Introduction
The giant trevally (Caranx ignobilis) is a large marine fish species belonging to the family Carangidae. It is widely distributed throughout the tropical and subtropical waters of the Pacific and Indian Oceans (Abdussamad et al., 2008). Giant trevally are predatory fish, feeding on smaller fishes, crustaceans, and mollusks, inhabiting depths of up to 170 m and exhibiting impressive schooling behavior (Abdussamad et al., 2008). Due to their high economic value, fast growth rate, and palatable flesh, giant trevally have become a popular aquaculture species in the Asia-Pacific region (Honebrink, 2000; Nguyen et al., 2022). Successful hatchery production of giant trevally has been achieved in several countries, including Vietnam (Mutia et al., 2020; Nguyen et al., 2022). In countries such as Taiwan, China, Indonesia, the Philippines, and Vietnam, giant trevally are commonly cultured in earthen ponds, concrete tanks, and cage systems (Abdussamad et al., 2008; Mutia et al., 2020; Nguyen et al., 2022). To date, research on giant trevally in the wild has mainly focused on biological characteristics, distribution, and stock assessment (Abdussamad et al., 2008). In contrast, studies on the captive culture of giant trevally are still relatively limited, with only a few addressing nutritional requirements and stocking density (Mutia et al., 2020; Nguyen et al., 2022). Research on the optimization of culture conditions, in general, remains scarce. The physical factors of the rearing environment, such as light, tank color, and tank material, have been shown to influence the physiology and biochemistry of fish, but have not been adequately investigated.
In recent years, the influence of color on the growth and coloration of fish has attracted the attention of researchers in evolutionary biology, behavior, cell biology, molecular biology, and aquaculture (McLean, 2021). The color of the rearing system, including tank color and light, has been shown to significantly affect the physiology and behavior of fish, thereby influencing their growth, survival, and overall health (Dopeikar et al., 2024; McLean, 2021). Tank color is an easily modifiable environmental factor with potential applications in fish farming, particularly for species with striking coloration (Dopeikar et al., 2024; McLean, 2021). Previous studies have demonstrated that tank color can affect stress response, immunity, and various other physiological and behavioral activities in fish (Morshedi et al., 2022; Ruchin, 2020). Tank color can also influence food recognition and feed intake, thereby impacting growth and survival rates (McLean, 2021; Strand et al., 2007). Furthermore, the color of the rearing system can affect the coloration of fish, an important quality attribute for consumer appeal and marketability, especially for species with vibrant colors (McLean, 2021; Ninwichian et al., 2022). Additionally, tank color may have indirect effects on body biochemical composition, including protein and lipid deposition, by influencing feeding behavior, digestive efficiency, and nutrient absorption from the diet (Mapunda et al., 2021; Okomoda et al., 2017; Papoutsoglou et al., 2000).
Numerous studies have shown that the effects of tank color on various performance indicators in fish culture vary depending on species, developmental stage, diet color, light regime, visual adaptation, and domestication level (El-Sayed & El-Ghobashy, 2011; Eslamloo et al., 2015; McLean, 2021; Padhi et al., 2022). White has been identified as the optimal color for the growth of goldfish (Carassius auratus) (Eslamloo et al., 2015), while red is preferred by Oscar fish (Astronotus ocellatus) (Dopeikar et al., 2024) and yellow is suitable for milkfish (Chanos chanos) (Bera et al., 2019). Striped gourami (Trichogaster pectoralis) exhibit better growth performance in blue tanks (Ninwichian et al., 2018), whereas black has been determined to be the appropriate color for Asian catfish (Clarias magur) (Ferosekhan et al., 2020). These findings suggest that choosing the appropriate tank color can significantly improve growth parameters, survival rates, and other indicators in certain fish species and should be considered when establishing aquaculture systems (McLean, 2021; Morshedi et al., 2022). However, research on the influence of tank color on giant trevally is still very limited. Therefore, evaluating the effects of tank color on giant trevally is crucial for developing and refining culture protocols for this economically valuable species. In this study, five tank colors, namely white, blue, red, yellow, and dark grey, were tested to assess their impact on growth, survival, feed utilization efficiency, coloration, and body biochemical composition of giant trevally. We hypothesized that the response of giant trevally to tank color would vary, and that certain colors would yield better results than others. This study is one of the first to investigate the effects of tank color on giant trevally. The findings will provide scientific evidence for selecting appropriate tank colors to enhance productivity, welfare, and economic efficiency in the culture of this species.
Materials and Methods
Giant trevally (C. ignobilis) is not listed as an endangered, critically endangered, or threatened and rare species under the National Regulations on the Use of Animals in Research in Vietnam: Vietnam Livestock Law 2018 and Government Decree 32/2006/ND-CP. Therefore, ethical approval for conducting research experiments on this fish species is not required in Vietnam. However, during the implementation process, best practices for the use of animals in research were applied to minimize the impact on the experimental fish.
The experiment was conducted at the Duong De Marine Fish Seed Production Station, Nha Trang City, Khanh Hoa Province, Vietnam. The fish were reared in 15 cylindrical and conical-bottomed composite tanks. Each tank had a volume of 100 L (diameter 70 cm, tank wall height 30 cm, total height 80 cm). The tanks were manufactured by the Institute of Marine Engine and Ship Research and Development, Nha Trang University, Vietnam. To evaluate the impact of tank color on giant trevally, we painted the inner surface of the tanks with Chokwang Vina Composite Epoxy paint according to the JOTUN RAL standard color chart (red color code: RAL 3000 – flame red; blue color code: RAL 5012 - light blue; yellow color code: RAL 1003 - signal yellow; grey color code: RAL 9023 - dark grey; white color code: RAL 9003 - signal white).
The experimental tanks were filled with seawater pumped directly from the sea and then treated with 20 ppm chlorine for 24 hours. Seawater was neutralized with sodium thiosulfate before use. Each rearing tank was equipped with an aeration nozzle with foam stones and ceramic stones placed at the bottom of the tank to provide optimal oxygen conditions. To assess the impact of tank color on giant trevally, a continuous lighting regime (light/dark: 24/0 h was applied). Light intensity was controlled within the range of 1,200–1,600 Lux with natural light during the day and fluorescent lamps at night (Mapunda et al., 2021).
The juvenile giant trevally used for the experiment were artificially produced at the Duong De Marine Fish Seed Production Station. Healthy and of uniform-sized juvenile giant trevally (average initial length of 1.91 ± 0.03 cm and initial weight of 0.24 ± 0.04 g) were stocked at a density of 1 fish per liter, corresponding to 70 fish per tank in five experimental tanks: white, blue, red, yellow, and dark grey in triplicate and reared for 28 days. The fish were acclimated to the rearing tanks for one week before the start of the experimental period.
The fish were fed a commercial diet, KAIO (Marubeni Nisshin Feed, Tokyo, Japan), with a particle size of 500–600 µm. The nutritional composition as declared by the manufacturer is: crude protein > 50.0%, crude lipid > 6.0%, crude fiber < 3.0%, crude ash < 15%, and moisture 10%. The fish were fed four times a day: 7:00 AM, 10:00 AM, 1:00 PM, and 4:00 PM. During feeding, the amount of food was adjusted according to the demand of the fish to minimize excess. The rearing tanks were siphoned and water was exchanged twice a day (7:00 AM and 5:00 PM), with 50% of the total water volume in the tank being removed and replaced.
Environmental factors were monitored and maintained within a suitable range for the growth and development of giant trevally. Specifically, the temperature ranged from 28°C–30°C, pH 7.8–8.2, dissolved oxygen > 5.0 mg/L, and total ammonia nitrogen (TAN) < 1.5 mg/L.
At the end of the experiment (day 28), the fish were fasted for 24 hours to ensure complete evacuation of the digestive tract. Thirty fish from each tank were randomly collected and mildly anesthetized with 0.05% Ethylene Glycol Monophenyl Ether (Merck KGaA, Darmstadt, Germany) to ensure accuracy in measuring length, weight, and skin color parameters (L*, a*, b*). Subsequently, seven fish from each tank were randomly sampled and stored at –80°C for further analysis of total carotenoid content accumulated in the skin and proximate biochemical composition of the whole body.
The total length (TL, cm) and total weight (BW, g) of fish at the beginning and end of the experiment were determined by randomly measuring and weighing 30 fish per tank. A plastic ruler (Thien Long Group, Ho Chi Minh City, Vietnam) and a digital scale (VNS LCD-A, Viet Nhat Electronic Scale, Ho Chi Minh City, Vietnam) with accuracies of 1.0 mm and 0.01 g, respectively, were used for measurements. The growth parameters were calculated using the following formulas:
Survival rate:
The survival rate was determined at the end of the experiment by counting the total number of surviving fish and dividing it by the initial number of fish stocked. It was calculated using the following formula:
The feed utilization efficiency parameters were calculated based on the actual amount of feed consumed by the fish (initial amount minus the amount of uneaten feed siphoned out and dried). After 30 min of feeding, uneaten feed was siphoned out and stored in a freezer. The total amount of uneaten feed was dried to the original feed moisture content of 10%. The parameters used to evaluate feed utilization efficiency included daily feed intake (FI, g/fish/day), FCR, FE, and PE. The detailed formulas are as follows:
where: L1, L2 are the total lengths (cm) and W1, W2 are the total weights (g) of fish at the beginning and end of the experiment, respectively. t is the experimental duration (28 days). SDL2, SDW2 are the standard deviations of length and weight of fish at the end of the experiment. N1, N2 are the initial number of fish stocked and the number of fish remaining (individuals) at the end of the experiment. FI is the mass of feed used (g). P is the protein content of the feed (50%).
Immediately after measuring length and weight, the skin color of fish was measured using a digital colorimeter (CR-400 Chroma Meter, Konica Minolta, Tokyo, Japan). Fish were measured at three locations on the body: the back, the belly, and the tail (Fig. 1). Each location was measured twice, corresponding to the two sides of the body, and the average value of the L*, a*, and b* indices at each location was recorded. The CR-400 meter was set to perform absolute color measurements in L*, a*, and b* (CIE 1976) mode, using L* to measure darkness (0) to lightness (100), a* to measure green (a–) to red (a+) pigments, and b* to measure blue (b–) to yellow (b+) pigments (Hunter & Harold, 1987). The measurement method followed the manufacturer’s instructions (Konica Minolta).
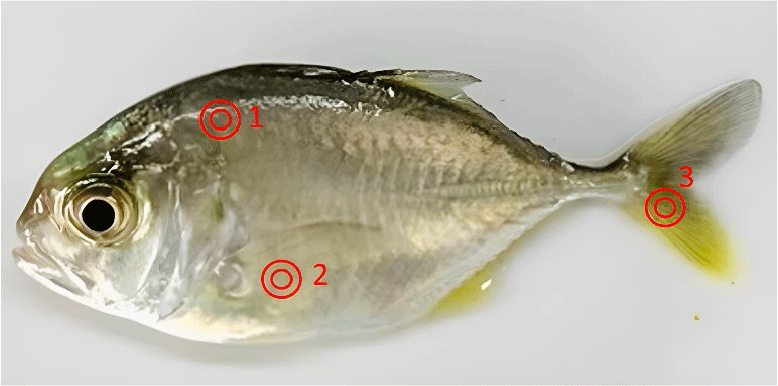
To determine the total accumulated carotenoid content in fish skin, three fish from each tank were collected immediately after skin color measurement and stored at –80°C for further analysis. The sampling method and analytical procedure were performed according to Tran et al. (Tran et al., 2022). Briefly, fish skin samples (0.5 g/sample) were collected from both sides of the body, then homogenized in acetone (20 mL) containing 1.5 g of anhydrous Na2SO4 using a homogenizer (T10 basic Ultra-turrax®, IKA Works, Staufen, Germany). The sample was then filtered through filter paper three times until the filtrate became colorless. The filtrate was centrifuged (7,920×g, 7 min) to collect the supernatant and kept at 4°C. The absorbance of the carotenoid-containing extract was measured using a spectrophotometer (Biochrom, Cambridge, UK) at a wavelength of 485 nm. The results were expressed as µg carotenoids/g sample (µg/g) and calculated using the formula:
where: A is the absorbance; V is the total volume of the extract (mL); D is the dilution factor; W is the sample weight (g); and E1cm1% is the conversion factor, 2,100 for edible oil solvent.
For the analysis of the proximate biochemical composition of fish, four fish from each tank were randomly collected and stored similarly to the total carotenoid analysis. Whole fish samples were used for the proximate composition analysis. The proximate biochemical parameters of the fish samples, including crude protein, crude lipid, ash, and moisture content, were analyzed according to the methods of the Association of Official Analytical Chemists (AOAC, 2006). Crude protein content was analyzed by the Kjeldahl method (N × 6.25) involving digestion with concentrated sulfuric acid and titration of the resulting ammonia. The Soxhlet method was used to determine the crude lipid content by extracting the samples with hexane solvent. Ash content was determined by incinerating the samples in a muffle furnace at 600°C for 6 hours. Moisture content was determined by drying the samples in an oven at 105°C to constant weight.
After data collection, the mean values were calculated using Microsoft Excel 2021 software (Microsoft, Redmond, WA, USA) before performing statistical analyses using SPSS 22.0 software (IBM, Armonk, NY, USA). Data were tested for normal distribution and homogeneity of variances before statistical analysis, using one-way ANOVA. Duncan’s test was used to determine significant differences between treatments at a significance level of p < 0.05. Data are presented as Mean ± SE.
Results
The growth and survival parameters of juvenile giant trevally reared in different tank colors are shown in Table 1. The results indicated that tank color significantly influenced the growth performance of fish in terms of length and weight. Specifically, fish reared in red tanks achieved the highest final length and weight (6.29 ± 0.10 cm and 3.49 ± 0.11 g), while the lowest values were found in fish reared in white tanks (5.60 ± 0.06 cm and 2.69 ± 0.13 g) (p < 0.05). Similar trends were observed for the other two growth parameters, specific growth rate in length and weight (p < 0.05). Notably, there were no significant differences in growth performance between fish reared in red and yellow tanks, suggesting that these two colors are suitable for the growth of juvenile giant trevally (p > 0.05).
Tank color also affected the survival rate of juvenile giant trevally, but the trend was opposite to that of growth parameters. Fish reared in dark grey and yellow tanks had the highest survival rates (77.14 ± 2.18% and 74.76 ± 4.15%), while fish reared in red tanks had the lowest survival rate (56.67 ± 2.65%) (p < 0.05).
The effects of tank color on the feed utilization efficiency parameters of juvenile giant trevally are presented in Table 2. Daily feeding rate was highest in fish reared in dark grey tanks (2.26 ± 0.06% BW/day), while feed conversion ratio and feed efficiency were best in yellow tanks (0.93 ± 0.02 and 1.07 ± 0.02, respectively). The poorest values were found in white and blue tanks. However, tank color did not affect the protein efficiency of juvenile giant trevally. Notably, there were no significant differences between fish reared in yellow and dark grey tanks for all the above-mentioned feed utilization efficiency parameters (p > 0.05), indicating that these two tank colors are suitable for enhancing feed utilization efficiency in this species.
Tank color had a pronounced effect on the skin color parameters of fish, both on the back, belly, and tail (Table 3). Analysis of color indices (L*, a*, b*) in different body regions (back, belly, and caudal fin) of juvenile giant trevally reared in different colored tanks revealed significant differences in color between body regions and among treatment groups.
Fish reared in white and yellow tanks had the highest L* values, while fish reared in dark grey tanks had the lowest L* values (p < 0.05). This indicates that fish reared in white and yellow tanks were lighter in color compared to those reared in dark grey tanks. Conversely, for the a* index, the highest values were found in fish reared in dark grey and red tanks, while the lowest values were recorded in fish reared in yellow tanks (p < 0.05). Fish reared in red and yellow tanks tended to have the highest b* values, while the lowest values were found in fish reared in white tanks (p < 0.05). This suggests that red and yellow tank colors enhanced the yellow coloration in the skin of juvenile giant trevally.
These results demonstrate that the color of the rearing tank significantly influences the coloration of juvenile giant trevally. Fish reared in lighter colored tanks tended to have lighter skin colors, while fish reared in darker colored tanks tended to have darker skin colors. Additionally, dark tanks enhanced the redness of the fish, yellow tanks made the fish more yellow, while red tanks provided an optimal combination of both hues.
The total accumulated carotenoid content in the skin of juvenile giant trevally was influenced by tank color (Table 4). Fish reared in red and yellow tanks had the highest total accumulated carotenoid content, followed by fish reared in blue and white tanks, and the lowest in fish reared in dark grey tanks (p < 0.05). The total accumulated carotenoid content in the skin of fish in red and yellow tanks was estimated to be 95.9% and 94.6% higher, respectively, compared to dark grey tanks, suggesting that these two tank colors can support the enhancement of pigment accumulation in the skin of juvenile giant trevally.
Parameters | Treatments | ||||
---|---|---|---|---|---|
White | Blue | Red | Yellow | Dark grey | |
Skin (µg/g) | 6.11 ± 0.17b | 6.44 ± 0.14bc | 7.19 ± 0.29c | 7.14 ± 0.34c | 3.67 ± 0.20a |
The effects of tank color on the proximate biochemical composition of juvenile giant trevally are shown in Table 5. Fish reared in white tanks had a higher body moisture content than fish reared in red, dark grey, and yellow tanks, at 71.3% compared to 70.1%–70.3%, respectively (p < 0.05). This trend was opposite to that of crude protein content in the fish body, with the highest content found in fish samples from yellow and red tanks and the lowest in white tanks, at 19.0% and 19.1% compared to 18.2%, respectively (p < 0.05).
Discussion
The results of this study demonstrate that tank color significantly influences growth performance, survival, and feed utilization efficiency of giant trevally. Fish reared in yellow tanks consistently performed the best across all three metrics, suggesting that this color is optimal for the juvenile rearing of giant trevally. Positive results were also recorded for growth parameters of fish reared in red tanks, and for survival and feed utilization efficiency of fish reared in dark grey tanks (Table 1). Conversely, fish reared in blue and particularly white tanks showed the poorest overall performance. Varying tank colors may have impacted the behavior, physiological state, feeding behavior, and feed utilization efficiency of the fish. Indeed, our results showed that giant trevally reared in yellow tanks exhibited better feed utilization efficiency parameters (DFR, FCR, and FE) than those in other tank colors (Table 2). The higher feed intake may have fueled better conversion of feed into fish body mass, leading to increased growth rates. These findings align with previous reports showing that juvenile red sea bream (Epinephelus akaara), snubnose pompano (Trachinotus blochii), thinlip mullet (Liza ramada), and milkfish (C. chanos) (Bera et al., 2019; El‐Sayed & El‐Ghobashy, 2011; Mapunda et al., 2021; Setiadi, 2006) reared in yellow tanks, or Asian sea bass (Lates calcarifer) and oscar (A. ocellatus) (Dopeikar et al., 2024; Ullmann et al., 2011) reared in red tanks, had higher growth, feed utilization efficiency, and survival than fish in other tank colors. In these studies, suitable tank colors were suggested to improve the contrast between the feed and the tank background, thereby enhancing feed visibility, increasing feed consumption, and ultimately improving growth performance (Dopeikar et al., 2024; Setiadi, 2006). Furthermore, lower stress levels, as indicated by reduced cortisol levels, have been observed in oscars reared in red and yellow tanks, which may allow the fish to allocate more energy towards metabolic processes and growth (Dopeikar et al., 2024).
Under similar rearing conditions, visual adaptation varies among fish species, with some preferring low-light environments while others are more active in brighter light conditions (McLean, 2021; Ruchin, 2020). Our findings suggest that giant trevally thrive in yellow, red, and dark grey tanks, with yellow being particularly favorable for growth and feed utilization efficiency. In contrast, the poorer performance in white tanks could be related to overstimulation, affecting feed utilization efficiency and ultimately reducing growth in giant trevally. This has also been reported in other marine fish species, with reduced feed utilization efficiency in orange-spotted grouper (Epinephelus coioides), growth rate in Asian sea bass (L. calcarifer), and both parameters in rainbow trout (Oncorhynchus mykiss) observed when reared in white tanks (Ghavidel et al., 2020; Rahnama et al., 2015; Santisathitkul et al., 2020). Rearing in brighter environments may induce stress, affecting feeding behavior, and the efficiency of nutrient digestion and absorption in fish (Ruchin, 2020). Observations in Beluga sturgeon (Huso huso) and oscars reared in white and blue tanks also recorded higher stress levels (elevated plasma cortisol and glucose) compared to other tank colors, leading to increased energy expenditure for adaptation and ultimately significantly reduced growth parameters (Banan et al., 2013; Dopeikar et al., 2024).
Some studies, however, have reported contrasting results to our findings. Notably, in a synthesis of dozens of studies investigating the effects of tank color on juvenile rearing outcomes across multiple fish families, McLean (2021) found that growth parameters and feed utilization efficiency were superior when fish were reared in white and blue tanks compared to other tank colors (McLean, 2021). These studies suggest that blue tanks resemble the natural habitat color of the fish species, while white tanks allow for better detection of feed, thus positively influencing growth (McLean, 2021). Nevertheless, other studies have reported no significant effects of tank color on growth parameters in summer flounder (Paralichthys dentatus) (McLean et al., 2008), seahorses (Hippocampus abdominalis) (Martinez-Cardenas & John Purser, 2007), orange-spotted grouper (E. coioides) (Ghavidel et al., 2020), or scaled carp (Cyprinus carpio) (Papoutsoglou et al., 2000). These observations further support the notion that the impact of tank color on fish varies depending on the species (Eslamloo et al., 2015; McLean, 2021), developmental stage (Morshedi et al., 2022), and that identifying the optimal color for a given species, or even life stage, in conjunction with other conditions, is crucial for optimizing rearing performance.
Tank color significantly influenced the skin coloration and accumulated carotenoid content of giant trevally. Fish reared in white and yellow tanks exhibited lighter skin colors, while fish reared in dark grey tanks displayed darker skin colors. Notably, red and yellow tanks enhanced the yellow coloration on the fish’s skin, resulting in more attractive coloration compared to other tank colors. Coloration changes in fish are complex processes in response to environmental changes, influenced by multiple factors, including neural, endocrine, signaling pathways, and molecular genetics (Luo et al., 2021; McLean, 2021). Color changes in fish can occur through two mechanisms, morphological or physiological, in response to environmental cues, nutrition, physiological state, or developmental stage (Cal et al., 2017). While the former is determined by increases or decreases in the number of pigment cells in the skin, the latter involves the translocation and rearrangement of pigment cells within the epidermis (Luo et al., 2021). However, both are influenced by the interplay between two main factors, environment and genetics (Luo et al., 2021; McLean, 2021). Most bony fish species can rapidly change their skin color or shade by translocating pigment granules, primarily melanosomes, within dermal pigment cells in response to changes in light and background color (Cal et al., 2017). These mechanisms could explain the skin color changes observed in giant trevally in the current study: lighter skin colors in lighter tanks (white, yellow), darker colors in dark grey, and yellow hues in red and yellow tanks (Fig. 2). This is also consistent with the general trend summarized from dozens of similar studies by Mclean (McLean, 2021). Accordingly, fish reared in white and yellow tanks had higher skin lightness, while black, brown, and grey tanks darkened the fish’s skin. The remaining tank colors, such as red and blue, enhanced the red, orange, and yellow hues of the fish (McLean, 2021; Ninwichian et al., 2018).
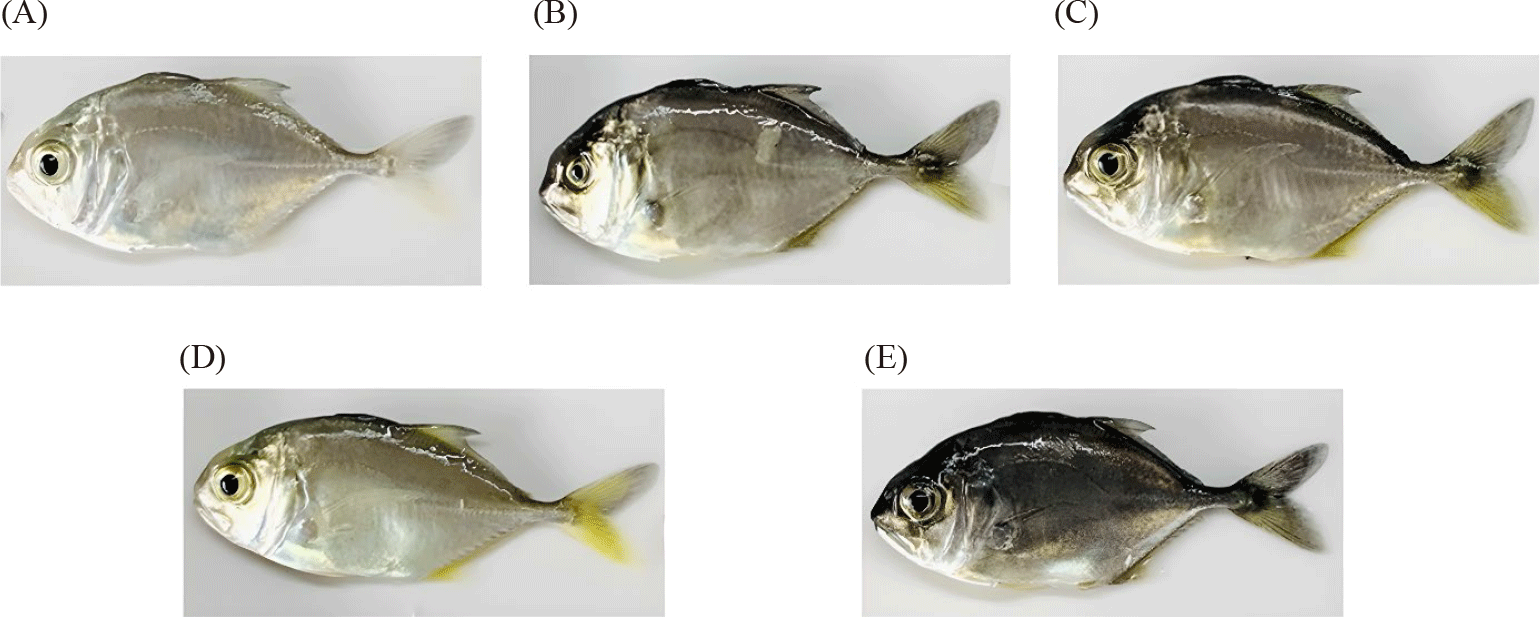
In species with prominent coloration, such as goldfish (C. auratus) or clownfish (Amphiprion ocellaris), the response to tank color changes is even more evident, with their skin becoming lighter and brighter in white tanks, darker in black tanks, instead of the expected yellow-orange-red hues, which reduces their commercial value and marketability (Díaz-Jiménez et al., 2021; Eslamloo et al., 2015). These color changes are linked to neural and endocrine mechanisms that act on pigment cells and pigment granules (Luo et al., 2021). Color changes in fish in response to environmental cues are an adaptive process, allowing them to camouflage for hunting or predator avoidance, thereby increasing their survival and fitness, and are the result of evolutionary processes (Luo et al., 2021; McLean, 2021). In summary, these findings suggest that farmers can use tank color to manipulate the coloration of giant trevally, which could be beneficial for enhancing the aesthetics and marketability of this fish species.
Similar to skin color expression, giant trevally reared in red and yellow tanks showed higher accumulated carotenoid content in their skin compared to other tank colors. Enhanced carotenoid accumulation in specific tank colors has also been reported in other species, such as clownfish (A. ocellaris) in blue, clear, and white tanks or leopard coral grouper (Plectropomus leopardus) in red and white tanks (Song et al., 2022; Tran et al., 2023). Using gene expression analysis of scarb1, a gene that regulates carotenoid uptake and metabolism in fish, Song et al. (2022) found that leopard coral grouper reared in red and white tanks had the highest gene expression levels, followed by blue and clear tanks, and the lowest in black tanks. Correspondingly, the carotenoid content in the fish’s body was also higher in the red and white tanks, while melanin was dominant in the clear and black tanks (Song et al., 2022). From a neuroendocrine perspective, tank color and light are thought to modulate fish skin color through neuropeptide hormones and photoreceptor cells (Shin & Choi, 2014). Background color influences scarb1 gene expression through the visual center, which subsequently affects carotenoid uptake and transport, ultimately altering skin color and accumulated carotenoid content in the fish (Song et al., 2022). Given the health benefits of carotenoids in fish, these findings suggest that red and yellow colors could support the overall health of giant trevally, further strengthening the conclusion that red and yellow, particularly yellow, are beneficial for the culture of this fish species.
The results of the study also revealed that tank color influenced the biochemical composition of giant trevally. While lipid and ash contents were not significantly different, crude protein content was found to be higher in samples from fish reared in yellow tanks, while moisture content was higher in samples from fish reared in white tanks. These differences could be related to feed intake and feed utilization efficiency, thereby affecting the efficiency of metabolic processes and nutrient accumulation. Indeed, feed utilization efficiency indices were better for giant trevally reared in yellow and red tanks. This could contribute to the higher protein content observed, as protein is a major component of muscle structures and body mass in fish (Fafioye et al., 2005; Ruchin, 2020), in line with the higher growth rates observed in fish from yellow and red tanks. On yellowfin seabream, Mapunda et al. (2021) also reported the effect of tank color on the biochemical composition of fish, with red resulting in higher crude protein and moisture contents while yellow led to higher crude lipid content than other tanks (Mapunda et al., 2021). Conversely, negative effects on both lipid and ash contents were reported in Asian sea bass (L. calcarifer) reared in red tanks, while other components were not significantly different (Santisathitkul et al., 2020). In scaled carp (C. carpio), blue tank was reported to promote growth, feed utilization efficiency, and higher body protein content compared to black tanks (Papoutsoglou et al., 2000). This contrasts with observations in African catfish (Clarias gariepinus), where body protein, lipid, and moisture contents were higher, corresponding to growth indices, in fish reared in black tanks compared to other tanks (Okomoda et al., 2017).
Higher feed consumption, along with higher protein and energy content, has been suggested to enhance body mass in fish (Manjappa et al., 2011). In addition to efficient feed utilization, energy conserved from a less stressful, favorable environment when reared in suitable tank colors could contribute significantly to growth and nutrient accumulation in fish (Manjappa et al., 2011; Okomoda et al., 2017). Overall, studies on the effects of tank color on the biochemical composition of fish are still limited, as stress-related parameters, immunity, and other aspects of overall health have received more attention (McLean, 2021). Furthermore, with the results obtained, there is no strong consensus on the effects of tank color on the biochemical composition of fish (Okomoda et al., 2017). In contrast to the reports above, no significant effects of tank color on the biochemical composition of scaled carp (C. carpio) (Papoutsoglou et al., 2000), thinlip mullet (L. ramada) (El‐Sayed & El‐Ghobashy, 2011), or orange-spotted grouper (E. coioides) (Ghavidel et al., 2020) were observed. The analyses above suggest that the extent of influence of tank color on the biochemical composition of fish remains variable and requires further investigation.
The data obtained in our study provide valuable preliminary information on the effects of tank color on growth parameters, survival rate, feed utilization efficiency, coloration, and biochemical composition of giant trevally, identifying yellow as the most suitable color for juvenile giant trevally rearing. These contributions are important in efforts to optimize rearing efficiency for this species, leading to improved technological processes and practical applications in production. However, our study has some limitations. Firstly, the effects of tank color on other physiological and behavioral parameters, such as stress levels, activities of metabolic enzymes, and immune function, were not addressed. Secondly, the biochemical and endocrine pathways responsible for the observed changes in growth, survival rate, feed utilization efficiency, skin coloration, and biochemical composition in fish exposed to different tank colors are not yet fully understood. Additionally, the neural, endocrine, and molecular genetic mechanisms governing the formation, and maintenance of coloration in this species under the influence of tank color, require further investigation.
Conclusion
This study demonstrates that tank color significantly influences growth parameters, survival rate, feed utilization efficiency, skin coloration, accumulated carotenoid content, and biochemical composition of giant trevally. These findings have important implications for giant trevally farmers, as they can help optimize rearing conditions to improve fish health, productivity, and quality. Future research should focus on the physiological mechanisms behind these effects and investigate the influence of tank color on other aspects of physiology and behavior in this economically valuable fish species.