Introduction
The genus Symphyocladia belongs to the family Rhodomelaceae and comprises several species of red algae. Seven Symphyocladia species (Symphyocladia linearis, Symphyocladia marchantioides, Symphyocladia glabra, Symphyocladia pumila, Symphyocladia lithophila, Symphyocladia jejuinsula, and Symphyocladia latiuscula) are known to inhabit Korean coasts (Kang & Kim, 2013). Among the seven species, S. latiuscula has been extensively studied for various biological activities, including anti-tyrosinase (Paudel et al., 2019c), anti-Alzheimer’s (Paudel et al., 2019b), anti-diabetic (Paudel et al., 2019a), antifungal (Xu et al., 2014), antiviral (Park et al., 2005), antioxidative (Zhang et al., 2007), and anti-aldose reductase activity (Wang et al., 2005). In addition, S. latiuscula has been registered as a food ingredient in Korea (Ministry of Food and Drug Safety) and is widely used industrially.
When subjected to environment stress (e.g., UV light, air pollution, ionizing radiation, smoke), reactive oxygen species (ROS) are generated, such as hydroxyl, superoxide, and lipid radicals. ROS induce lipid peroxidation and can damage DNA and proteins. Antioxidant enzymes, such as superoxide dismutase and peroxidase, help protect cells from oxidative stress, but if this system does not work properly and ROS cannot be removed, various conditions may develop, including high blood pressure, cancer, and diabetes (Lee, 2021). Several methods, such as oxygen radical absorbance capacity, hydroxyl radical antioxidant capacity, total peroxyl radical trapping antioxidant parameter, cupric ion reducing antioxidant capacity, ferric reducing antioxidant power, 2,2-diphenyl-1-picryl-hydrazyl-hydrate (DPPH), and 2,2’-azino-bis-3-ethylbenzothiazoline-6-sulfonic acid (ABTS), are used to measure antioxidant activity as determined by their ability to scavenge ROS (Munteanu & Apetrei, 2021).
The inflammatory response is an immune response that restores damaged tissues from external stimuli such as stress and injury. Inflammation is regulated by inflammatory mediators and cytokines secreted from macrophages, and synthesis of inflammatory factors such as nitric oxide (NO) and prostaglandin E2 (PGE2) may be induced by lipopolysaccharide (LPS) and reactive nitrogen species (Cho et al., 2009). When the production of inflammatory mediators increases, diseases such as diabetes, cancer, and atherosclerosis can develop; thus, it is important to suppress the generation of these inflammatory mediators (Liu et al., 2016; Yu et al., 2016).
Bacterial infection and the development of resistant bacteria continue to be serious concerns worldwide, necessitating the development of new antibacterial agents (Moghadamtousi et al., 2014). Staphylococcus aureus, Escherichia coli, Bacillus cereus, and Salmonella typhimurium are well known food-borne pathogens (Al-Zoreky, 2009; Kanatt et al., 2010). Research to identify marine algal extracts that are active against these bacteria is being actively conducted (Shannon & Abu-Ghannam, 2016). To date, research on Symphyocladia has been limited to S. latiuscula. Therefore, we examined another species, S. linearis, to determine its biological activity and potential use as an antioxidative, anti-inflammatory, or antibacterial agent.
Materials and Methods
S. linearis specimens were collected in April 2018 from Gangneung-si city, Gangwon-do province, Korea, and deposited at the National Marine Biodiversity Institute of Korea (MABIK).
Freeze-dried whole seaweed of S. linearis (30 g) was extracted by sonication using 70% ethanol (EtOH; 300 mL × 3) for 1 h, and evaporated to dryness by heating under reflux in vacuo. The dried 70% EtOH S. linearis extract (3.16 g dry weight) was stored at –80°C until biological activity assessment.
RAW 264.7 macrophages (ATCC, USA) were cultured in Dulbecco’s modified Eagle medium (DMEM) supplemented with 10% fetal bovine serum, penicillin (100 U/mL), and streptomycin (100 μg/mL) in a humidified incubator with 5% CO2 at 37°C.
To assess cell viability, 100 μL of RAW 264.7 macrophages was seeded in triplicate at a density of 3 × 105 cells/mL in wells of a 96-well plate and cultured for 20–24 h. After removing the DMEM, 10 μL of S. linearis extract in 80 μL of fresh medium was added to each well and the samples were incubated for 30 min. Subsequently, 10 μL of LPS was added and the samples were incubated for 20–24 h. Finally, 10 μL of Cell Counting Kit-8 reagent (Dojindo Laboratories, Japan) was added and the samples were allowed to react for 2 h. Thereafter, the sample absorbance in each well was measured at 450 nm using a microplate reader.
A slightly modified version of a method described by Blois (1958) was used to measure the DPPH radical scavenging activity of the S. linearis extract. Specifically, 100 µL of various concentrations (10–100 μg dry weight extract/mL) of the S. linearis extract was added to 100 µL of a 150 μM DPPH solution in a 96-well plate. The plate was shaken vigorously and placed in the dark at room temperature for 30 min, after which the absorbance of each sample was measured at 516 nm. The DPPH radical scavenging activity of the S. linearis extract was calculated and expressed as the half maximal inhibitory concentration (IC50) value.
The ABTS radical scavenging activity of the S. linearis extract was measured using a slightly modified version of a method described by Re et al. (1999). ABTS•+ was produced by mixing a 7 mM aqueous stock solution of ABTS•+ with 2.45 mM potassium peroxodisulfate in the dark at 4°C for 16 h. The stock solution was diluted with EtOH to an absorbance of 0.700 ± 0.100 at a wavelength of 734 nm. Various concentrations (10–100 μg dry weight extract/mL) of the S. linearis extract (100 µL) were added to wells containing 100 μg/mL of ABTS•+ solution in a 96-well plate. The plate was shaken vigorously and placed in the dark at room temperature for 15 min. The absorbance was then measured at 734 nm against a blank test.
The inhibition of ROS generation by the S. linearis extract was measured using a method described by Lee et al. (2021). RAW 264.7 cells were cultured in 96-well plates (3 × 105 cells/mL) and incubated at 37°C for 24 h. The cells were then incubated with 5 μM 2',7'-dichlorofluorescin diacetate (DCFH-DA) for 30 min in the dark, treated with various concentrations (10–100 μg dry weight extract/mL) of the S. linearis extract, and incubated again for 30 min. The cells were then exposed to 10 μg/mL LPS for 24 h. ROS production was measured by recording the fluorescence at an excitation/emission wavelength of 492/525 nm using a fluorescence microplate reader.
Inhibition of NO production by the S. linearis extract was measured using a method described by Lee et al. (2021). RAW 264.7 cells were plated in 12-well plates (3 × 105 cells/mL) and upon confluency they were treated with various concentrations (10–100 μg/mL) of the S. linearis extract and 100 μg/mL LPS for 24 h. Thereafter, the NO produced was measured using the Griess reagent kit (Invitrogen, USA). The absorbance of each sample well was measured at 548 nm using a microplate reader and inhibition of NO production by the S. linearis extract was expressed as a percentage (%) of the LPS-stimulated group value.
Inhibition of PGE2 production by the S. linearis extract was measured using a method described by Lee et al. (2021). RAW 264.7 cells were plated in 12-well plates (3 × 105 cells/mL) and upon confluency they were treated with various concentrations of the S. linearis extract (10–100 μg/mL) and 100 μg/mL LPS for 24 h. Thereafter, PGE2 production was measured using a PGE2 high sensitivity enzyme immunosorbent assay kit (Enzo, Japan). The absorbance of each sample well was measured at 405 nm using a microplate reader and inhibition of PGE2 production by the S. linearis extract was expressed as a percentage (%) of the LPS-stimulated group value.
The antibacterial activity of the S. linearis extract was tested against four reference microbial strains (S. aureus ATCC 6538P, B. cereus ATCC 14579, E. coli KCTC 2571, and S. typhimurium KCTC 1925) using the disc diffusion method and calculating the minimum inhibitory concentration (MIC) of the extract. A bacterial suspension (1 × 108 CFU/mL) was spread on Müeller Hinton Agar plates. An aliquot of S. linearis extract was then placed on the paper discs in the inoculated plates. The plates were allowed to stand for 15 min at room temperature, after which they were incubated at 35 ± 2°C for 16–18 h. The diameters of the inhibition zones were measured in millimeters and all tests were performed in triplicate. To evaluate the MIC of the S. linearis extract, the extract was diluted in each liquid medium, the concentration of the test bacteria was measured by suspending it at a concentration of 2–8 × 105 CFU/mL, and the test bacteria was serially diluted two-fold in Müeller Hinton broth. Thus, the concentration range was determined. After incubation at 35 ± 2°C for 16–20 h, the minimum concentration of algal extract at which no bacterial growth was observed was determined to be the MIC.
Results
The potential cytotoxicity of the S. linearis extract was measured in cell experiments, revealing that a concentration range of 10–100 μg dry weight extract/mL did not reduce the viability of LPS-stimulated RAW 264.7 cells (Fig. 1A). Therefore, a concentration range of 10–100 μg/mL extract was chosen for subsequent experiments. The IC50 values for DPPH and ABTS of the S. linearis extract were 34.57 and 11.70 μg/mL, respectively (Table 1). The inhibition of ROS production by the S. linearis extract was 12.8%, 38.2%, 49.3%, and 48.8% at 10, 25, 50, and 100 μg/mL, respectively (Fig. 1B). The S. linearis extract demonstrated greater antioxidant activity than the LPS-treated (control) group at all concentrations of extract.
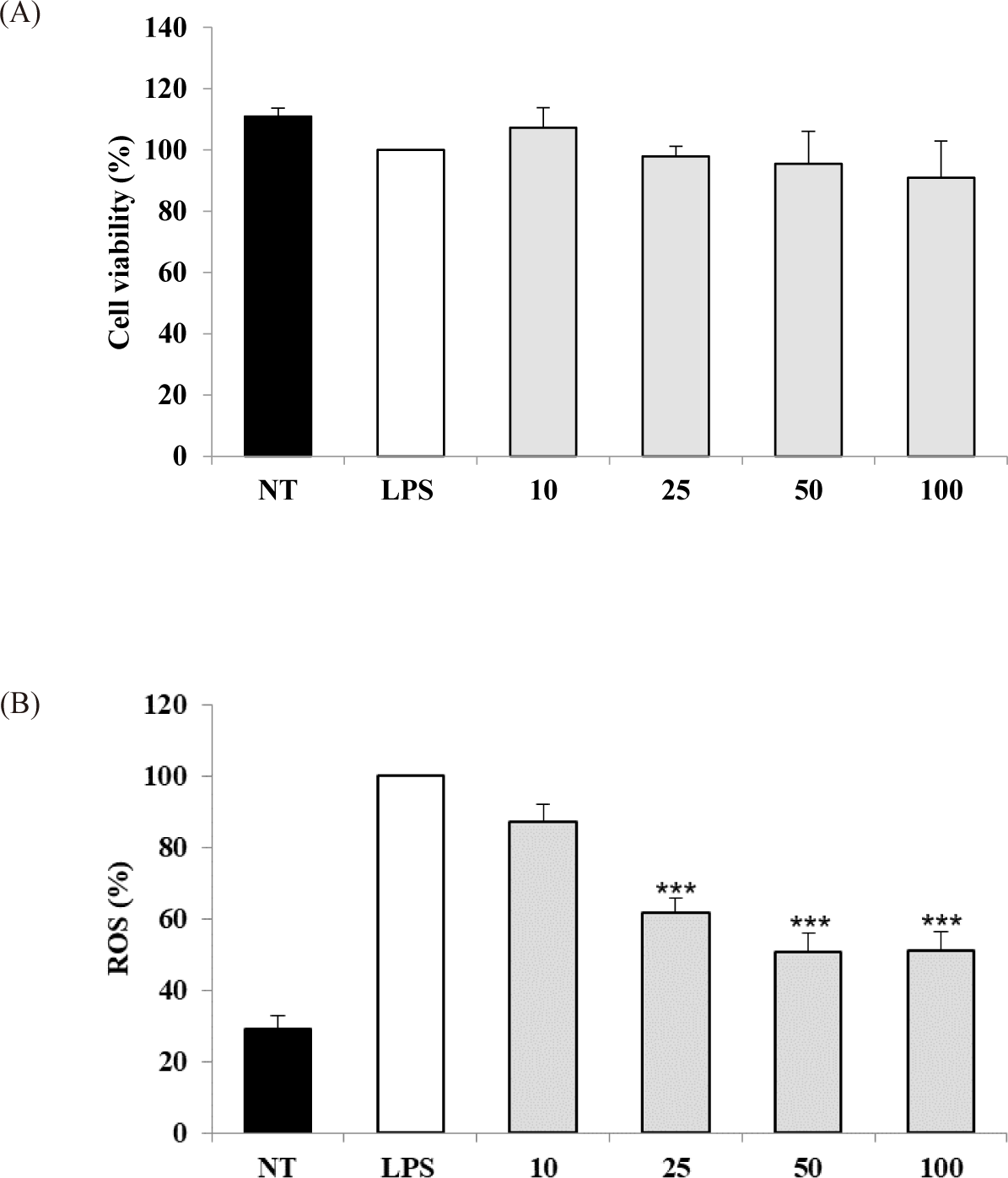
Sample | DPPH (IC50) | ABTS (IC50) |
---|---|---|
S. linearis extract (μg/mL) | 34.57 ± 0.361) | 11.70 ± 0.05 |
The anti-inflammatory activity of the S. linearis extract was evaluated based on inhibition of NO and PGE2 production in LPS-stimulated RAW 264.7 cells. S. linearis extract inhibited NO production by 10.0%, 42.1%, 61.1%, and 78.0% at 10, 25, 50, and 100 μg/mL extract, respectively (Fig. 2A). In turn, PGE2 production was inhibited 1.5%, 69.1%, 83.2%, and 94.8% at 10, 25, 50, and 100 μg/mL extract, respectively (Fig. 2B). The inhibitory effect of S. linearis extract at 100 μg/mL on PGE2 production (94.8%), in particular, is indicative of the strong anti-inflammatory effect of the extract.
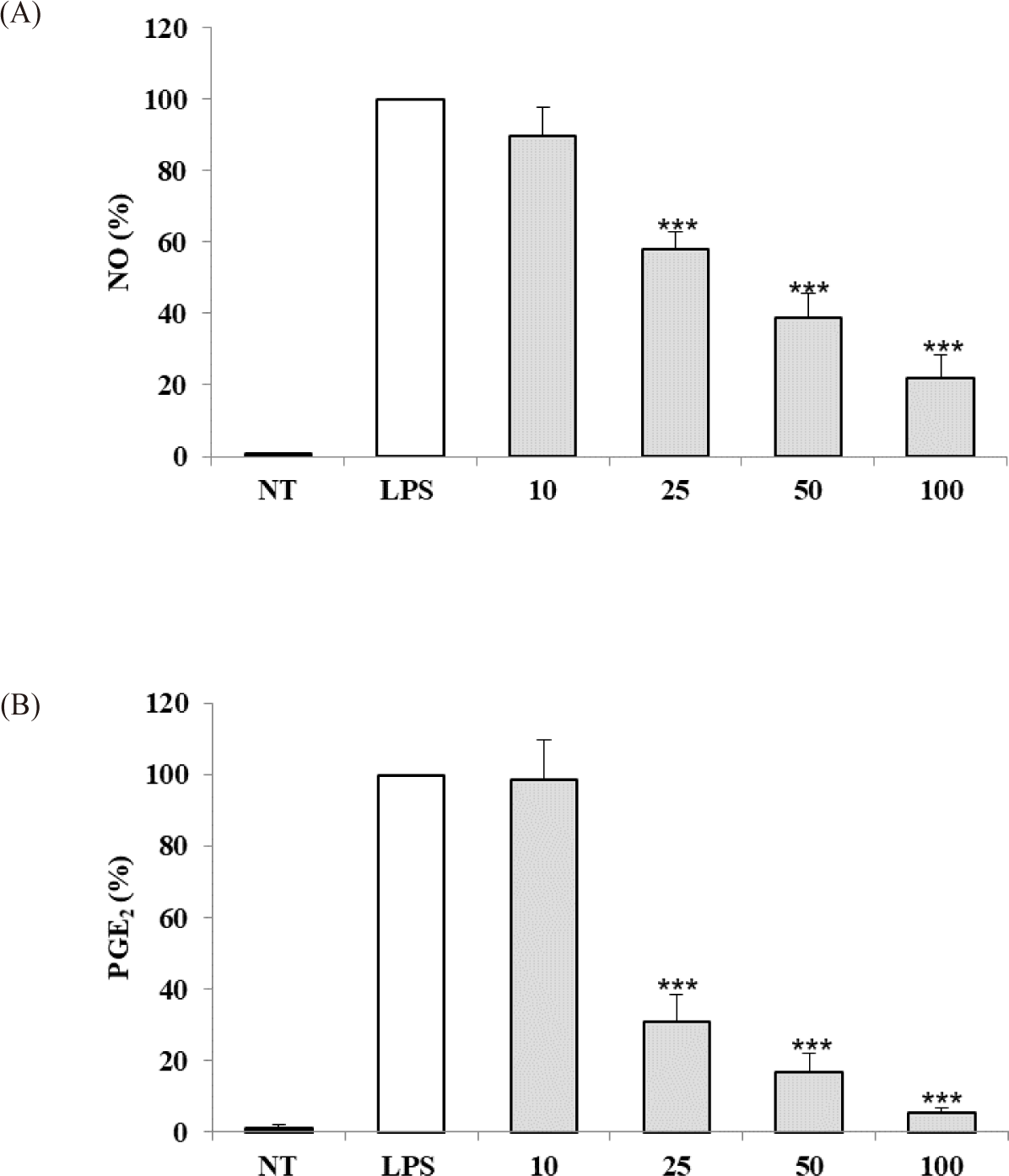
The S. linearis extract inhibition zones obtained using the disc diffusion method were 10 mm and 8 mm against S. aureus and B. cereus, and the MIC was 256 μg/mL and 1,024 μg/mL extract, respectively (Table 2). Our experimental results showed that the S. linearis extract had a stronger effect on gram-positive bacteria than on gram-negative bacteria.
Assay | Escherichia coli KCTC 2571 | Salmonella typhimurium KCTC 1925 | Staphylococcus aureus ATCC 6538P | Bacillus cereus ATCC 14579 |
---|---|---|---|---|
Paper disc diffusion (mm) | - | - | 10 | 8 |
MIC (μg/mL extract) | - | - | 256 | 1,024 |
Discussion
The existing literature on red algae includes reports on the biological activity (anti-diabetic, antioxidant, and antiviral) and chemical composition of S. latiuscula, which is registered and used as a food ingredient in Korea. The lesser-known species S. linearis was reported as a new species in 2013 by Kang & Kim (2013); however, to our knowledge, there have been no studies on its biological activity. Therefore, in this study we investigated the antioxidant, anti-inflammatory, and antibacterial activity of a 70% EtOH extract of S. linearis.
S. linearis extract exhibited weaker antioxidant activity (considering both DPPH and ABTS) than that previously reported the IC50 value for DPPH of S. latiuscula MeOH extract was 9.03 μg/mL and the ABTS radical scavenging effect of S. latiuscula EtOH extract was 3.8% at 60 μg/mL (Cho et al., 2012; Park et al., 1998). Additionally, inhibition of ROS production by the S. linearis extract (even when assayed at a high concentration of 100 μg/mL) was ≤ 50% (Fig. 1B), whereas inhibition by the S. latiuscula extract was 50.6% at 25 μg/mL (Kang et al., 2004). These results suggest that an equivalent extract of S. linearis has lower antioxidant activity than that of S. latiuscula extract.
In the assessment of the anti-inflammatory activity of S. linearis extract, increasing inhibition of NO and PGE2 production occurred in a concentration-dependent manner. The S. linearis extract inhibited NO production > 50% at a concentration of 50 μg/mL and that of PGE2 at a concentration of 25 μg/mL extract, showing that the extract exhibited high anti-inflammatory activity. PGE2 not only serves as a mediator of the inflammatory response, but it also promotes Th2-type immune responses, suppresses Th1-type immune responses, and suppresses the production of inflammatory cytokines such as tumor necrosis factor-α, interleukin (IL)-1β, and IL-8 and anti-inflammatory cytokines such as IL-10. PGE2 is known as an immune response modulator that promotes the production of cytokine (Harris et al., 2002). Our study, therefore, suggests that S. linearis could potentially be used in the prevention and treatment of inflammatory and immune diseases.
In the evaluation of the antibacterial activity of the S. linearis extract, we found that it was effective only against gram-positive bacteria (e.g., S. aureus and B. cereus), and was particularly effective against S. aureus (MIC of 256 μg/mL). S. aureus is one of the most common gram-positive bacteria that causes food poisoning in people (Rauha et al., 2000). In a previous study, a MeOH extract of S. latiuscula showed a MIC of 500 μg/mL extract for S. aureus and E. coli (Lim et al., 2000). It is difficult to compare the results because the extract types differed, MeOH vs. EtOH; however, it appears that S. linearis and S. latiuscula extracts exhibit similar antibacterial activity.
Based on our findings, we propose that a 70% EtOH extract of S. linearis has greater potential as an anti-inflammatory material than as an antioxidant or antibacterial material.
Conclusion
To the best of our knowledge, there have been no research studies on the biological activity of S. linearis; thus, we conducted experiments to assess the antioxidant, anti-inflammatory, and antibacterial properties of a 70% EtOH extract of S. linearis. Our results showed that S. linearis extract possesses strong anti-inflammatory properties, and therefore has the potential to be used in the prevention and treatment of inflammatory and immune diseases.