Introduction
Larval mortality caused by problems with nutrition, metamorphosis, moulting, and larval growth is a significant problem in crab aquaculture, particularly in the cultivation of mud crabs and swimming crabs (Chong, 2022; Pérez-Pérez et al., 2023). Crab hatcheries frequently utilize live feed, such as rotifers and Artemia, to provide the nourishment for early stage of crab. These live feeds are commonly supplemented with microalgae species to enhance the nourishment provided to the crab larvae. However, the survival rates (SRs) associated with this feeding method can be suboptimal or unpredictable (Chong, 2022).
In crustaceans, lipids serve as a crucial energy source and provide essential fatty acids (EFA), phospholipids, and vitamins soluble in fats (Ouraji et al., 2010; Sheen & Wu, 1999; Wei et al., 2018). One key component of crab diets is highly unsaturated fatty acids (HUFA; Suprayudi et al., 2002), derived primarily from fish oil (Kabeya et al., 2018), which are polyunsaturated fatty acids (PUFAs) with 20 or more carbon atoms and at least two double bonds (Wei et al., 2018). Despite its nutritional value, fish oil is expensive and only sometimes readily available, prompting the search for more cost-effective alternatives. Research has shown that the FA profile in the hepatopancreas and muscle of mitten crabs reflects their dietary FA intake (Wang et al., 2021). Fish oil is a rich source of HUFA. In contrast, plant oils mainly contain α-linolenic acid (ALA) and linolenic acid (LA, 18:2n-6), precursors to HUFAs like eicosapentaenoic acid (EPA) and docosahexaenoic acid (DHA; Turkmen et al., 2017). Live feeds such as rotifers and Artemia nauplii are commonly used to feed early-stage marine finfish and shrimp. However, they often lack sufficient levels of FAs, particularly EPA (20:5n-3) and DHA (22:6n-3), which are vital for proper growth and development in marine larvae (Khairy & El-Sayed, 2012; Wang et al., 2021). These FAs are necessary nutrients for ensuring the correct growth and development of animals (Ramos-Llorens et al., 2023). The most essential n-3 long-chain polyunsaturated fatty acids (LC-PUFAs) for crustacean growth, moulting, and development are DHA and EPA (Wang et al., 2021). Previously reported that the Juvenile mud crab requires a higher amount of DHA (Paran et al., 2022) compared to EPA. A research study found that the rotifer species has a composition characterized by the presence of 2% EPA and 0.1% DHA. In contrast, Artemia has a composition defined by a content of 5.3% EPA and 0.0% DHA (Bell & Sargent, 2003; Wang et al., 2021).
Enriching live feed with FAs of natural origin is one potential strategy to ensure that live feed satisfies the nutritional requirements of crab larvae. Moringa oleifera leaf powder is a natural component with the potential to enhance the nutritional composition of live feed. FA in M. oleifera has been documented in scientific literature. The most prevalent FA in its leaves was LA (C18:3), with 62.4% of the total FA content. This was followed by LA (C18:2) and palmitic acid (C16:0) (Monteiro et al., 2022). The utilization of Moringa leaves in aquaculture activities holds promise due to their diverse nutritional composition. These leaves contain a comprehensive range of macronutrients, including proteins, carbohydrates, and fats, as well as a variety of micronutrients such as vitamins A, B1, B2, B3, C, E, and K, and minerals such as calcium, copper, iron, magnesium, phosphorus, potassium, and zinc (Aja et al., 2021; Monteiro et al., 2022).
The importance of utilizing appropriate feed during the larval rearing phase is unquestionable. It is crucial to enhance the nutritional content of rotifers and Artemia by increasing the quantities of EFAs through bioencapsulation, specifically by adding Moringa leaf powder. The issue of optimism revolves around the potential improvement in live feed quality by utilizing the bioencapsulation technique to incorporate Moringa leaf powder into rotifers and Artemia. The importance of utilizing appropriate feed during the larval rearing phase is unquestionable. It is crucial to enhance the nutritional content of rotifers and Artemia by increasing the quantities of EFAs through bioencapsulation, specifically by adding Moringa leaf powder. The issue of optimism revolves around the potential improvement in live feed quality by utilizing the bioencapsulation technique to incorporate Moringa leaf powder into rotifers and Artemia. The objective of this study was to evaluate the effect of enrichment of live food with M. oleifera leaf on the FA composition, especially EPA and DHA, in Rotifer and Artemia, as well as its impact on the SR and larval stage index of mud crabs. This study also aims to explore the potential benefits of using moringa leaf meal as a natural food enrichment for aquatic organisms, focusing on improving nutritional quality and supporting the early growth of mud crab larvae.
Materials and Methods
Fresh Moringa leaves with a dark green color that is taken from the first petiole branch (under the stem) to the seventh petiole and is not yet yellow are the first steps in making Moringa leaf powder. Additionally, it is carried out to separate the fresh leaves from the yellow leaves. The gathered leaves are then thoroughly cleaned. Afterwards, enter the blanching step for 1–2 minutes at 80°C while utilizing a water bath (Premi & Sharma, 2018). The Moringa leaves were additionally dried in an oven at 60°C for 5 hours until they turned dry, which was shown by the leaves turning brittle and easily broken. Furthermore, flour is made from ground Moringa leaves. Then, 80 mesh-sized sieves separated the flour from the moringa leaves. Until the following step, the gathered Moringa leaf powder is kept in an airtight polyethene bag.
Brachionus sp. cultured in autoclaved seawater (salinity 25 ppt). Before the enrichment experiment, the rotifers were screened and deprived of feed for 12 hours to avoid disturbance of the gut contents with yield. For enrichment, the filtered rotifers were transferred to enrichment media with a stocking density of 500,000 ind./L. The enrichment process was carried out directly using the immersion method on Rotifer using Moringa leaf flour dissolved at 0, 50, 100, and 150 mg/L concentrations.
Artemia was hatched in 25 ppt saline seawater, which had been sterilized using an autoclave. Artemia was cultured for 24 hours until they hatched into nauplii and were ready for enrichment. Enrichment was done using moringa leaf flour with 0, 50, 100, and 150 mg/L concentrations by soaking. Each immersion treatment was repeated three times to obtain an accurate value. Soaking was carried out for 6 hours at a density of 300,000 ind./L.
FA concentrations in Moringa leaf powder, Rotifer, Artemia and mud crab larvae were carried out using gas chromatography-flame ionization detection based on the American Oil Chemists’ Society (AOCS) Ce 2-66 method with slightly modification (AOAC, 2000; Nimal Ratnayake et al., 2006). Instrument and condition of GC was presented in Table 1.
Mud crab larvae were sourced from the Brackish Water Aquaculture Centers in Takalar Regency, South Sulawesi, Indonesia. The live feeds utilized were rotifers and Artemia nauplius, which were either not enriched or enriched with varying concentrations of moringa leaf powder at 50, 100, and 150 mg/L. At the onset of the feeding experiment, zoea stage 1 larvae were placed in a 450 L seawater tank with a salinity of approximately 25 ppt in a fibre tank at a density of 50 individuals per liter. Each feeding regimen was replicated three times. The developmental stages of the mud crab larvae were identified according to the criteria established by Paran et al. (2022), as shown in Fig. 1. The feeding protocol is outlined in Fig. 2. Feeding the mud crab larvae with rotifers began at the zoea 1 stage and continued until the megalopa stage, occurring twice daily at a density of 30 individuals per milliliter. Subsequently, feeding with Artemia nauplius was carried out from the zoea 2 to the megalopa stage twice daily, but at a lower density of 5 individuals per milliliter.
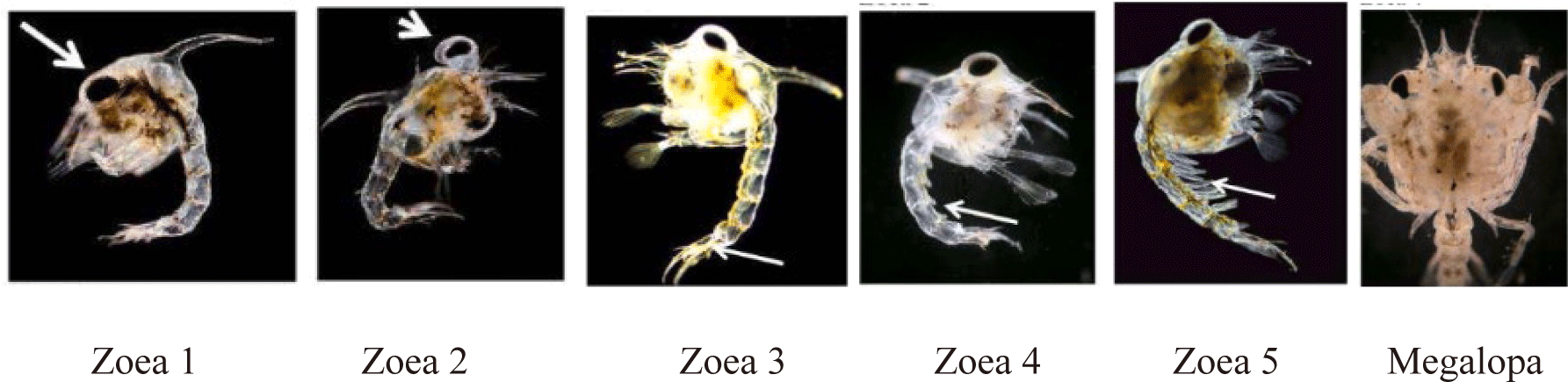
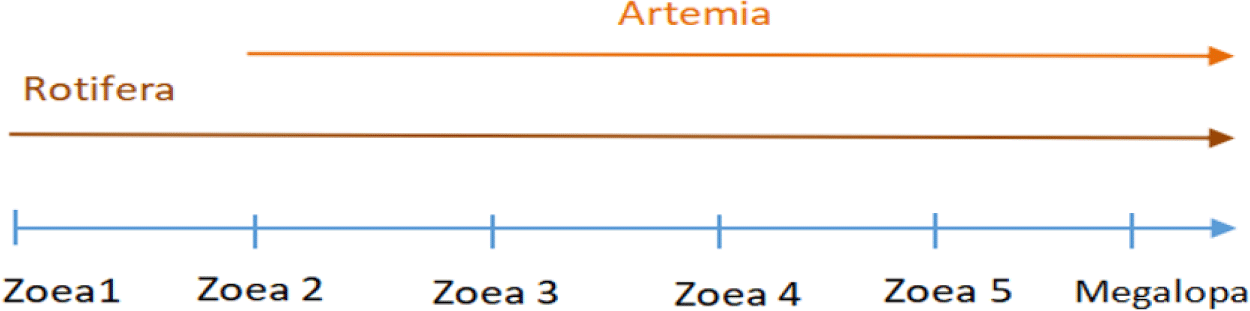
Larvae Stage Index (LSI) for the mud crab larvae was calculated daily throughout the study using the Equation (1):
X1 = amount of previous stage larvae, X2 = previous stage, Y1 = amount of highest stage larvae, Y2 = highest stage, Z = total number of samples. The stages are assigned scores as follows: Zoea 1 = 1, Zoea 2 = 2, Zoea 3 = 3, Zoea 4 = 4, Zoea 5 = 5, and Megalopa = M (Paran et al., 2022; Syafaat et al., 2019).
The calculation of the SR for mud crab larvae was performed using the following Equation (2) (Chen et al., 2023):
Results
The measurement of FA composition in Moringa leaf powder was conducted after flouring. The analysis indicated the presence of linoleic acid as a prominent constituent, comprising approximately 2.85% of the total composition (Table 2). Cromatogram identification of FA compound from Moringa leaf powder indicated that C18n3 was the highest compound (Fig. 3).
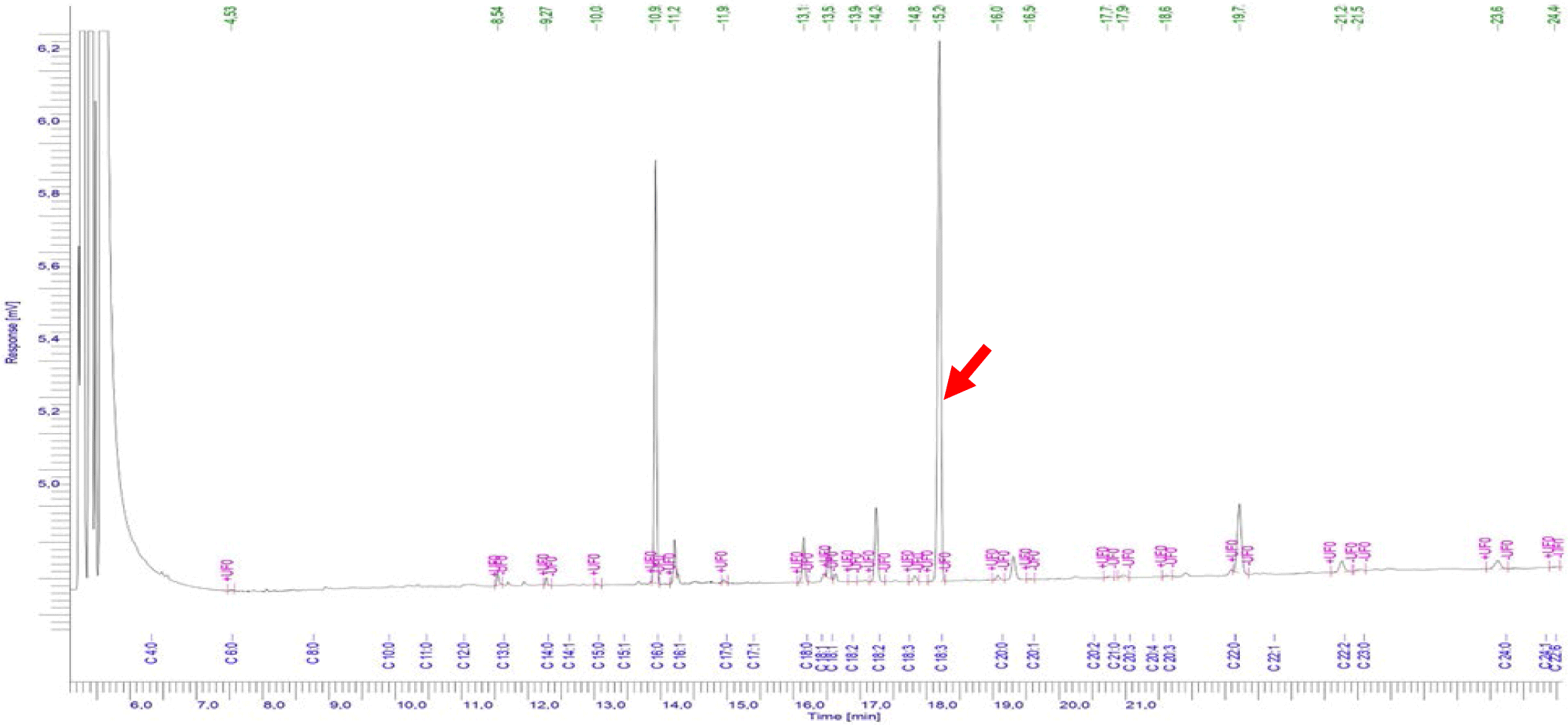
EPA concentrations increased in Rotifer and Artemia along with additional doses in M. oleifera powder at either 50, 100, or 150 mg/L (Table 3). The ANOVA outcomes revealed that there were statistically significant variations in the treatment groups of Rotifer and Artemia (p < 0.05). The levels of EPA in the groups treated with Moringa flour enrichment at concentrations of 50, 100, and 150 mg/L were significantly different from those in the control group without enrichment (0 mg/L) for both Rotifer and Artemia.
Treatment (mg/L) | EPA content (mg/g) in Rotifer | EPA content (mg/g) in Artemia |
---|---|---|
0 | 0.71 ± 0.02c | 0.03 ± 0.01d |
50 | 0.81 ± 0.01b | 0.05 ± 0.01c |
100 | 0.88 ± 0.01a | 0.07 ± 0.01b |
150 | 0.93 ± 0.04a | 0.09 ± 0.01a |
Table 4 shows data on DHA in Rotifer and Artemia enriched with Moringa oliefera powder. DHA content in both Rotifer and Artemia increased as the concentration of M. oliefera powder increased. The highest value of DHA in Rotifer is enriched with 150 mg/L moringa leaf powder, which resulted in 6.41 mg/g of DHA. The treatment with the highest value of DHA in Artemia was also the one with 150 mg/L of moringa leaf powder, which resulted in 0.07 mg/g of DHA. These values are significantly higher than other treatments.
Treatment (mg/L) | DHA content (mg/g) in Rotifer | DHA content (mg/g) in Artemia |
---|---|---|
0 | 2.72 ± 0.01d | 0.01 ± 0.01c |
50 | 4.75 ± 0.02c | 0.03 ± 0.01bc |
100 | 5.62 ± 0.04b | 0.05 ± 0.01ab |
150 | 6.41 ± 0.09a | 0.07 ± 0.01a |
The protein content in mud crab larvae increased along with increasing enrichment in rotifers and Artemia up to a dose of 100 mg/L. A decrease in crude protein content was found in the 150 mg/L treatment. The same thing was also found in the results of proximate analysis of crude lipid and energy content in mud crab larvae, and they were significantly different across treatments (p < 0.05) (Table 4). The mud crab larvae group had the highest protein and fat content levels when subjected to 100 mg/L. The depletion of lipids and proteins in mud crab larvae exhibited a positive correlation with the concentration of EPA/DHA in Artemia and Rotifer, as indicated by the data in Table 3 and Table 4. This correlation was observed up to the treatment level of 100 mg/L.
The observed LSI values of crab larvae at different stages up to 21 days post-hatching indicated a general trend of increasing values across the various treatments (Fig. 4). The feeding treatment without the enrichment of Moringa flour yielded the lowest LSI value (0 mg/L). Using a enriched live feed with 100 mg/L Moringa flour resulted in significant values of LSI for mud crab larvae over most of the experiment period.
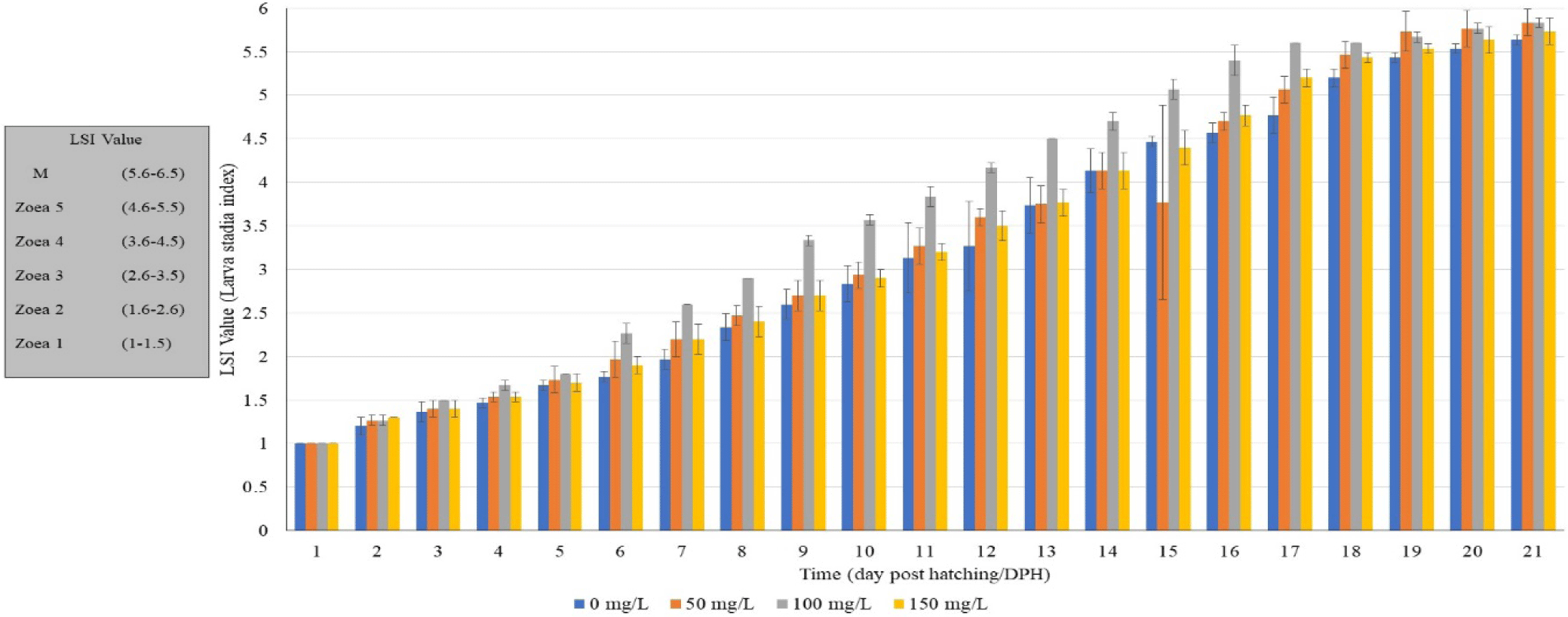
Statistically significant variations (p < 0.05) were observed in the transformation of mud crab larvae from the Zoea 1 (Z1) stage to the Megalopa (M) stage throughout the 21-day cultivation period. The mud crab larvae subjected to the treatment with a concentration of 100 mg/L exhibited the most rapid transition to stage M (Megalopa), specifically occurring on the 16th day post hatching (Fig. 5). Changes from Zoea 1 to Zoea 4 require about three days in each developmental stage. Zoea 5 to Megalopa was in 3 to 4 days. The treatment with a concentration of 0 mg/L, also known as the control treatment, exhibited the most prolonged duration for mud crab larvae to undergo metamorphosis into megalopa.
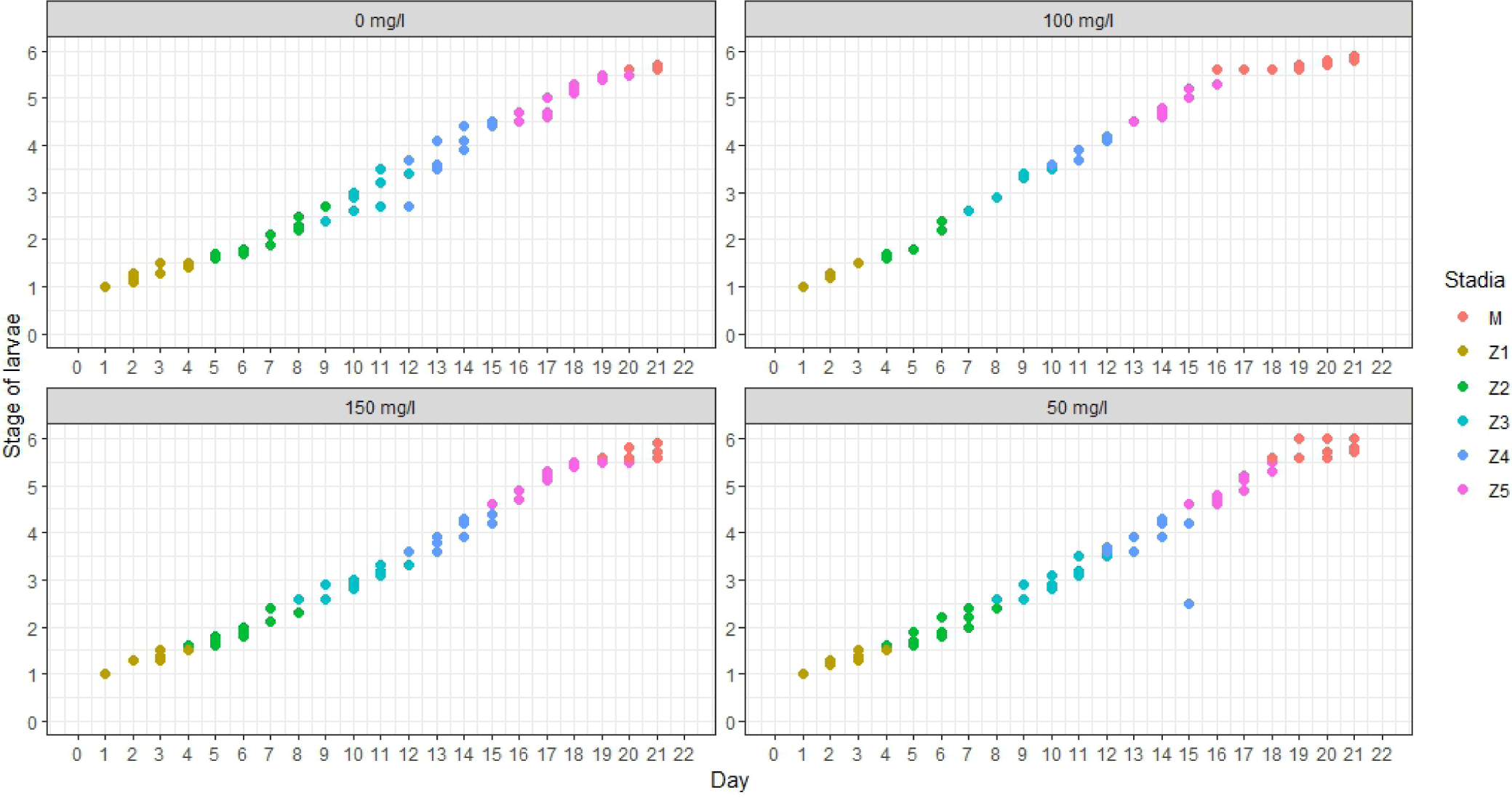
The SR positively correlated with the amount of M. oleifera in the natural feed provided to the rotifers and Artemia. However, a drop in the SR was observed in the treatment with a 150 mg/L concentration. The experimental results indicate that the natural feed enrichment treatment utilizing 100 mg/L M. oleifera flour yielded the best SR of crab larvae, reaching 39.82% (Fig. 6).
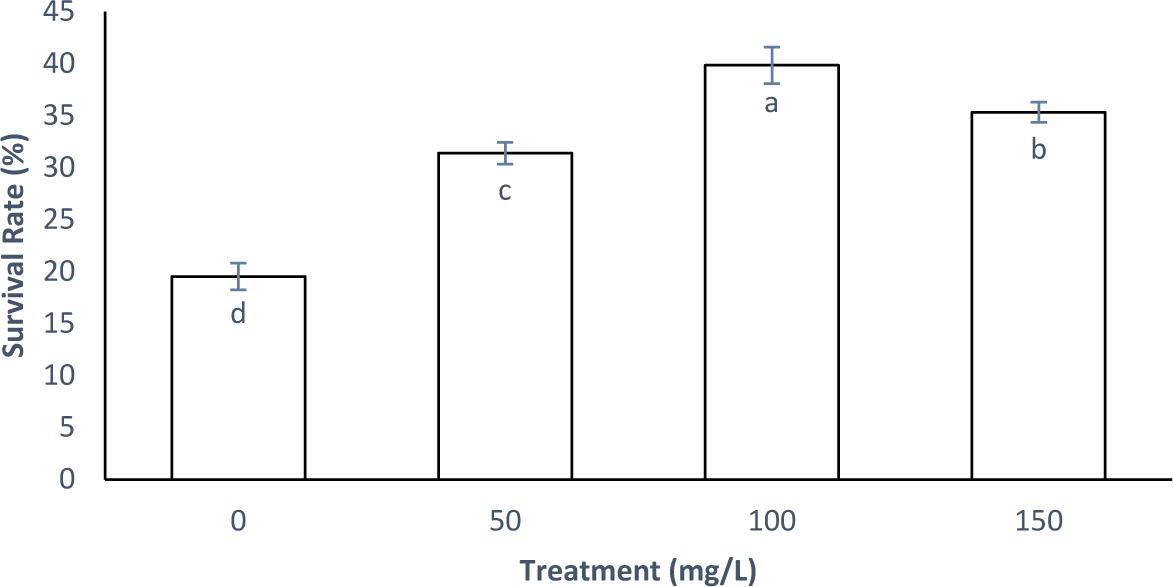
Discussion
The soft woody plant, M. oleifera, which grows quickly, is found mainly in tropical and subtropical regions (Su & Chen, 2020). Because of M. oleifera’s extensive nutritional, antioxidant, and therapeutic content, researchers studying animal husbandry have studied it more and more in recent years. The leaf of M. oleifera is rich in crude protein, vitamins, minerals, and FAs. M. oleifera contains a protein concentration ninefold greater than yogurt, a calcium concentration seventeenfold greater than milk, a vitamin C concentration sevenfold greater than oranges, a vitamin A concentration tenfold greater than carrots, an iron concentration twenty-fivefold greater than spinach, and a potassium concentration fifteenfold greater than bananas (Gopalakrishnan et al., 2016; Su & Chen, 2020). The analysis of FA content in Moringa leaf flour indicates a substantial presence of LA (C18:3n3), which is 2.85% W/W. The FA components found in M. oleifera powder present a favorable prospect for Rotifers and Artemia to produce LC-PUFA. According to Pérez et al. (2022), the transformation of LA (C18:3n3) into EPA and DHA is facilitated by a series of metabolic processes, including elongation, desaturation, and β-oxidation (Pérez et al., 2022).
The organisms under examination are rotifers and artemia. During the first growth phase of fish and prawns, a natural feed is utilized to ensure the provision of adequate necessary nutrients that are crucial for supporting the overall health of these aquatic organisms. These nutrients include EPA and DHA. DHA, often known as 22:6n-3, is an omega-3 FA consisting of 22 carbon atoms and six double bonds (Carlson & Colombo, 2016). DHA can be obtained either by ingesting dietary sources rich in this FA or by the body’s own production from ALA. The transformation of ALA into DHA involves a sequence of enzyme-driven steps, including desaturation and chain elongation (Domenichiello et al., 2015).
Essential nutrients like LC-PUFA, including EPA (20:5n-3) and DHA (22:6n-3), are vital for supporting robust growth and ensuring the survival of various organisms, encompassing both humans and different animal species (Castro et al., 2016; Ramos-Llorens et al., 2023; Swanson et al., 2012). DHA is type of FA that have a long chain of carbon atoms, with a carboxyl group (-COOH) at one end and a methyl group (–CH3) at the other. The carbon atoms in the chain ca be connected by single or double bonds, which affect the shape and properties on the FA.
According to this study, it has been proven that the concentration of DHA in rotifer is significantly higher than that found in Artemia. The observed effect can be attributed to the ability of Rotifers to synthesize DHA internally. The utilization of linolenic acid as a starting material to produce DHA in rotifers has been observed when M. oleifera leaf powder is included in their diet, as shown in Table 1. This raw material was administered to the rotifers by an enrichment procedure. Rotifers are filter organisms that exhibit passive and non-selective feeding behavior, making them amenable to alteration of their nutritional composition via enrichment techniques (Domenichiello et al., 2015).
Rotifers are microscopic aquatic animals that have the remarkable ability to biosynthesize DHA, LC-PUFA that is essential for many biological functions. DHA is usually obtained from dietary sources, such as fish oil, but rotifers can produce it from other FAs by using enzymes called elongases and desaturases. The synthesis of DHA in rotifers involves two main steps: elongation and desaturation. Elongation is the process of adding two carbon atoms to the end of a FA chain, while desaturation is the process of introducing a double bond between two carbon atoms. Rotifers have different types of elongations and desaturases that can modify the length and the degree of unsaturation of FAs. For example, rotifers can convert ALA, an 18-carbon FA with three double bonds, into DHA, a 22-carbon FA with six double bonds, by following this pathway:
where:
SDA = stearidonic acid
DPA = docosapentaenoic acid
ETA = eicosatetraenoic acid
EPA = eicosapentaenoic acid
des = desaturation
e = elongation
This pathway has been confirmed by several studies that measured the FA composition of rotifers fed with different diets or incubated with different precursors (Abbas et al., 2023; Kabeya et al., 2018, 2020; Lubzens et al., 1985; Matsunari et al., 2012; Paran et al., 2022; Pérez et al., 2022; Reis et al., 2021).
Artemia, on the other hand, have fewer enzymes that can modify FA. They mainly rely on the FA that they get from their food, and they store them in a form of natural lipids which mainly used for energy. Artemia have less EPA and DHA than rotifer because they use DHA when they were growing and molting (Roo et al., 2023). Ramos-Llorens et al. (2023) have indicated that Artemia franciscana lacks the capability to produce LC-PUFA because it does not possess the necessary desaturase enzymes genetically. They have also shown that it is crucial to supplement with external EFAs to prevent signs of deficiency (Ramos-Llorens et al., 2023).
Based on the Table 5, the results indicated that adding Moringa flour to the live feed for mud crab larvae increase their crude protein, crude lipid, and energy content, but decrease their ash content. The highest value for crude protein, crude lipid, and energy were obtained in group that their live feed received 100 mg/L Moringa flour, while the lowest values were obtained in group that none enrichment live feed with Moringa flour (0 mg/L). The highest value for ash was obtained in the group that their live feed received no Moringa flour, while the lowest value was obtained in group that their live feed received 100 mg/L of Moringa flour. The results suggest that enrichment live feed with moringa of mud crab larvae can improve their nutritional quality and energy efficiency, but may also reduce their mineral content. The optimal dose to feed of mud crab larvae around 100 mg/L, as it provides the best balance protein, lipid, energy, and ash. Additionally, it was observed that including 150 mg/L of M. oleifera flour in the life feed reduced the crude protein content. This phenomenon demonstrates the existence of an optimal threshold for the EPA and DHA levels in the live feed supplied. If this threshold is surpassed, it will reduce the crude protein content in crab larvae. It has been reported that there is a negative correlation between the crude protein content in mud crab hepatopancreas and the EPA/DHA ratio of the diet fed (Wang et al., 2021). Wang et al. (2021)’s study has demonstrated that elevating the EPA/DHA ratio in the crabs’ diet correlates with a lowered expression of the cptI gene (carnitine palmitoyltransferase I). This lowered expression suggests a diminished movement of long-chain FAs into the mitochondrial matrix, which leads to a decrease in β-oxidation and an increase in the buildup of lipids (Wang et al., 2021).
In the current research, the improvement of FA including EPA and DHA in the diet of live feed had a beneficial impact on the growth of mud crab larvae. As the moringa enrichment concentration in live feed increased, the DHA content decreased according to Table 6. The highest growth rate was observed in the group with 100 mg/L enrichment, which suggested that DHA supported their faster growth. The transition from the phase of zoea 1 to the megalopa occurred most rapidly in larvae that consumed natural food supplemented with 100 mg/L of Moringa flour, achieving this developmental milestone within 16 days after hatching. This is a faster rate compared to a previous study that recorded a metamorphosis duration of 18 days for Scylla serrata larvae to progress from zoea 1 to megalopa (Paran et al., 2022). These findings are similar with Jinbo et al. (2013), which their finding that growth and morphogenesis of horsehair crab (Erimacrus isenbeckii) faster if Artemia have more EPA by enrichment. N-3 Highly Unsaturated Fatty Acids (N3-HUFAs), especially EPA and DHA, are critical for the development of S. serrata larvae when provided in specific amounts (Suprayudi et al., 2002).
The lack of adequate EPA and DHA in live feeds such as Rotifer and Artemia has been shown to affect the vitality of the larvae (Gunarto & Herlinah, 2015). Moreover, the lipid profiles in Rotifer and Artemia nauplii may influence the growth and SRs of marine crustacean larvae, including those of mud crabs (Holme et al., 2007). This study suggests the use of M. oleifera flour as an enrichment for live feeds such as rotifers and Artemia, as it appears to promote the early developmental stages of mud crab larvae. This research revealed that the live feed enrichment treatment with 100 mg/L of moringa leaf powder had the highest SR. However, the SR went down when the amount of moringa increased to 150 mg/L. This indicates that the best value for enhancing the SR is around 100 mg/L. FAs are essential nutrients for the development and growth of crustaceans, and different FAs may have different effects on their physiological and biochemical processes. According to Suprayudi et al. (2004), the survival of mud crab (Scylla serrata) larvae was strongly affected by EFA concentrations between Artemia and enriched rotifer diets, where insufficient EFA was observed in larvae fed unenriched Artemia. Another finding showed similar result, that the survival of red deep-sea crab (Chaceon quinquedens) larvae was affected by the diet and temperature, and the larvae fed with rotifers and Artemia had higher SRs than those fed with algae or unfed (Pérez-Pérez et al., 2023). This finding is a new opportunity for future research development to support the sustainability of aquaculture by using organic materials to help the fitness of mud crabs in their early developmental stage. Further research on developing mud crab larvae in the next phase is quite an exciting challenge.