Introduction
The mandarin fish Siniperca scherzeri is a freshwater species with high commercial value found in East Asia. The fish is mainly distributed in North Vietnam, China and Korea (Li, 1991; Sankian et al., 2019; Zhang et al., 2009; Zhou et al., 1988). It is a temperate perch belonging to the family Percichthyidae (Li, 1991; Zhou et al., 1988). As natural resources have become depleted owing to overfishing and habitat destruction, S. scherzeri has drawn attention as a fish species that can be cultured in inland fisheries. Its promising prospects, such as rapid growth rate and high disease resistance, have generated high demands for methods for its culturing (Liang et al., 2005; Sankian et al., 2018; Sankian et al., 2019; Su et al., 2005; Zhang et al., 2009).
According the literature, the growth rate and feed efficiency (FE) of a farmed fish species depend on feed quality and quantity (Bureau et al., 2006; Yuan et al., 2010) and is affected by culture conditions, feed composition and type, and feeding method (Lee et al., 2000a; Lee et al., 2000b). An optimal feeding regimen can minimize feed waste and maximize FE and was thus crucial for farming of fish (Blanquet & Oliva-Teles, 2010; Cho et al., 2006; Van Ham et al., 2003; Xie et al., 2011). In general, the best feeding ratio was feeding just below satiation (Blanquet & Oliva-Teles, 2010; Cho et al., 2006; Cho et al., 2007; Eroldoğan et al., 2004; Meyer-Burgdorff et al., 1989; Shimeno et al., 1997; Van Ham et al., 2003; Zoccarato et al., 1994). Commercial fish farms preferred to use a restricted feeding regimen that did not inhibit fish growth; in brief, they preferred to use an optimal feeding ratio for financial and environmental reasons, such as high feed utilization, improved water quality, reduced labor cost, and enhanced product quality (Blanquet & Oliva-Teles, 2010; Cho et al., 2006; Eroldoğan et al., 2006; Li et al., 2005; Van Ham et al., 2003). However, the optimal feeding ratio depends on various factors, such as species, size, and culture conditions (Blanquet & Oliva-Teles, 2010; Cho et al., 2006; Cho et al., 2007; Van Ham et al., 2003).
Therefore, it was necessary to investigate the effect of feeding ratio on different farmed fish species and to decide whether a predetermined feeding ratio was realistic to use in fish farming. Regarding research on culturing S. scherzeri, studies had been conducted on the efficiency of live feed utilization (Liu et al., 1998), nutrient demand (Mo et al., 2019; Sankian et al., 2017; Sankian et al., 2019), efficiency of mealworms as a substitute for fish meal (Sankian et al., 2018) and feeding time (Zhang et al., 2009). However, to best of our knowledge, no study has investigated the optimal feeding ratio for S. scherzeri. The goal of this study was to investigate the effects of feeding ratio on the growth, blood chemistry, and body composition of juvenile S. scherzeri.
Materials and Methods
The mandarin fish Siniperca scherzeri bred at the Chungcheongbuk-do Inland Fisheries Research Institute were used in this experiment. The experiment was conducted in a recirculating culture system consisting of a nitrification filter tank (volume: 3,000 L), a foam separator (100 L), and 21 cylindrical fiberglass water tanks (300 L). Before the beginning of the feeding trial, all fish were acclimated to environmental culture condition for 2 weeks. Twenty fishes (an average initial body mass: 9.6 g ± 0.05 g) per tank were randomly distributed in each cylindrical water tank. Experimental diet was provided twice daily (09:00 h and 17:00 h) until satiation. The tank was aerated to supply sufficient levels of dissolved oxygen. During the experimental period, levels of dissolved oxygen, ammonia, nitrite, temperature, and pH were monitored daily. The water temperature was maintained at 26.1°C–27.7°C, dissolved oxygen level > 7.5 mg/L, ammonia < 0.45 mg/L, nitrite < 0.17 mg/L, and pH within 6.8–7.2 during the experimental period.
Seven feeding ratios were used in this experiment; 100% (satiation) for the control and 95%, 90%, 85%, 80%, 75%, and 70% of satiation for the experimental groups. The feed amount of each feeding treatment including the control group was followed by the method of Cho et al. (2006); the control group (100%) was fed twice daily until 100% satiation (09:00 h and 17:00 h). The satiation level was determined based on apparent visual satiety. The remaining six feeding ratios were determined based on the feed intake (FI) amount in the control group. During the experimental period, feed was carefully provided ensuring there was no feed leftover on the tank floor. Uneaten feed were siphoned from the each tank floor for 30 min after begin of feeding at each meal everyday. Once we measured the dried mass of siphoned food, we deducted from total feed consumption (FC) to calculate FI. In the six experimental groups, feed allowance of fish was re-adjusted everyday according to the FI of fish in the control group previous day. The experiment was conducted in triplicate and lasted 10 weeks.
The ingredients and chemical compositions of the experimental diet are shown in Table 1. The diet consisted of anchovy meal as the main protein source. In addition, squid liver oil and soybean oil was added in a 1:1 ratio as the main lipid sources (Sankian et al., 2017). These raw materials were mixed and an adequate amount of water was added to the mixture. The mixture was compressed into pellets (diameter: 3 mm). The compositions of experimental diet were 55.8% crude protein and 14.5% lipid, which meets the nutrient demands for growth of juvenile mandarin fish (Sankian et al., 2017). The pellets were dried at room temperature and were stored in a freezer at –30°C until they were used in the experiment.
Pesquera Bahia Caldera, Caldera, Chile. Fishmeal composition (% dry matter): crude protein, 67.3; crude lipid, 8.6.
WooSung Feed, Daejeon, Korea. Corn gluten meal composition (% dry matter): crude protein, 66.1; crude lipid 2.8.
Vitamin premix contained the following ingredients (g/kg premix), which were diluted in cellulose: thiamin hydrochloride, 2.7; riboflavin, 9.1; pyridoxine hydrochloride, 1.8; niacin, 36.4; Ca-D-pantothenate, 12.7; myo-inositol, 181.8; D-biotin, 0.27; folic acid, 0.68; p-aminobenzoic acid, 18.2; menadione, 1.8; retinyl acetate, 0.73; cholecalciferol, 0.003; and cyanocobalamin, 0.003.
The total length and weight of the fish were measured at the beginning and end of the experiment. Prior to weight measurement, all fish were starved for 24 h to remove any remaining metabolites from the gut and were anesthetized using 150 mg/L of 2-phenoxyethanol (Sigma, St. Louis, MO, USA). Ten fishes were selected randomly from each feeding treatment group, and their blood chemistry, hepatosomatic index (HSI), and viscerosomatic index (VSI) were measured. Blood samples were collected from the tail artery using a heparin-treated syringe after anesthetizing the fish with 150 mg/L of 2-phenoxyethanol for 1 minute. Hematocrit (HCT) was measured from whole blood, and serum was extracted by centrifuging the blood samples at 8870×g for 5 min. DRI-CHEM NX500i (Fujifilm, Tokyo, Japan) was used to measure serum total protein (TP), glutamic oxaloacetic transaminase (GOT), total cholesterol (TCHO), high-density lipoprotein cholesterol (HDLC), glutamic pyruvic transaminase (GPT), and glucose (GLU).
For analysis of the whole body composition, 10 fish specimens from each group were stored in a freezer at –40°C prior to analysis and then analyzed according to standard procedures (AOAC, 1995). Crude protein content was analyzed using the Auto Kjeldahl System (Buchi B-324/435/412, Switzerland; Metrohm 8-719/806, Switzerland) according to the Kjeldahl method. Moisture content was measured after drying the specimens in a dry oven at 105°C for 24 h . The crude lipid content was measured using the ether-extraction method, and the ash content was measured after burning the specimens for 4 h at 600°C.
One-way ANOVA was performed using SPSS 20.0 (SPSS, Chicago, IL, USA). Tukey’s HSD (honestly significant difference) test was used to examine the statistical significance of the mean values at 95% confidence intervals. The optimal feeding ratio was calculated using a broken-line model (Robbins et al., 1979).
Results
The survival rate, weight gain (WG), and specific growth rate (SGR) of S. scherzeri fed for 10 weeks at different feeding ratios are provided in Table 2. The feeding ratio did not affect the survival rates of the fish during the experimental period (p > 0.05). There were no significant (p > 0.05) differences in WG and SGR between the 100% satiation group and the 95%, 90%, 85%, and 80% satiation groups. However, the 100% satiation group showed significantly (p < 0.05) higher WGs and SGRs than the 75% and 70% satiation groups.
Table 3 shows FC, FI, FE, protein efficiency ratio (PER), and protein retention (PR) of the fish fed at different feeding ratios. The FC and FI of 100%, 95%, and 90% satiation groups were significantly higher than those of 85%, 80%, 75%, and 70% satiation group (p < 0.05), but there was no significant (p > 0.05) difference among 100%, 95%, and 90% satiation groups. Although there was no significant (p > 0.05) difference in PER and PR between the 100% satiation group and the 95% and 90% satiation groups, the 100% satiation group exhibited significantly (p > 0.05) lower PER compared with the 85%, 80%, 75%, and 70% satiation groups. The condition factor (CF), HSI, VSI, coefficient variation of body length (CVBL), and coefficient variation of body weight (CVBW) are presented in Table 4. The feeding ratio did not affect any of these parameters (p > 0.05). In a broken-line model analysis based on the WG of S. scherzeri at different feeding ratios, the optimal feeding ratio was found to be 87.7% of satiation (Fig. 1).
Values (mean of triplicates ± SE) in the same column not sharing a common superscript are significantly different (p < 0.05).
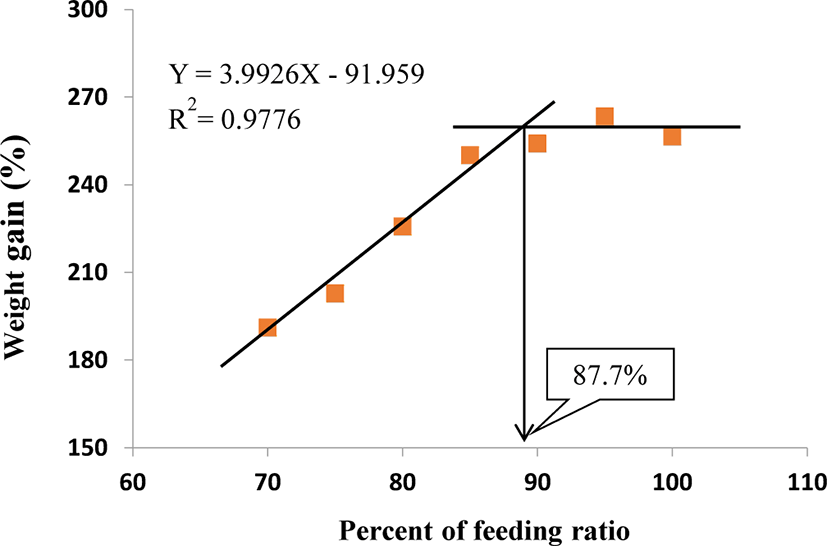
Proximate compositions of fish measured at the end of the experiment for each feeding ratio are shown in Table 5. Moisture, crude protein, crude lipid and crude ash contents of S. scherzeri were not significantly (p > 0.05) different according to the feeding ratio. The blood chemical contents of HCT, GLU, TP, TCHO, GOT, GPT, and HDLC in S. scherzeri fed various feeding ratio are presented in Table 6. The feeding ratio did not affect the contents of HCT, GLU, HDLC, GOT, TP, and GPT (p > 0.05). The 100% satiation group showed significantly (p < 0.05) higher TCHO contents compared with the 80%, 75%, and 70% satiation groups. However, the 100% satiation group did not show significant (p > 0.05) differences in TCHO levels compared with the 95%, 90%, and 85% satiation groups.
Discussion
In this study, reduction in the feeding ratio influenced the growth of S. scherzeri. A similar result was observed in some of the previous studies (Cho et al., 2006; Cho et al., 2007; Cleveland & Burr, 2011; Eroldoğan et al., 2004; Shimeno et al., 1997; Van Ham et al., 2003). Our results also showed that there were no differences in WG and SGR between the 100% satiation group and 80% satiation group (Table 2). The effect of feeding ratio on the fish growth was associated with the fish species and size. For instance, common carps (Cyprinus carpio) (Shimeno et al., 1997) and rainbow trouts (Oncorhynchus mykiss) (Cleveland & Burr, 2011) fed to 80% and 75% satiation, respectively, exhibited no significant differences in WG and SGR compared with fish from the same species fed to 100% satiation, whereas turbots (Scophthalmus maximus) (Blanquet & Oliva-Teles, 2010) exhibited a significant reduction in WG when fed to 90% satiation as opposed to being fed to 100% satiation. In their experiment on olive founders with initial body weights of 17–319 g, Cho et al. (2006) and Cho et al. (2007) reported no significant difference in WG and SGR between fish fed to 90% or 95% satiation and fish fed to 100% satiation and suggested that the optimal feeding ratio decreases as fish growth increases (Hatlen et al., 2005; Skalli et al., 2004; Sweilum et al., 2005).
The FE, PER, and PR in present study increased as the feeding ratio decreased whereas the FI showed opposite trends. Van Ham et al. (2003) reported increased FE and protein and energy retention in turbots fed to 65% satiation despite observing that the turbots had reduced growth compared with turbots fed to 100% satiation. Furthermore, Blanquet & Oliva-Teles (2010) reported that feeding efficiency marginally increased as the feeding ratio decreased. In general, feeding below satiation improved feeding efficiency, and indeed, this had been the case for striped bass (Cox & Coutant, 1981), minnow (Cui & Wootton, 1988), channel catfish (Ictalurus punctatus) (Li & Lovell, 1992; Xu et al., 2017), rainbow trout (Zoccarato et al., 1994), and yellowtail flounder (Limanda ferruginea) (Puvanendran et al., 2003). This was because increasing the feeding ratio reduces time for feed digestion and absorption in the intestines, thereby preventing efficient digestion (Henken et al., 1985; Liu & Liao, 1999). In contrast, studies have reported that the feeding ratio does not affect the FE, PER, and PR of olive founders (Cho et al., 2006; Cho et al., 2007) suggest that the effect of feeding ratio varies in different fish species.
The feeding ratio in this study was found not to affect CF, HSI, and VSI, which are used to evaluate the nutritional and physiological condition of fish (Eroldoğan et al., 2004; Mihelakakis et al., 2002). A similar results were previously reported for CF and HSI in oliver flounders (Cho et al., 2006; Cho et al., 2007) and for CF in European sea bass (Dicentrachus labrax) (Eroldoğan et al., 2004). However, it is known that the CF, HSI and VSI of fish are affected by feeding ratio or feeding rate according to many previous reports such as sea bass (Dicentrarchus labrax) (Hidalgo et al., 1987), striped bass (Morone saxatilis) (Hung et al., 1993), tropical bagrid catfish (Mystus nemurus) (Ng et al., 2000), and gilthead sea bream (Sparus aurata) (Mihelakakis et al., 2002), rainbow trout (Cleveland & Burr, 2011), and channel catfish (Xu et al., 2017). Eroldoğan et al. (2004) suggested that the HSI of European sea bass reared in seawater or freshwater decreased more than the VSI with feeding rate decrease, indicating that the liver is a more labile storage organ than viscera. In addition, since morphological indices such as CF and VSI can be affected not only feeding ratio but also by feed processing method (pelleting or extrusion) (Xu et al., 2017), future studies are required.
In this study, the feeding ratio did not affect the CVBW and CVBL. Similar results have been reported for tambaqui (Colossoma macropomum) (Silva et al., 2007) and turbots (Van Ham et al., 2003). Based on the results of this study, the feeding ratios of 70%–100% satiation promoted the growth of S. scherzeri weighing from 9.0 g to 37 g without affecting the CV in the body weight and total length. However, it has been reported in rock bream (Oplegnathus fasciatus) (Oh & Venmathi Maran, 2015) and in the hybrid sunfish (Wang et al., 1998) that an increase of diet allowance with feeding frequency can reduce size variation and produce fish of a uniform size.
There was no effect of the feeding ratio on the chemical composition (moisture, crude protein, lipid, and ash content) of S. scherzeri. Similar results had been reported for olive flounder (Cho et al., 2007). However, these results were inconsistent with previous reports that the lipid content increased as the feeding amount increases (Eroldoğan et al., 2004; Mihelakakis et al., 2002; Shimeno et al., 1997; Van Ham et al., 2003; Xu et al., 2017). Whole body composition of fish generally under low feeding ratio show the high protein content (Eroldoğan et al., 2004; Ng et al., 2000; Xu et al., 2017) and the low lipid content (Mihelakakis et al., 2002; Ng et al., 2000; Van Ham et al., 2003). Increase of body lipid content with increase feeding ratio was reported in previous studies such as tropical bagrid catfish (Ng et al., 2000), striped bass (Hung et al., 1993), sea bass (Eroldoğan et al., 2004), and channel catfish (Xu et al., 2017). Xu et al. (2017) reported that the decreased dietary protein ingested under a low feeding ratio is mainly used for tissue protein synthesis and maintenance other than energy metabolism, however increased dietary protein ingested under the high feeding ratio would be involved in energy metabolism and converted into lipid, resulting in a decrease in PR and an increase in body lipid content. In addition, the difference of body composition content such protein and lipid with diet allowance is also affected by temperature, salinity and feed composition, which demand further study in the future (Hidalgo et al., 1987; Xu et al., 2017).
Increasing the feeding ratio did not affect the blood HDLC, GLU, GOP, GPT, TP, and HCT contents of S. scherzeri but tended to increase the TCHO contents. Shimeno et al. (1997) reported similar results where the cholesterol, TP, GLU, triglycerides, and ammonia levels increased as the feeding ratio increased. Increasing the feeding ratio by increasing the feeding frequency led to elevated serum contents of TCHO in dark-banded rockfish (Sebastes inermis) (Oh et al., 2018). However, the feeding ratio does not affect serum GLU, TP, triglycerides, and GPT levels in olive flounder (Cho et al., 2006). It was also reported that reducing the feeding ratio can induce stress and increase serum GOP and GPT levels in fish (Mizanur & Bai, 2014; Okorie et al., 2013). Further studies are necessary to clarify such discrepancies.
The feeding ratio significantly affected the growth, body composition, and blood chemistry of juvenile mandarin fish. Our results showed that an optimal daily feeding ration for growth of juvenile mandarin fish weighing from 9.0 to 37 g was 87.7% of satiation using a broken-line analysis based on the feeding efficiency observed at feeding ratios of 100%–70% and WG (Fig. 1) under 26.1°C–27.7°C condition. Overall, the utilization efficiency of protein and feed were important parameters in the determination of improved growth performance by the restricted feeding ratio from 100% to 70% of satiation. These findings provide important information for aquaculturists trying to establish a profitable feeding strategy for optimizing the growth of juvenile mandarin fish.