Introduction
Lipid is one of the most important nutrients in aquafeeds as a source of energy and essential fat-soluble substances (Meng et al., 2019). Supplementation of optimum levels of lipid into the feeds can promotes the animal growth through sparing protein (Weihe et al., 2019). Thus, the lipid levels in feed is closely associated with fish growth and feed utilization (Lin & Yeh, 2022; Zheng et al., 2010). For juvenile (43.05 g) Japanese seabass (Lateolabrax japonicus), the optimum dietary lipid level was suggested to be 3.9%–7.3% based on fish growth (Xie et al., 2021). Wang et al. (2021) suggested 104.6–128.3 g/kg as optimal dietary lipid level for maximum growth of spotted knifejaw (Oplegnathus punctatus) in juvenile stage (6.26 g).
However, excessive lipid levels in aquafeeds can cause lipid accumulation and inflammation in the liver and intestine of fish, impairing their immune response and stress resistance (Jin et al., 2019). Previous studies emphasized that the high rancidity of high-lipid feeds can lead to poor fish growth and low carcass quality through oxidative damage of macromolecules such as DNA, proteins and lipids (Guo et al., 2019; Jia et al., 2020). Shen et al. (2022) demonstrated that juvenile (2.08 g) black seabream (Acanthopagrus schlegelii) fed diet with lipid exceeded 188.9 g/kg showed poor growth, hepatic lipid deposition, lower antioxidant enzyme activity and downregulation of antioxidant enzyme gene expression. Therefore, dietary supplementation of adequate levels of lipid is important to maximize growth and metabolic homeostasis of fish in aquaculture (Wang et al., 2019).
Parrot fish (Oplegnathus fasciatus) is one of the most popular fish in South Korean aquaculture. However, studies have not been yet reported for the optimum lipid levels of parrot fish through a long-term feeding trial. In this study, the optimum lipid level for juvenile parrot fish was established by 22 week of a feeding trial, based on growth, feed utilization and lipid accumulation.
Materials and Methods
The main protein sources were fish meal and soybean meal in experimental diets. Five experimental diets to have 80, 120, 160, 200, and 240 g/kg lipids (designated as L80, L120, L160, L200, and L240, respectively) were prepared by graded supplementation of squid liver oil (Table 1). The determined lipid levels in the experimental diets were 79, 119, 160, 193, and 228 g/kg for the L80, L120, L160, L200, and L240, respectively. Squid liver oil and distilled water (80 g/kg) were added to the dry material mixture to prepare the dough. The experimental diets were pelletized to be 3 mm by a pelletizer (SMC-12, Kuposilice, Busan, Korea) and dried (30°C for 10 h). The dried pellets were stored at –20°C.
3) Mineral premix (g/kg): magnesium sulfate heptahydrate, 80; sodium phosphate monobasic dihydrate, 370; potassium chloride, 130; Ferric citrate, 40; zinc sulfate heptahydrate, 20; Ca-lactate, 356; copper chloride, 0.2; aluminium chloride hexahydrate, 0.15; sodium thiosulfate pentahydrate, 0.01; manganese sulfate monohydrate, 2; cobalt chloride hexahydrate, 1.
4) Vitamin premix (g/kg of mixture): L-ascorbic acid monophosphate, 100; α tocopheryl acetate, 20; thiamin hydrochloride, 4; riboflavin, 4.4; pyridoxine hydrochloride, 4; niacin, 30; pantothenic acid hemicalcium salt, 14.5; myo-inositol, 40; biotin, 0.2; folic acid, 0.48; menadione, 0.2; retinyl acetate, 1; cholecalficerol, 0.05; cyanocobalamin, 0.01.
After acclimation, juvenile parrot fish (initial mean body weight: 6.35 ± 0.03 g) was randomly distributed into 15 tanks (25 fish per tank). During 22 week of the feeding trial, the experimental diets were supplied to the parrot fish two times a day (08:30 and 18:30 h). Sand-filtered sea water was flowed (3 L/min) into the tanks. Dissolved oxygen (DO) concentration in the tanks were maintained by constant aeration. DO, salinity and water temperature were daily monitored. The photoperiod schedule was controlled by fluorescent light to be 12L:12D. The water quality was maintained during feeding trial as follows: temperature 17.8°C ± 1.7°C, salinity 33.0 ± 1.2 psu and DO 7.15 ± 0.52 ppm. The experimental protocols complied the guidelines of animal ethical treatment of Jeju national university for the management and use of experimental animals.
After 22 week of the feeding trial, all the fish in tanks were captured to measure body weight and length, individually for the calculation of growth performance and feed utilization. Three fish from each tank were anesthetized with 2-phenoxyethanol (200 ppm; Sigma-aldrich, St. Louis, MO, USA). Fish were dissected and the viscera was collected to measure visceral weight and fat contents, for viscerosomatic index (VSI) and visceral fat (VF). Fish liver was weighed to calculate hepatosomatic index (HSI) and then frozen at –80°C. Other 3 fish were captured for the whole-body proximate composition analysis. The methods described in AOAC (2005) were used for the proximate composition analysis of diets and whole-body.
The experimental tanks were allocated by using a randomized complete block design. Data of each parameter were initially checked for normality. All the data were presented as mean ± SD, and percentage data were transformed to arcsine values before statistical analysis. Statistical analyses of results were done using software of SPSS 24.0 (International Business Machines, Armonk, NY, USA). Duncan’s multiple range test was used to test the significant difference by one-way ANOVA (p < 0.05). The optimum dietary lipid level was determined by broken-line regression model based on the fish specific growth rate (SGR) and lipid retention (LR).
Results
Final body weight (FBW) of the fish was increased as lipid levels in diets increased to 160 g/kg (Table 2). Fish fed the L160, L200, and L240 diets showed significantly higher FBW and SGR than fish fed the L80, and L120 diets (p < 0.05). Feed conversion ratio (FCR) was decreased as lipid levels in diets increased to 160 g/kg. Fish fed the L160, L200, and L240 diets showed significantly lower FCR than fish fed the L80, and L120 diets (p < 0.05). Fish fed the L160, L200, and L240 diets showed significantly higher protein efficiency ratio (PER) than fish fed the L80, and L120 diets (p < 0.05). Lipid efficiency ratio (LER) of the fish were significantly decreased with increasing dietary lipid level (p < 0.05). Fish fed L160, L200, and L240 diets showed significantly lower LR than fish fed the L80, and L120 diets (p < 0.05). Dietary lipid levels from 80 g/kg to 240 g/kg were not significantly affected to fish survival (p > 0.05). Based on broken-line regression with SGR (Fig. 1A) and LR (Fig. 1B) results, the optimum dietary lipid level for maximum growth and lipid utilization in juvenile parrot fish was estimated as 120.06–152.73 g/kg.
a–f The significant difference among treatments was indicated by different superscripts in each column (p < 0.05).
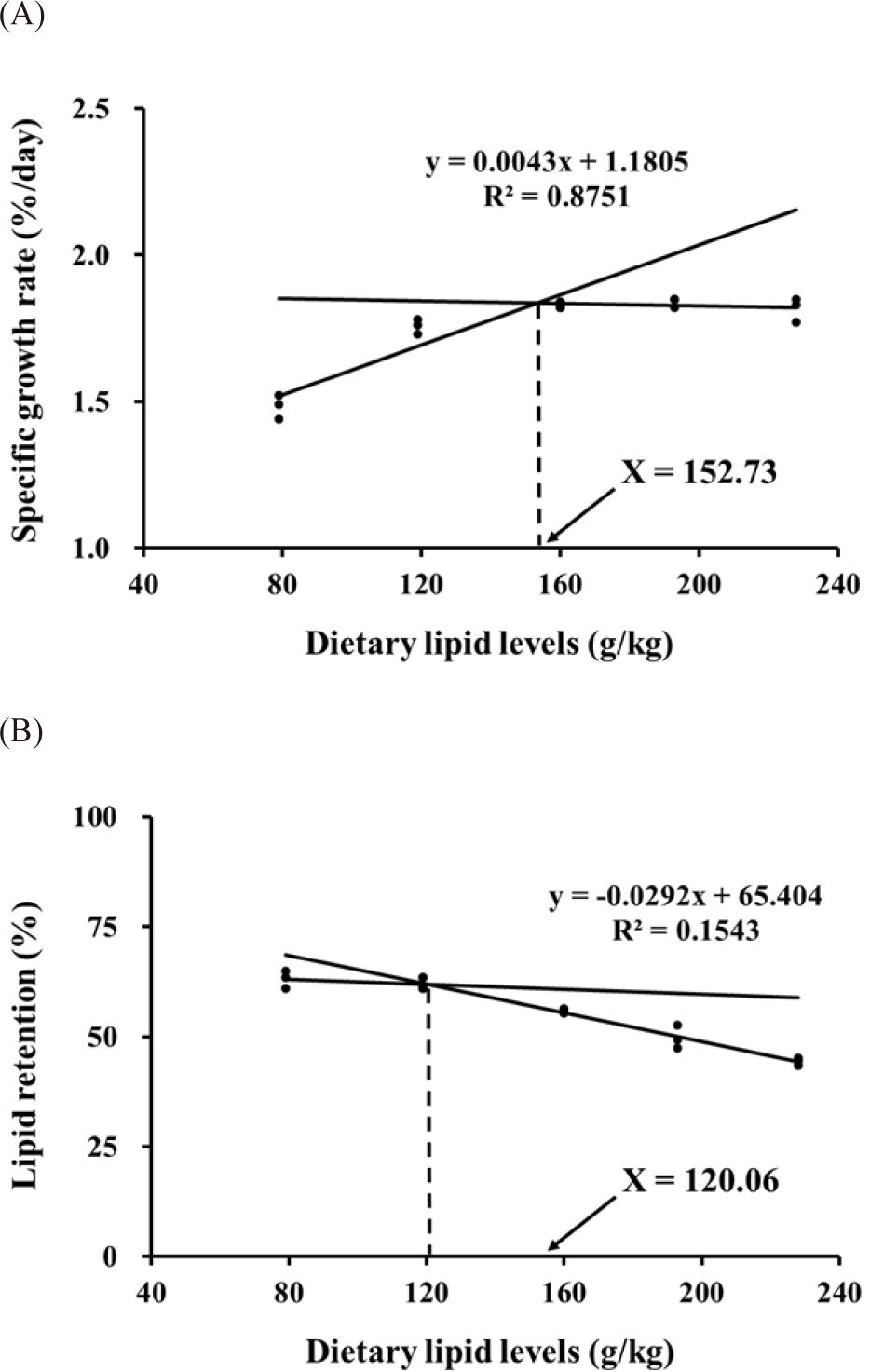
The L160, and L240 groups showed significantly lower whole-body crude protein than that of the L80 group (Table 3, p < 0.05). The crude lipid in whole-body was increased as lipid levels in diets increased to 160 g/kg. The L160, L200, and L240 groups showed significantly higher crude lipid than that of the L80 group (p < 0.05). The L120, L160, L200, and L240 groups showed significantly higher whole-body moisture than that in the L80 group (p < 0.05). The whole-body ash was not significantly different among all the groups (p > 0.05). The L160, L200, and L240 groups showed significantly higher liver fat levels than that of the L80 group (p < 0.05).
The L200 group showed significantly higher HSI than that of the L80 group (Table 4, p < 0.05). VSI of fish was increased as lipid levels in diets increased. The L160, L200, and L240 groups showed significantly higher VSI and condition factor than those of the L80 group (p < 0.05). The L200, and L240 groups showed significantly higher VF than that of the L80, and L120 groups (p < 0.05).
Discussion
Fish growth is the most important factor in aquaculture and is a criterion for determining the optimum lipid level in the feed. The optimum lipid levels in aquafeeds are known to largely differ among fish species. Chang et al. (2018) suggested that 173.8–195.0 g/kg of lipid in diets are optimal for the maximum growth of Manchurian trout (Brachymystrax lenok) in juvenile stage (0.54 g). The optimum lipid level in feed for the maximum growth of sub-adult (233 g) triploid rainbow trout (Oncorhynchus mykiss) was suggested to be 233 g/kg (Meng et al., 2019). The optimum lipid level for juvenile (21.82 g) largemouth bass (Micropterus salmoides) feed based on weight gain was suggested to be 184.2 g/kg (Guo et al., 2019). The effect of lipids in fish growth is associated to the high energy level of lipids. Fish fed diet with suboptimal levels of lipids use protein for energy instead of tissue growth (Deng et al., 2011). In addition, a lack of lipids in feed may not meet the essential fatty acid requirements of fish, which leads to poor growth (Ghanawi et al., 2011). The high energy density and protein sparing effect of lipids can be responsible of fish growth through sparing dietary protein used as an energy source (Weihe et al., 2019). This may explain the high growth of fish fed the L160, L200, and L240 diets in this study. In this study, P/E ratio in the feeds were decreased with increasing dietary lipid levels, which directly indicated protein sparing effects of the feeds. Liu et al. (2021) demonstrated that the growth of northern whiting (Sillago sihama) was significantly increased with increasing lipid levels and decreasing P/E ratio in the feeds. Thus, higher growth of the fish in high lipid groups in this study may be promoted by protein sparing effect with high energy contents in the feeds. The broken line regression analysis clearly indicated that the optimum lipid level in feeds for maximum growth of juvenile parrot fish would be 152.73 g/kg.
The excessive lipid supplementation in fish feeds may have reduced feed intake, nutrient digestion and lipid absorption and assimilation (Yan et al., 2015). In addition, excessive levels of dietary lipid can cause obesity, tissue oxidative stress and steatosis (Wei et al., 2017). Common sole (Solea solea) fed a high-lipid diet showed intestinal steatosis, cellular engulfment and lower feed intake (Bonvini et al., 2015). Ren et al. (2021) emphasized that growth of juvenile (14.19 g) Siberian sturgeon (Acipenser baerii) fed diets with excessive levels of lipids (208.4–248.8 g/kg) was decreased than that in fish fed diets with optimum levels of lipids (169.9–176.0 g/kg). Wang et al. (2021) demonstrated that the same phenomenon was observed in juvenile spotted knifejaw (6.26 g) when the dietary lipid levels were higher (160.8–189.9 g/kg) than optimal level (129.3 g/kg). In this study, LER of fish was also decreased with increasing dietary lipid level. In addition, LR of fish in this study was significantly decreased from the L120 group to L240 group, indicating a decrement in lipid utilization. Consequently, the optimum dietary lipid level of juvenile parrot fish was estimated to be 120.06 g/kg, based on LR results. However, growth and feed utilization were not reduced in parrot fish fed 160–228 g/kg of high lipid diets in this study. Dietary lipids can be used as energy sources instead of proteins in the body, lowering feed costs by the protein sparing effects. Supplementation up to 240 g/kg lipids in juvenile parrot fish feeds may allow for reducing feed cost and sparing proteins.
Liver and intestine are main organs of lipid and glycogen storage and can be used as indicators of energy status in fish (Chen et al., 2013). Excessive lipid supplementation impairs lipid metabolism in fish intestine, which may lead steatosis (Bonvini et al., 2015). Lipid accumulation in fish body was frequently described through HSI and VSI (Thirunavukkarasar et al., 2022). Also, liver fat level and HSI were increased in Manchurian trout fed diet with excessive levels of lipid (Chang et al., 2018). Diets with high lipid levels were reported to increase lipid accumulation in the carcass of silver barb (Puntius gonionotous) (Nayak et al., 2018) and blunt snout bream (Megalobrama amblycephala) (Huang et al., 2022). Lipid accumulation in fish liver, visceral cavity and fillet can become highly rancid and unstable during a long storage (Martins et al., 2007). Lipid peroxidation and free radical production from accumulated fat can drive oxidative stress in tissues (Yin et al., 2021), disrupt the intestinal microflora and reduce performance in fish (Wang et al., 2017). In this study, carcass lipids and organosomatic indices were increased as the lipid level in diets increased to 160 g/kg, but were not significantly different among the L160, L200, and L240 groups. Thus, up to 228 g/kg of lipid in diets seems to have no negative effect on parrot fish. In conclusion, the optimal lipid level for maximum growth of juvenile parrot fish seems to be as 120–153 g/kg diet on the basis of SGR and LR.