Introduction
Cardiovascular diseases (CVDs) have gained more attention due to their high morbidity and mortality worldwide (Kaptoge et al., 2019). CVD is a multifactorial disease, which could be caused by inflammation, hypertension, atherosclerosis, dyslipidemia, insulin resistance, elevated heart rate and enteric dysbacteriosis (Ebbesson et al., 2015; Zhou et al., 2021). Endothelial cells play an essential role in cardiovascular homeostasis which are important constituents of blood vessels. Normal vascular endothelium is considered as a gatekeeper of cardiovascular health, while abnormal vascular endothelium is the main cause of CVDs (Sun et al., 2020). Extensive evidence supports that inflammation plays a key role in the pathogenesis of CVDs by promoting endothelial cell activation or dysfunction (Carter, 2012). Therefore, the prevention, systematic management and effective intervention of vascular disease are particularly important and necessary (Liu and Wang, 2016).
Saturated fatty acids (FAs) such as palmitate (PA) have been associated with cardiovascular mortality, which was thought to induce chronic low-grade inflammation, activate the innate immune system, and bring harmful effects on vascular cells and other tissues and organs through the inflammatory processes (Ebbesson et al., 2015; Volpe et al., 2014). Vascular dysfunction is tumor necrosis factor- (TNF-) α dependent and is related to the increase of inducible nitric oxide synthase (iNOS) levels in aortic endothelial cells and excessive generation of nitric oxide (NO), which is the reason for the reduced reactivity of aortas to vasoconstrictors. Therefore, TNF-α blockers might be useful in the prevention of CVDs (Aires et al., 2013) . In addition, LIM domain only 2 (Lmo2) is an essential gene for vertebrate primitive hematopoiesis and normal endothelial development (Zhu et al., 2005); Friend leukemia integration 1 transcription factor (Fli1) plays an important physiological role in vascular development at the top of the transcriptional network that regulates blood and endothelial development (Li et al., 2015). Thus, maintaining the balanced expression of vascular-related genes also plays a positive role in preventing the occurrence of CVDs (Seo et al., 2006).
At present, several synthetic drugs have been applied for treatment of CVDs, whereas some adverse effects such as arrhythmias, hyperkalemia and gastrointestinal reaction are giving cause for concern (Giudicessi et al., 2018; Ziff and Kotecha, 2016). On the contrary, mounting evidence suggests that natural products could be a safe and effective alternative for the prevention and treatment of CVDs (Miller et al., 2017; Tang et al., 2017; Tufail et al., 2018). Sea cucumber, belongs to the class of Holothuroidea and the phylum of Echinodermata, has attracted much attention as a marine functional food due to its biological activity and medicinal properties (Hossain et al., 2020). Several studies have confirmed that sea cucumbers and their extracts have various potential containing anti-inflammatory (Kareh et al., 2018), antithrombotic (Chen et al., 2012), anticoagulant (Mansour et al., 2019), hypoglycemic activities (Hu et al., 2013) and hypolipidemic (Liu et al., 2002), all of which have significant impact on the prevention and treatment of CVDs.
Apostichopus japonicus (A. japonicus) is a species of sea cucumber in the family Stichopodidae. Previous studies have shown that A. japonicus polysaccharides have antioxidant and antihyperlipidemic activities (Liu et al., 2012). Moreover, the nortriterpene and triterpene glycosides from A. japonicus displayed antifungal activities (Wang et al., 2012). And the depolymerized glycosaminoglycan from A. japonicus showed anticoagulant activities (Yang et al., 2015a; Yang et al., 2015b). Furthermore, A. japonicus extract (AJE) has been shown to have anti-skin cancer activities (Kim et al., 2017). However, the research evidence about the function of A. japonicus in vascular dysfunction has not been revealed. Zebrafish is recognized as a link between invertebrate and mammalian models due to its vascular development of the circulatory system and molecular pathways are highly similar with human and other higher vertebrates (Li et al., 2014). In recent years, the vascular-specific Tg (fli1a:eGFP) transgenic zebrafish has been exceptionally useful for examining vascular development in zebrafish (Lawson and Weinstein, 2002). Therefore, the present study applied PA to stimulate human umbilical vein endothelial cells (HUVECs) and zebrafish embryos to establish in vivo and in vitro models to evaluate whether the extracts from A. japonicu have protective effect against PA-induced vascular damage.
Materials and Methods
A 80% of ethanol extract of A. japonicus (AJE) was donated from Marine Bio Resource Information System (MBRIS), https://www.mbris.kr/.
The embryos of wild type zebrafish were derived from the zebrafish system of our lab. The management of zebrafish was in accordance with established procedures, http://zfin.org/zf_info/zfbook/zfbk.html. Transgenic zebrafish line Tg (fli1a:eGFP) was acquired from Zebrafish Center for Disease Modeling (ZCDM). Zebrafish embryos used in the experiment were processed according to the guidelines of Hanseo University.
Palmitic acid (PA; Sigma-Aldrich, St. Louis, MO, USA) was dissolved in pre-heated 0.1 N NaOH (Daejung, Korea) at 70°C and conjugating with FA-free bovine serum albumin (BSA; Sigma-Aldrich) to obtain a 10 mM stock solution, which was improved based on the previous report (Sinha et al., 2004). We prepared two stock solutions: one for embryos, which mixing 20 mM PA with 12% BSA (w/v) containing DPBS (Dulbecco’s Phosphate Buffered Saline, Welgene) at a ratio of 1:1; another one for cell culture that mixing 20 mM PA with 12% BSA (w/v) containing DMEM (Dulbecco’s Modified Eagle’s Medium, Welgene, Gyeongsan, Korea) at a ratio of 1:1. Control solution contained NaOH and BSA without lipids.
Tg (fli1a:eGFP) transgenic embryos were applied to evaluate the effect of AJE on vascular formation under PA treatment. Embryos at 24 hours post-fertilization (hpf) were distributed to 12-well plate (20 embryos/well) and incubated in the AJE (25 μg/mL) for 1 h prior to the additional PA (2 mM) in 2 mL embryo media (0.003% sea salt, 0.0075% calcium sulfate) including 75 μM phenylthiourea (PTU, Sigma-Aldrich) for 96 hpf at 28.5°C. The phenotype of embryos was checked daily, the malformation and mortality were calculated, and the dead embryos were removed from the well in time. The phenotype morphology, yolk sac area and body length of embryos were observed by a stereomicroscope (SZ61, Olympus, Tokyo, Japan) and measured using cellSens software (Olympus). Vascular morphology, axial vascular segment (AVS) and intersegmental vessel (ISV) were observed and measured by a fluorescent microscope (SZX16, Olympus).
Heart rate is an important indicator reflecting the toxicity of cardiac development (Sarmah and Marrs, 2016). After embryos were exposed to PA or AJE/PA from 24 to 75 hpf, 12 embryos were randomly selected from each group and the heart rate was recorded by counting the beats per minute under stereomicroscope (SZ61). The results were presented in average heart rate per minute.
NO production was measured in living zebrafish embryos by a fluorescent probe dye of diaminofluorophore 4-amino-5-methylamino-2’,7’-difluorofluorescein diacetate (DAF-FM DA, Invitrogen, Carlsbad, CA, USA). In the presence of dioxygen, NO transforms DAF-FM DA to produce highly fluorescent triazole derivatives. Cell death was determined in living zebrafish embryos by staining with acridine orange (AO, Sigma-Aldrich). AO is a kind of cell-permeable nucleic acid dye that can be released into the nucleus when a cell begins to die, and interacts with partially uncoiled DNA to produce high fluorescence (Thomé et al., 2016). The embryos developed to 86 hpf were rinsed and stained with 5 μM DAF-FM DA or 5 μg/mL AO for 30 min at 28.5°C in the dark. After fully rinsed and anesthetized by 0.003% methanesulfonate (MS-222), the embryos were observed and photographed under a fluorescent microscope (SZX16, Olympus). The fluorescence intensity of images was quantified by ImageJ software (http://rsb.info.nih.gov/ij) and the triplicate mean value were represented by a bar graph.
Total RNA was isolated from zebrafish embryos using TRIzol Reagent (Invitrogen), and RNA was reverse-transcribed to cDNA by cDNA synthesis PrimeScriptTM 1st strand cDNA Synthesis kit (Takara) following the manufacturer’s instructions. qPCR was performed using TOPrealTM qPCR 2X PreMIX (SYBR Green with low ROX, Enzynomics) on Qiagen Rotor-Gene Q instrument (Hilden Düsseldorf, Germany). Gene expression levels were acquired based on the normalization of the endogenous β-actin gene, which was not affected by PA. Relative expression was calculated with ΔΔCT method. The sequences of q-PCR primer pairs were presented in Table 1.
All graphs were generated by GraphPad prim version 9.0 (GraphPad software, San Diego, CA, USA) and one-way analysis of variance (ANOVA) with subsequent multiple comparison test (Tukey) were applied for significance analysis. Data are presented as mean ± SD. p < 0.05 was considered statistically significant.
Results
To evaluate the toxicity of PA on embryonic development in zebrafish, we investigated the survival rate of embryos treated with PA (1.5–3 mM) at the 24 hpf stage during 62 h and screened the concentration of PA. As shown in Table 2, 20 % and 100% lethality occurred by 50 hpf at 2.5 mM and 3 mM of PA, respectively. No mortality occurred in embryos at 1.5 and 2 mM by 86 hpf and we selected a concentration of 2 mM PA for subsequent experiments. Furthermore, we confirmed that AJE alone had no toxicity to embryos in the concentration range of 5–50 µg/mL since no significant change in heart rate (Table 3).
Treatment at 7 hpf | |||||
---|---|---|---|---|---|
AJE (μg/mL) in egg water | |||||
0 | 5 | 10 | 50 | 100 | |
Mortality (50 hpf) | 0/20 | 0/20 | 0/20 | 0/20 | 0/20 |
Heartbeat (3 dpf) | 180 ± 15 | 171 ± 14 | 186 ± 19 | 186 ± 19 | 129 ± 9* |
Treatment at 24 hpf | |||||
---|---|---|---|---|---|
PA (mM) in egg water with PTU | |||||
0 | 1.5 | 2 | 2.5 | 3 | |
Mortality (50 hpf) | 0/20 | 0/20 | 0/20 | 4/20 | 20/20 |
Heartbeat (2 dpf) | 145 ± 5 | 150 ± 5 | 159 ± 14** | – | – |
To determine whether AJE has a protective effect on PA-induced embryotoxicity, we analyzed the developmental phenotypes containing heartbeat, survival, malformation, yolk sac area and body length, to identify morphological differences of 24 hpf embryos after exposed to PA (2 mM PA) alone or 1 h pretreatment with AJE (25 μg/mL) prior to PA. The results showed pretreatment with AJE could reduce the PA-induced acceleration of heartbeat at 75 hpf and increase embryos survival rate at 55 and 75 hpf relative to the control group (Fig. 1a, b). Meanwhile, PA treated embryos revealed morphological abnormalities, including spinal curvature, edema, and delayed absorption of yolk sac, which were alleviated by pretreatment with AJE (Fig. 1c, d). In addition, the yolk sac area and body length were larger and shorter than that of the control group, respectively, which were also improved by AJE treatment (Fig. 1e, f).
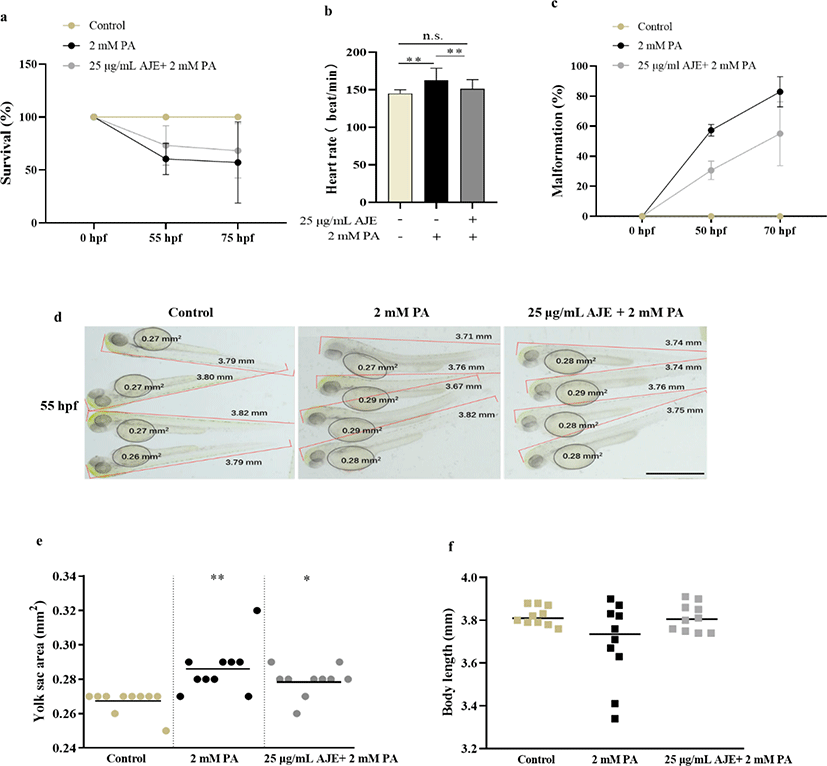
The overproduction of NO in blood vessels is related to circulatory failure, which occurs in systemic inflammatory response and may have cytotoxicity to surrounding tissues (Stoclet et al., 1998). Therefore, we examined whether excessive NO induced by PA (2 mM) could be reduced by AJE in zebrafish. After pretreatment with AJE (25 μg/mL), the excessive NO production induced by PA was significantly reduced compare with control (Fig. 2a). Moreover, mRNA levels of inflammatory cytokines such as iNOS and TNF-α were enhanced by PA, while significantly decreased by AJE (Fig. 2b, c). TNF-α has been shown to induce NF-κB activation which result in cell death (Zhi et al., 2015). Our results showed that PA-induced NF-κB activation which was attenuated by AJE (Fig. 2d). Meanwhile, PA-induced cell death was significantly higher than in the control group, however, pretreatment AJE significantly reduced the level (Fig. 2e). These results suggest that AJE can protect zebrafish embryos against PA-induced inflammation and cell death.
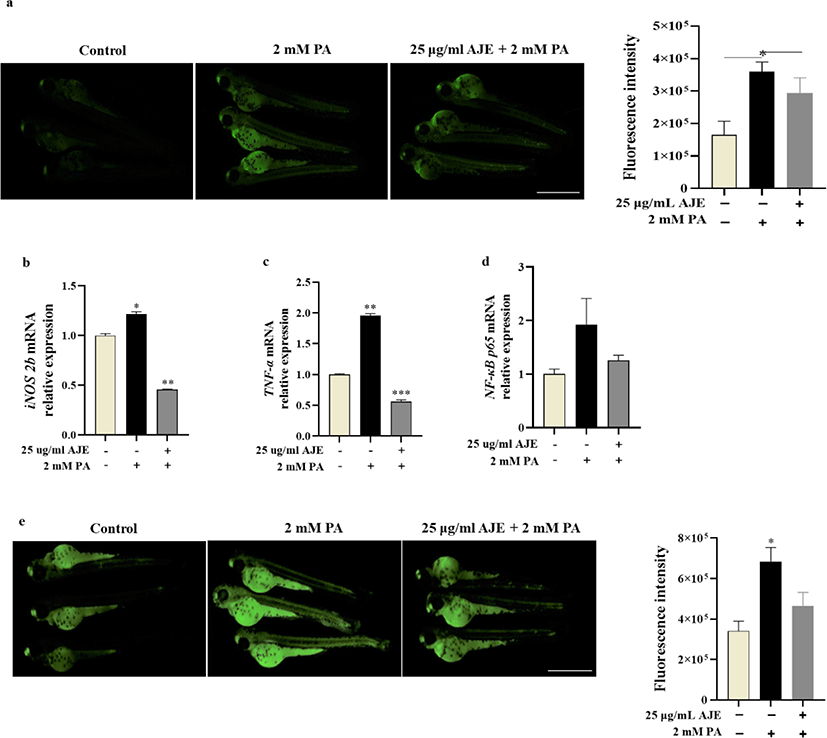
Vascular morphogenesis includes three major processes that vasculogenesis, angiogenesis and vascular remodeling (Patan, 2000), among which angiogenesis plays a key role in the pathological process of CVDs (Zhang et al., 2018). To evaluate the phenotypic effects of PA or AJE treatment on vascular morphogenesis, we measured the length of AVSs and ISVs in Tg (fli1a:eGFP) transgenic zebrafish embryos treated with 2 mM PA, or co-treated with AJE at 24 hpf. The results showed the length of AVSs and ISVs are both significantly decreased after PA treatment compare with control at 60 hpf. However, it has been improved by pretreatment of AJE (Fig. 3a, b). In addition, we determined the mRNA levels of Fli1 gene, which regulates endothelial development, as well as Lmo2, a key gene necessary for angiogenesis and related to ISV formation (Ganta and Annex, 2017; Li et al., 2015; Meng et al., 2016). The results showed that the mRNA expression of both Lmo2 and Fli1 in PA-treated groups was significantly higher than those in control group, while the expression level of the AJE pretreatment group was reduced to a level comparable to that of the control group (Fig. 3c, d).
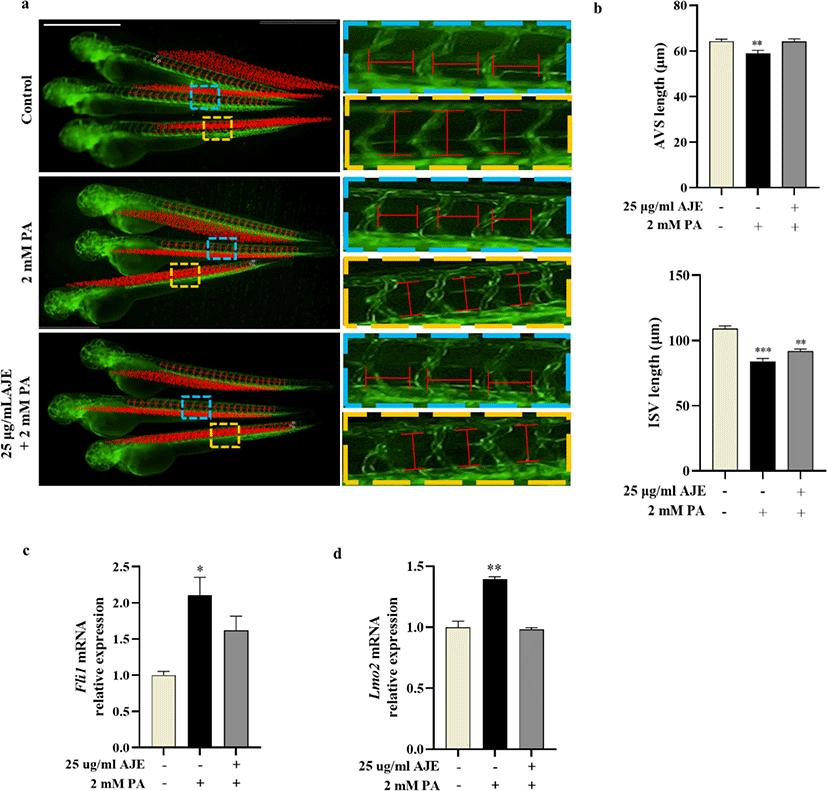
Discussion
The etiology of CVDs is multifactorial, lifestyle changes and drug treatment can reduce the incidence of CVDs, such as heart attacks and strokes, thereby reducing premature morbidity, mortality and disability (WHO, 2007). Evidence confirming that A. japonicus has antioxidant, hypolipidemic, antifungal, anticoagulant and anti-skin cancer activities (Kim et al., 2017; Liu et al., 2012; Wang et al.; 2012, Yang et al., 2015a; Yang et al., 2015b). To our knowledge, its protective effect on blood vessels is not reported so far. PA is well known to damage the proliferation and invasion of HUVECs in vitro, and increase cell apoptosis (WHO, 2007). Therefore, this study firstly established the PA-induced apoptosis model of HUVECs, and confirmed that AJE has protective effect on PA-induced damage in HUVECs (Data was not shown). Furthermore, we established an in vivo zebrafish model induced by PA and evaluated the efficacy of AJE-pretreatment. In this study, AJE-pretreatment reduced the physiological indicators similar to the symptoms of CVDs, such as mortality, accelerated heartbeats and malformations induced by PA in zebrafish embryos (Fig. 1). These results preliminarily suggested that AJE may have the potential to prevent CVDs due to its inhibitory effect on PA-induced toxicity in both in vitro and in vivo.
Previous studies demonstrated that abnormal production or activity of NO is considered to be a major mechanism of CHD as a CVD (Besedina, 2016). As well as iNOS-derived NO is also proposed as adverse effects on myocardium (D’Oria et al., 2020). Evidence confirmed that increased expression of iNOS was detected in the endothelium of coronary arteries in aged rats (Csiszar et al., 2002; Santhanam et al., 2007). Additionally, the upregulation and overexpression of iNOS can induce cardiac apoptosis, hypertrophy, dilatation and fibrosis in mice (Umar & van der Laarse, 2010). In the present study, PA-induced excessive NO production and iNOS mRNA expression in zebrafish which was downregulated by AJE-pretreatment compared with the control (Fig. 1a, b). This result could explain the phenomenon of accelerated heartbeat may cause by the detrimental effects of iNOS-derived NO on zebrafish myocardium. Furthermore, evidence shows that diverse processes induced CVDs are converging on regulation of TNF-α signaling and result in endothelial dysfunction and vascular disease. TNF-α can independently or depend on the activation of transcription factors, such as NF-κB, to induce gene expressions of various inflammatory cytokines and chemokines. This eventually leads to vascular remodeling, inflammation and oxidative stress, as well as atherosclerosis, thrombosis, endothelial cell apoptosis and impaired NO bioavailability (Zhang et al., 2009). In this study, mRNA levels of TNF-α and NF-κB were elevated by PA treatment while largely reduced after pretreatment of AJE (Fig. 1c, d). In addition, PA-induced embryonic cell death was also ameliorated by AJE-pretreatment (Fig. 1e). These results suggest that AJE probably block the NF-kB signaling pathway by inhibiting the overexpression of TNF-α, and ultimately prevent cell death induced by PA in zebrafish embryos.
Fli1 gene participates in the regulatory process of vasculogenesis and angiogenesis. Fli1 deficient xenopus and zebrafish embryos display a block in the development of hemangioblast, whereas Fli1 overexpression was observed in a variety of cancers and diseases (Li et al., 2015). In addition, Fli1 induces the expression of key hemangioblast genes such as Lmo2 (Liu et al., 2008). Lmo2 is a crucial determinant of tissue healing and angiogenesis in vivo zebrafish. Meng et al. identified that gene expression of Lmo2 elevated during caudal fin resection and regeneration (Meng et al., 2016). Further studies demonstrated that Lmo2 knockdown decreased the length and number of ISVs, impaired axial vessel formation and migration of endothelial cells (Matrone et al., 2017; Patterson et al., 2005). On the other hand, overexpression of Lmo2 caused T-cell lymphoblastic leukemia in mouse thymus (McCormack et al., 2010). In the present study, the length of AVS and IVS in Tg (fli1a:eGFP)zebrafish embryos was reduced under PA stimulation, while AJE-pretreatment ameliorated it close to the control (Fig. 2a, b). Furthermore, AJE-pretreatment protected against PA-induced overexpression of Fli1 and Lmo2 and maintained it at the control level (Fig. 2c, d). As we know, PA could inhibit angiogenesis by interfering with endothelial cell function (Zhang et al., 2017). In this study, we observed that PA-induced a mild or severe antiangiogenic phenotype on Tg (fli1a:eGFP) lines (data not shown). Therefore, our study suggests that PA probably induce varying degrees of embryonic anti-angiogenesis and trigger the up-regulation of Fli1 and Lmo2 expression levels to compensate for the dysfunction or damage of endothelial cells caused by PA, while AJE-pretreatment can block the negative effects of PA on zebrafish embryos.
Anti-TNF therapy is widely used in the treatment of many diseases including CVDs (Monaco et al., 2015). However, recent study pointed out that TNF inhibitors could decrease the CVDs events via controlling systemic inflammation, on the contrary can increase the risk of overall and certain cancers (Lee et al., 2018; Yuan et al., 2020). Our results suggest AJE, on the one hand, could be used as a substitute for TNF inhibitor to prevent CVDs by decreasing PA-induced over expression of TNF-α; on the other hand, may have the potential to reduce cancer risk by protecting zebrafish embryos against PA-induced overexpression of Fli1 and Lmo2. However, further study is necessary to elucidate.
In conclusion, AJE exhibited vascular protective effect in vivo zebrafish embryo models, AJE protected against PA-induced embryotoxicity by maintaining normal physiological indicators, regulating inflammation and genes expression related to vascular development. These findings might provide a potential mechanism basis for AJE in the prevention of CVDs.