Introduction
Annual aquaculture production of abalone has kept increasing globally because of high demand for human consumption (FAO, 2016). Since macroalgae (Undaria pinnatifida, Saccharina japonica and Eisenia bicyclis) commonly used for abalone culture in Korea and China are likely to be deficient in nutrient, such as protein (amino acid [AA]) and lipid (fatty acids) as abalone feed (Ansary et al., 2019; Jang et al., 2018; Mai et al., 1996; Myung et al., 2016; Uki et al., 1986a, 1986b), development of artificially formulated diet is highly needed for stable aquaculture production of abalone.
Determining dietary protein requirement of abalone is very important to produce a practical diet because protein is an expensive component in formulating abalone feed. Mai et al. (1995) demonstrated that protein requirements of two species of juvenile (initial weight of 0.38 and 0.18 g) abalone (H. discus hannai and H. tuberculata) were estimated to be 25%–35% and 35%, respecitvley, for their maximum growth when one of the combined casein and gelatin-basal diets containing 0%–50% protein levels was fed to abalone. In the earlier study of Uki et al. (1986a), however, dietary protein requirement was reported to be 20%–30% for growth rate, feed conversion ratio and protein retention of juvenile (initial weight of 3.5 g) abalone (H. discus hannai), but the maximum growth rate was obtained in abalone fed the casein-basal diet containing 46.5% crude protein or white fish meal-basal diet containing 43.1% crude protein.
Generally speaking, the smaller or younger fish require the higher dietary protein requirements than the larger or older one (Einen & Roem, 1997; NRC, 1993; Sweilum et al., 2005; Wilson & Halver, 1986). Stone et al. (2013) reported that optimum dietary protein level for 1-year (initial weight of 1.8 g) and 2-year old (initial weight of 22.9 g) greenlip abalone (H. laevigata), known as preferred temperature condition of 18.3°C for growth (Gilroy & Edwards, 1998), were 29% and 24% at 14°C, but 35% and 34% at 22°C, respectively. On the contrary, Britz & Hecht (1997) reported that the small abalone (H. midae) grown from 0.2 g to 1.0 g responded better on a 34% protein diet, but the large one grown from 7.0 g to 14.0 g responded better on a 44% protein diet when two sizes of abalone were fed with one of diets containing three (24%, 34%, and 44%) protein levels at 18°C. Therefore, the optimum dietary protein requirements of abalone depending on abalone size are still controversial and need to clarify.
Dietary protein requirements of abalone (Haliotis discus, Reeve) depending on abalone size were evaluated and compared in this study.
Methods
The similar size of small and large groups were bought from local abalone farms and moved to Ocean and Fisheries Research Institute (Jeju Special Self-Governing Province, Jeju, Korea). Abalone were acclimated to the experimental conditions for 2 weeks and fed with the dry Undaria at the ratio of ca. 2.0%–3.5% biomass daily before initiating the feeding trial.
One thousand and fifty small abalone [initial weight of 2.7 g ± 0.01 g (mean ± SE)] were distributed into each of 15, 70 L plastic rectangular containers (120 cm × 36 cm) (seventy abalone/container). Five containers receiving different 5 feeds were placed into each of three 10 ton concrete raceway tanks (water volume: 2.8 ton) at a flow rate of 48.2 L/min/raceway. The sand-filtered seawater was continuously supplied throughout the 16-week feeding trial and water temperature ranged from 14.7°C to 17.2°C (mean ± SD: 16.6 ± 0.52°C).
Aeration was properly supplied in each raceway and photoperiod followed natural conditions. The experimental diets were daily (1,700 h) fed to triplicate groups of abalone at a quantity to ensure satiation (ca. 2.0%–3.5% of total biomass) with a small amount remaining. Dead abalone were daily eliminated and the bottoms of the containers were daily cleaned. At the end of the both 16-week feeding trial, all surviving abalone were harvested from each container and collectively weighed.
Five hundred forty large abalone [initial weight of 16.0 g ± 0.04 g (mean ± SE)] were distributed into each of 18, 70 L plastic rectangular containers (thirty abalone/container). Six containers receiving different 6 feeds were placed into each of three 10 ton concrete raceway tanks (water volume: 2.8 ton) at a flow rate of 110 L/min/raceway. The sand-filtered seawater was continuously supplied throughout the 16-week feeding trial and water temperature ranged from 16.8°C to 20.7°C (mean ± SD: 18.0 ± 0.71°C). Other experimental conditions, except for abalone and water temperature were same as Trial 1.
The authors confirm that the ethical policies of the FAS, as noted on the journal’s author guidelines, have been adhered to. No ethical approval was required for this study.
Five and six experimental diets containing crude protein level from 20% to 40% and 20% to 45% with 5% increment of crude protein at the expense of dextrin for small (Trial 1) and large abalone (Trial 2) were prepared and referred to as the CP20, CP25, CP30, CP35, CP40, and CP45 diets, respectively (Table 1). Fish meal, defatted soybean meal and casein were used as protein source in the experimental diets. Dextrin and squid liver and soybean oils were used as carbohydrate and lipid sources, respectively, in the experimental diets. The experimental diets are isocaloric at 14.8 kJ/g diet.
Mineral mix contained the following ingredients (g/kg mix): NaCl, 10, MgSO4 · 7H2O, 150; NaH2PO4 · 2H2O, 250; KH2PO4, 320; CaH4 (PO4)2 · H2O, 200; Ferric citrate, 25; ZnSO4 · 7H2O, 4; Ca-lactate, 38.5; CuCl, 0.3; AlCl3 · 6H2O, 0.15; KIO3, 0.03; Na2Se2O3, 0.01; MnSO4 · H2O, 2; CoCl2 · 6H2O, 0.1.
Vitamin mix contained the following amount which were diluted in cellulose (g/kg mix): L-ascorbic acid, 200; α-tocopheryl acetate, 20; thiamin hydrochloride, 5; riboflavin, 8; pyridoxine, 2; niacin, 40; Ca-D-pantothenate, 12; myo-inositol, 200; D-biotin, 0.4; folic acid, 1.5; p-amino benzoic acid, 20; K3, 4; A, 1.5; D3, 0.003; choline chloride, 200; cyanocobalamin, 0.003.
Estimated energy (kJ/g diet) based on 16.8 kJ/g for protein and carbohydrate, and 37.8 kJ/g for lipid (Garling & Wilson, 1976).
All ingredients were mechanically mixed well after 22% sodium alginate was added, and then water was added at a ratio of 1:1. A paste was made from each of the diets by using an electronic mixer (Samwoo Industry, Daegu, Korea) and shaped into 0.15 cm thick sheets, which were then cut by hand into 1 cm2 flakes. The flakes were then dipped into an aqueous solution of 5% CaCl2 for 1 minute and then dried in a convection oven at 35°C for 2 days. They were stored frozen at –20°C until use.
Weight gain (g/abalone) of abalone was calculated as follows: Final weight of abalone – Initial weight of abalone. Specific growth rate (SGR, %/day) was calculated using the formula of Britz (1996b): SGR = [(Ln (final weight of abalone) – Ln (Initial weight of abalone) / Days of feeding] × 100. Weight gain used for regression analysis was calculated as follows: weight gain (%) of abalone = [(Final weight of abalone – Initial weight of abalone) / Initial weight of abalone)] × 100.
At the end of 16-week feeding trial, 20 abalone from each container in the small abalone study (Trial 1) were sampled and frozen at –20°C for the chemical analysis. The experimental diets and all separated carcass of abalone from each container were homogenized for the chemical analysis based on the standard method of AOAC (1990); moisture content determined by drying at 105°C for 24 h, crude protein content determined by Kjeldahl method (Buchi B-324/435/412; Auto Kjeldahl System, Flawil, Switzerland), crude lipid content determined by an ether-extraction method and ash determined by using a muffle furnace at 550°C for 4 h.
The AA composition of the experimental diets (Trial 2) was determined by using a high speed AA analyzer (Hitachi L-8800, Hitachi, Tokyo, Japan) after which the samples were hydrolyzed in 6 N HCl for 24 h at 110°C.
One-way ANOVA and Duncan`s multiple range test (Duncan, 1955) were used to determine the significance of the differences among the means of treatments by using SAS version 9.3 program (SAS Institute, Cary, NC, USA). Regression analysis was applied to evaluate dietary protein requirements of the small and large sizes of abalone (Lovell, 1989).
Results
AA profiles of the experimental diets (Trial 2) are presented in Table 2. All essential and non-essential AA of the experimental diets were proportionally increased with dietary protein levels. Leucine and glutamic acid were the richest essential and non-essential AA in the experimental diets, respectively.
Survival and weight gain of the small abalone was not significantly (p > 0.05) influenced by dietary protein level (Table 3). However, SGR of the small abalone fed the CP20 diet was statistically (p < 0.05) lower than that of abalone fed all other diets. Dietary protein requirement for the small abalone was estimated to be approximately 33.0% based on the 2nd polynomial analysis (weight gain vs dietary protein level) (Fig. 1).
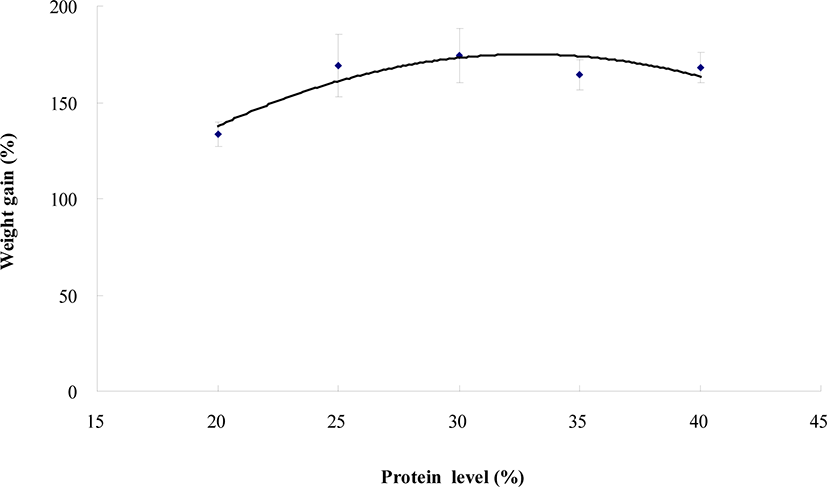
Survival of the large abalone was not significantly (p > 0.05) influenced by dietary protein level (Table 4). However, weight gain and SGR of the large abalone fed the CP30, CP35, and CP40 diets were statistically (p < 0.05) greater than those of abalone fed the CP20, CP25, and CP45 diets. The greatest weight gain and SGR were obtained in large abalone fed the CP30 diet, but lowest ones for the CP20 diet. Dietary protein requirement for the large abalone was estimated to be approximately 33.5% based on the 2nd polynomial analysis (weight gain vs dietary protein level) (Fig. 2).
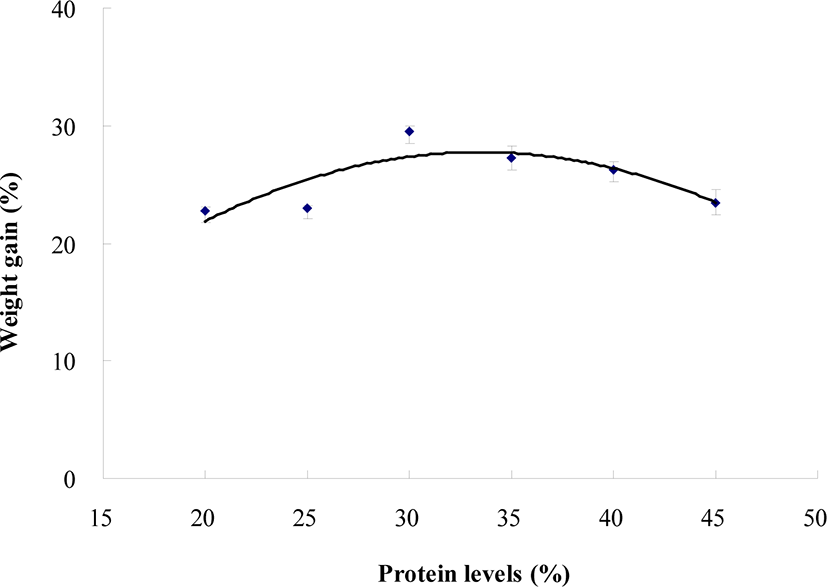
However, moisture, crude lipid and ash content of the carcass of the small abalone was not statistically (p > 0.05) affected by dietary protein level in Trial 1 (Table 5). However, crude protein content of the carcass of the small abalone increased with dietary protein level.
Discussion
The essential and non-essential AA content in the experimental diets increased with dietary protein level. Many researchers (Bautista-Teruel et al., 2003; Cho, 2010; Cho et al., 2008; Shipton & Britz, 2001) reported that essential AA, such as lysine, methionine and histidine are limiting factors to determine substitutability of the alternative animal and/or plant protein sources for fish meal in abalone feed or compare formulated diets with macroalgae for growth of abalone. Mai et al. (1994) also compared AA profiles of 6 species of macroalgae (Laminaria saccharina, L. digitata, Ulva lactuca, Chondrus crispus, Palmaria palmate and Alaria esculenta) commonly used as natural feeds for 2 species abalone (H. tuberculata and H. discus hannai) and concluded that arginine could be limiting factor in all macroalgae tested in abalone, but methionine, threonine or histidine might be also another limiting factor in some macroalgae.
The greatest weight gain and SGR were obtained in abalone fed the CP30 diet regardless abalone size in this study. Since temperature condition is not quite different for both small (mean temperature of 16.6°C) and large abalone (mean temperature of 18.0°C) in this study, it did not influence either growth performance of abalone or availability dietary optimum protein level. No distinctive difference in dietary protein requirements for the small and large abalone in this study is in consistent with Stone et al. (2013)’s study showing that the optimal dietary protein levels for 1- and 2-year old greenlip abalone (H. laevigata) were 35% and 34% at 22°C, respectively when abalone were fed with one of four protein levels (24%, 27%, 31%, and 37%) of diets containing solvent-extracted soybean meal, lupins and salmon fish meal as protein source at 14°C, 18°C, and 22°C for 12 weeks. However, there are also some conflicting studies showing that optimal protein level for 1-year old abalone was 29%, on the other hand, 24% for 2-year old one at 14°C (Stone et al., 2013) or that large (initial weight of 7 g) abalone (H. midae) grew better on a 44% protein diet, on the other hand, small (initial weight of 0.2 g) one grew better on a 34% protein diet (Britz and Hecht, 1997). Generally speaking, the smaller or younger fish require the higher dietary protein levels than the larger or older one (Einen & Roem, 1997; NRC, 1993; Sweilum et al., 2005; Wilson & Halver, 1986).
Mai et al. (1995) reported dietary protein level of 35% for maximum growth of abalone and 25%–35% for requirements when the mixture of casein and gelatin-basal diets containing various protein levels (0%–50%) were fed to two species of juvenile abalone (H. discus hannai) grown from 0.38 g to 0.97 g and (H. tuberculata) grown from 0.18 g to 0.55 g, respectively, at 13°C–15°C. Uki et al. (1986a) also demonstrated that dietary protein requirement was 20%–30% based on growth rate, feed conversion ratio and protein retention when the casein- and white fish meal-basal diet was fed to juvenile abalone (H. discus hannai) grown from 3.5 g to 4.8 g, however, the greatest growth rate was achieved in abalone fed the casein-basal diet containing 46.5% crude protein or white fish meal-basal diet containing 43.1% crude protein at 19.7°C. Similarly, Coote et al. (2000) showed that the greatest SGR was obtained in abalone (H. laevigata) fed a 29.9% protein diet when abalone were fed with one of diets containing protein levels of 12.0% to 46.1% and reported dietary protein requirement of 27%. Sales et al. (2003) reported that dietary protein requirement was estimated to be 25.5% based on broken-line analysis for weight gain of juvenile South African abalone (H. midae), but the greatest weight gain was achieved in abalone fed a 39.4% protein diet when diets containing various protein levels (5.5%, 13.4%, 21.8%, 28.6%, 39.4%, and 47.9%) were fed to abalone for 95 days.
The smaller abalone achieved the higher SGR than larger one in this study, being consistent with other studies (Britz & Hecht, 1997; Stone et al., 2013) showing that the smaller abalone grew faster than the larger one at the same temperature condition.
Crude protein content of the carcass of the small abalone was well reflected from dietary protein content. Similarly, proximate of the carcass of abalone was well reflected from dietary nutrient composition (Garcia-Esquivel & Felbeck, 2009; Jang et al., 2018; Myung et al., 2016; Sales et al., 2003; Thongrod et al., 2003; Uki et al., 1986a).