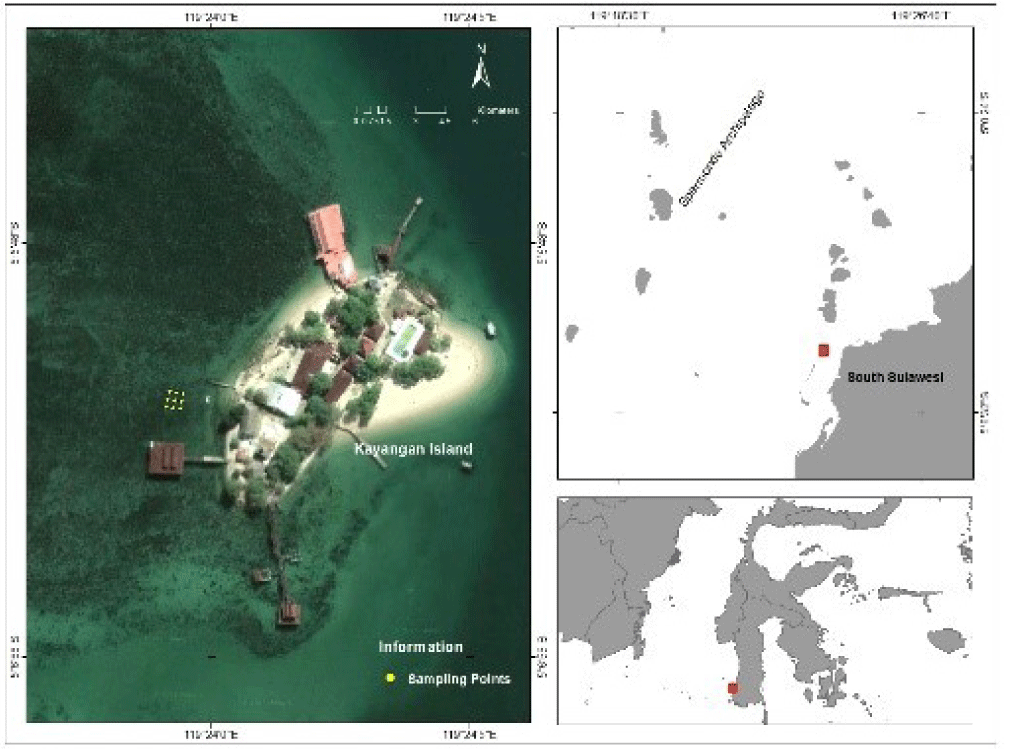
Extraction of samples by using maceration method the use of organic solvents methanol and hexane according to El Shafay et al. (2016) which had been modified. A total of 150 g samples had been immersed in 600 mL of every solvent (1:4 w/v) and left at room temperature for three days while stirring occasionally with the use of a magnetic stirrer. The walls and glass surface of the maceration container were protected with aluminum foil to keep away from touch with open-air and light exposure. The maceration results were vacuum filtered with Whatman paper No. 1 to obtain the filtrate which was then concentrated using a rotary vacuum evaporator at a temperature of 40°C–45°C. The resulting extract was weighed and then stored in the freezer before use. The yield of the extract was expressed as a percentage of the weight of the resulting extract to the weight of the seaweed flour used in extraction.
NB and NA media had been prepared one after the other dissolving 13 g NB and 23 g NA in 1 L of distilled water and after which heated till dissolved. Both media had been sterilized in an autoclave at 121°C for 15 minutes. Similarly, TSA media become prepared in an equal way as NA media. The agar medium was cooled to about 50°C before being poured into the Petri dish. The test bacteria had been rejuvenated on sterile sloping media and incubated at 37°C for 24 hours. A complete of 1 ose of bacteria on slanted agar turned into inoculated on NB media, homogenized the use of a vortex, after which incubated at 37°C for 24 hours to obtain viability of 108 colonies/mL.
The antibacterial activity test of crude extracts of methanol and hexane refers to Bauer et al. (1966) and Christobel et al. (2011) with the modified agar diffusion method. A complete of 1 mL of each bacterial culture turned into inoculated on NA media (besides V. harveyi on TSA media) in a Petri dish. Every extract of methanol and hexane turned into taken as plenty as 1,000 µg and dissolved in 50 µL of the solvent till dissolved. 10 µL turned into taken and carried out to a 6 mm diameter paper disk at a dose of 200 µg/disk. After the solvent evaporated, every disc changed into dripped with 5 µL of DMSO after which placed on agar media that already contained the test bacteria. As a positive control, the commercial antibiotic ciprofloxacin 5 µg was used (El Shafay et al., 2016) at the same time as the negative control used DMSO 5 µL. Petri dishes that already comprise the test sample are wrapped with plastic wrap and incubated at 37°C for 24 hours. The clear zone formed around the disc indicated the inhibition of bacterial activity by the extract which turned into expressed in mm as the average value of three replications.
An egg hatching container was prepared, namely a two insulated vessel with a 2 mm diameter hole as a connector, one room was left dark and the other room was lit with lamps. A total of 250 mL of seawater was filled into the dark and aerated. Inserted 2.5 mg of artemia eggs then covered with aluminum foil, the other side was left to stay bright. The eggs hatch after 24 hours into larvae and move to a bright room through a connecting hole. After 48 hours larvae or nauplii are ready to use for toxicity tests (Nurhayati et al., 2006; Puspitasari et al., 2018).
Stock solutions of each methanol and hexane extract were prepared by weighing 100 mg of extract and then dissolved in 10 mL of solvent. A series of dilutions were made by taking 1,000, 500, 250, 125, 62.5, and 31.25 µL of the stock solution, respectively, into the vial, and the solvent was evaporated. Each was added 100 µL of DMSO and 2 mL of seawater and stirred until the extract was completely dissolved. Furthermore, the extract solution was transferred to a 10 mL volumetric flask and then added with seawater to a volume of 10 mL, homogenized to obtain an extract solution with concentrations of 1,000, 500, 250, 125, 62.5, and 31.5 µg/mL. The solution was transferred into a glass vial and then 10 larvae of A. salina were added to each. Each dilution was made 3 times with a solution without the addition of extract as a negative control. Observation of larval mortality was carried out after 24 hours at room temperature. Data analysis to calculate half-maximal inhibitory concentration (IC50) µg/mL with sigmoid type inhibition curve (Kasmiati et al., 2018).
Results
The yield of H. durvillei flour is the percentage ratio of the weight of seaweed flour produced (337 g) to the weight of wet seaweed (6,600 g) which is 5.11%. Furthermore, the yield of methanol and hexane extracts were 5.32% and 4.11%, respectively, which was expressed in terms of the percentage by weight of the crude extract produced, namely 7.98 and 6.16 g to the weight of 150 g seaweed flour, respectively. This indicates that the biomass of H. durvillei contains more polar compounds.
In this study, we tested the antibacterial activity of methanol and hexane extracts of H. durvillei against gram-negative bacteria. Three of the five gram-negative bacteria used are bacteria that cause disease in humans, namely E. coli, S. typhi, and P. aeruginosa, while the other two bacteria A. hydropila and V. harveyi are bacteria that commonly attack well-cultivated organisms shrimp and fish. Methanol extract from H. durvillei confirmed antibacterial activity against all test bacteria besides E. coli.
An important finding resulting from this study is the methanol extract of H. durvillei showed the highest activity against S. typhi with an inhibition zone of 26.2 mm. The inhibitory ability of the H. durvillei methanol extract was two times higher than the commercial antibiotic activity of ciprofloxacin as a positive comparison (Table 1). This indicates that the methanol extract of H. durvillei has excellent potential as a supply of new antibiotics, especially against S. typhi. Another thing that was found in this study was that the methanolic extract of H. durvillei had moderate inhibitory activity against P. aeruginosa, A. hydrophila, and V. harveyi with inhibition zones of 9.6, 9.3, and 8.9 mm, respectively (Table 1). The zone of inhibition in question is the diameter of the clear zone formed including a 6 mm diameter paper disk (Fig. 2).
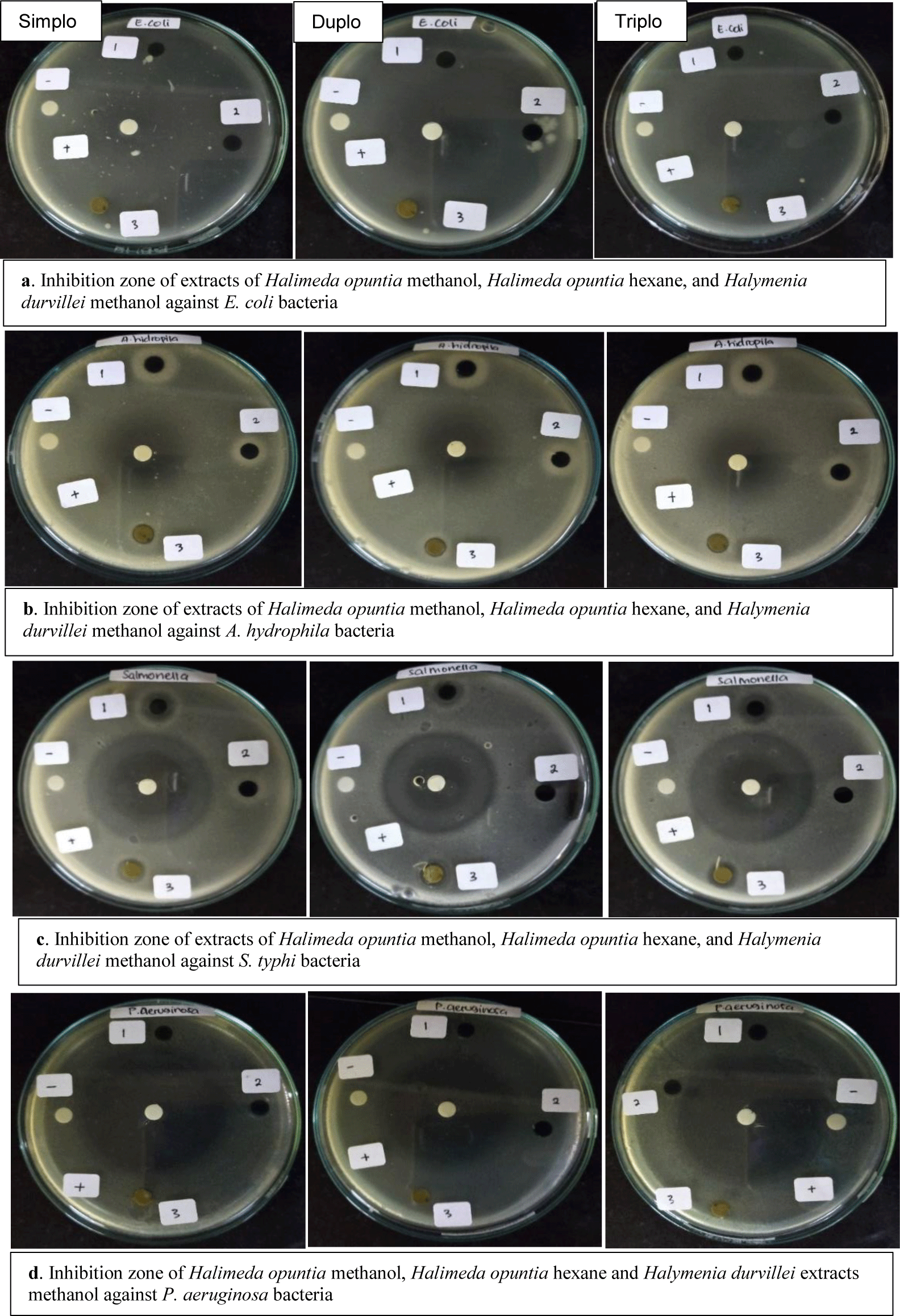
Hexane extract H. durvillei showed inhibitory activity against all test bacteria except against bacteria P. aeruginosa (Fig. 3). Extract activity against A. hydrophila showed the highest response with a zone of inhibition 21.0 mm higher than the positive control of ciprofloxacin antibiotics (Table 1). The potency of the inhibition of hexane extract against the other three bacteria was moderate with inhibition of 8.5, 11.3, and 8.0 mm for each of the tested bacteria E. coli, S. typhi, and V. harveyi.
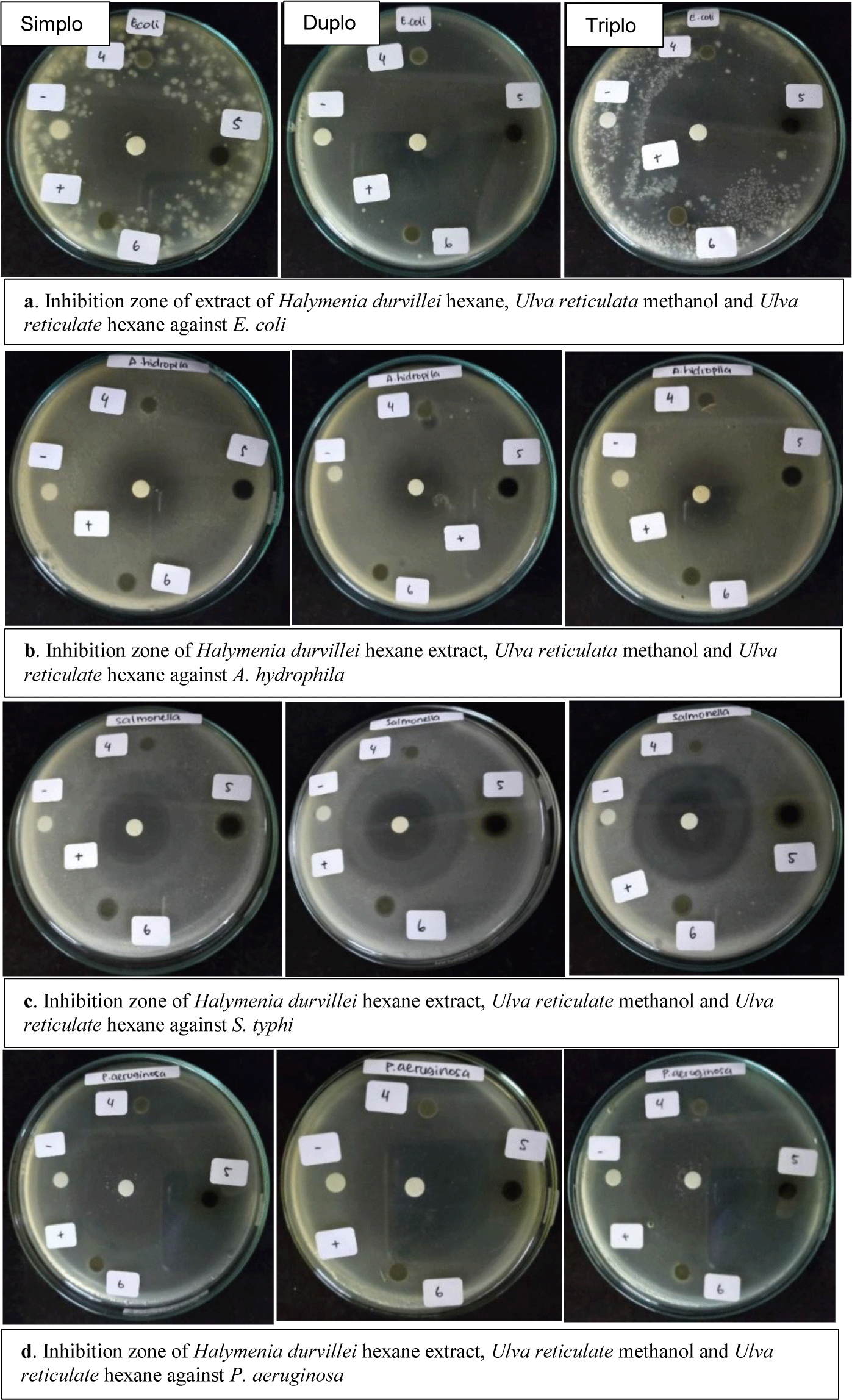
In this study, the potential toxicity of red seaweed extract H. durvillei was also tested against larvae A. salina as a preliminary study to determine the potential of methanol and hexane extracts as anticancer agents as listed in Table 2.
Toxicity testing was based on the ability of the extract to inhibit the growth of the larvae of the test animals, the lower the concentration of the extract to inhibit 50% of larval growth, the more potent the extract was. Toxicity of seaweed methanol and hexane extracts H. durvillei evaluated after exposure for 24 hours at room temperature. After 24 hours, the percentage of dead larvae was calculated for each concentration. Methanol extract causes mortality rate A. salina respectively 10%, 42%, 58%, 75%, 80%, and 100% at a concentration of 31.25; 62.5; 125; 250; 500; and 1,000 µg/mL. Meanwhile, the hexane extract at the same concentration caused average mortality of larvae of test animals of 30%, 36%, 41%, 48%, 61%, and 82% after exposure for 24 hours.
The results of this study indicate the potential toxicity of methanol extract H. durvillei higher than the hexane extract activity with IC50 of 96.24 and 184.21 µg/mL, respectively.
Discussion
Seaweed is a unique resource from the ocean because of its ability to thrive in the dynamic marine environment. A study showed that the antibacterial activity of seaweed is due to the symbiotic relationship between bacteria and seaweed which plays an important role in the development and growth of seaweed (Singh & Reddy, 2014). Seaweed contains epiphytic bacteria which are an excellent source of natural antimicrobial and antioxidant compounds (Horta et al., 2014). The potential broad-spectrum antibacterial effect of epiphytic bacteria (Phylum: Firmicutes; closely related to Bacillus pumilus) suggests that seaweed-associated bacteria may be producers of antibacterial compounds (Ismail et al., 2016). Another study by Sujuliyani et al. (2019) showed that C. racemosa had symbiotic bacteria belonging to the genus Neisseria that we’re able to synthesize potential antibacterial compounds against Staphylococcus aureus and S. typhi. Our results show that H. durvillei seaweed is a potential source of antibacterial compounds.
The results of the study showed that the methanol extract of H. durvillei showed antibacterial activity against all test bacteria except E. coli. The results obtained may be greater or much less similar to results reported through previous experiments. For instance, about the effect of different extraction solvents on the antibacterial activity of the different seaweeds, ethanol extract of Sargassum vulgare was reported to exhibit inhibitory activity against S. aureus and Klebsiella pneumoniae (Bolaños et al., 2017). Another previous study reported alkaloid extracts from brown (S. vulgare, Dictyopteris membranacea, and Cystoseira barbata), green (Ulva lactuca and Codium tomentosum), and red seaweeds (Gelidium latifolium), respectively, exhibited remarkable antibacterial activity against S. typhi, S. aureus, Staphylococcus epidermidis, Bacillus spp., Bacillus subtilis, E. coli, Klebsiella spp. and P. aeruginosa (Alghazeer et al., 2013). Our results might be different from the previous findings which demonstrated greater activity, but our results showed antibacterial activity against all test bacteria except E. coli. According to Nazarudin et al. (2020), the absence of the inhibition zone is probably due to the low concentration of antibacterial active metabolites, which causes resistance of microorganisms to seaweed extracts. The research found by Yap et al. (2019) stated that the methanol extract of C. racemosa had moderate antibacterial activity against E. coli K1 (gram-negative bacteria). Another research also reported by Al-Saif et al. (2014) using the disc diffusion method showed that the chloroform extract from Caulerpa occidentalis was very effective against E. coli but has moderate antibacterial activity against S. aureus and Enterococcus faecalis. The difference in results may be due to the effect of seaweed’s antibacterial activity, such as the different species of seaweed tested, the solvent used for extraction, the geographical location of the seaweed, and so on (Anjali et al., 2019; Nazarudin et al., 2020).
As mentioned before methanol extract exhibit a higher antibacterial activity against S. typhi. This indicates that the methanol extract of H. durvillei has great potential as a source of new antibiotics, especially against S. typhi. S. typhi is the causative agent of systemic infection and typhoid fever in humans. Salmonellosis is common in people in developing countries and is a high-risk group (Hussain et al., 2019; Sah et al., 2020). To prevent complications of death and disease, antibiotics are the first treatment modality in an enteric fever until the prevalence of S. typhi (Wong et al., 2019). Salmonella is known to be resistant to various types of antibiotics such as ampicillin, chloramphenicol, and trimethoprim-sulfamethoxazole (Akram et al., 2020). Thus, the results of this study are important findings as an initial step in efforts to discover natural compounds from seaweed as an alternative solution to overcome resistance Salmonella to commercial antibiotics. Substitution of antibiotics with natural functional molecules will help overcome the currently developing resistance to antibiotics by pathogenic microorganisms (Fraga-Corral et al., 2020; Kini et al., 2020). In addition to having activity against the test bacteria in this study, the methanol extract H. durvillei also shows activity against K. pneumoniae (Hamza et al., 2014). In a study of the antibacterial activity of seaweed, S. typhi is a species of Salmonella the most widely used as test bacteria. This is because testing with S. typhi allows the classification of moderate or moderate to susceptible antibacterial activity (inhibition zone diameter more than 15 mm) (Akremi et al., 2017; Silva et al., 2020). Thus the antibacterial activity of red seaweed methanol extract H. durvillei found in this study is categorized as high in other words S. typhi is susceptible to methanol extract H. durvillei. Therefore, the abundance of seaweed as a source of active compounds can be used to obtain extracts rich in antimicrobial components to be applied in the food, cosmetic, and pharmaceutical fields (Silva et al., 2020).
The results of the study showed that the hexane H. durvillei extract showed inhibitory activity against all the tested bacteria except for P. aeruginosa. The activity of the extract against A. hydrophila showed the highest response. This indicates that the red seaweed hexane extract H. durvillei has promising potential as an antibacterial agent, especially against pathogenic bacteria A. hydrophila causes disease and crop failure in cultured fish (Rozi et al., 2018). The results of this study are supported by the report of Vinosha et al. (2020) that the sulfate galactan component Halymenia sp., besides having antioxidant activity, is also able to increase the resistance of fish Oreochromis against bacteria Aeromonas hydrophilic. Thus the antibacterial activity of red seaweed hexane extract H. durvillei was found in research. This could be a promising finding to reduce the use of antibiotics in the aquaculture sector where antibiotic-resistant bacteria commonly thrive.
To the best of our knowledge, the difference in results on antimicrobial activity may be due to differences in bacterial cell wall configuration and permeability, bacterial composition, and resistance charge between the cell wall of pathogenic and secondary bacteria metabolism (Amorim et al., 2012; Lin et al., 2014; Peng et al., 2017). Regarding secondary metabolites, phenolic compounds are a group of secondary metabolites which are components of seaweed cell walls or as a defense mechanism against abiotic and biotic stressors (Generalić Mekinić et al., 2019). Several mechanisms of antimicrobial activity of phenolic compounds are inhibition of enzymes and metabolic pathways, changing membrane permeability that causes cell lysis, and binding to surface molecules (Silva et al., 2020). The mechanism of action of antibacterial activity disrupts membrane stability and cellular function in microorganisms by interacting with bacterial cell wall glycoreceptors, membrane compounds, and nucleic acids (Silva et al., 2020). Another report states that the mechanism of antimicrobial activity is the accumulation of lysozyme (immune enzyme) which digests bacterial cell walls (Perez-Santaescolástica et al., 2022). In addition to the phenolic components, previous reports stated flavonoids (Górniak et al., 2018) and alkaloids (Casciaro et al., 2020) are also important antimicrobial agents against a variety of microorganisms.
Method Brine Shrimp Lethality Test (BSLT) uses shrimp larvae A. salina as test animals to find new anticancer compounds derived from plants (Kim & Choi, 2017). This was also stated by Zamani et al. (2017) that the determination of toxicity as an initial test is a very important part of research in the search for drug substances or drug discovery. Several methods have been developed to study the viability and proliferation of cultured cells. The most widely applied method is to use a 96-well-format which is based on the principle of colorimetry. Through this method, a large number of samples can be tested quickly and simultaneously. The cytotoxic assay with this platform can directly measure the enzymatic activity in the microplate using a microplate reader (Weyermann et al., 2005).
The results of this study showed that the potential toxicity of the methanol extract of H. durvillei was higher than that of the hexane extract. The toxicity of the methanol extract is slightly lower than the toxicity of the red algae ethanol extract Eucheuma cottonii namely 58.01 µg/mL (Sharo et al., 2013). On the other hand, the extract potency of H. durvillei in this study was higher than the toxicity Sargassum sp. proposed by Riyanto et al. (2014) and Jannah et al. (2014) which are 170 and 139 µg/mL, respectively. The potential toxicity of the methanol extract found in this study is similar to the toxicity of the red seaweed ethanol extract Eucheuma spinosum to A. salina with an IC50 of less than 100 µg/mL (Hamrun et al., 2020). Red seaweed toxicity was also reported by De Alencar et al. (2014) that the ethanol extract Amansia multifida and Meristiella echinocarpa besides having antioxidant activity, the total phenolic content of 45.40 and 28.46 mg gallic acid equivalent g-1 also showed toxicity to A. salina.
Thus, red seaweed H. durvillei which is found abundantly in Kayangan island, South Sulawesi waters, has the opportunity to be developed as a sourcing agent for new compounds to overcome the development of abnormal cells in human tissues.
Conclusion
Crude extract of methanol and red seaweed hexane H. durvillei from Kayangan island, South Sulawesi, each has high antibacterial activity, especially against gram-negative bacteria S. typhi and A. hydrophila. Methanol extract has promising toxicity with IC50 value of less than 100 µg/mL which indicates that the extract has potential as a source of new anticancer compounds.