Introduction
Ascorbic acid (AA), vitamin C, is an essential micronutrient for normal immunological, physiological and metabolic functions, including anti-inflammatory activities, antioxidant activities, disease resistance, stress response, wound healing and collagen, carnitine and norepinephrine biosynthesis (Marik, 2020). It plays a vital role as a cofactor for enzymes involved in gene transcription, protein synthesis, epigenetics and neurotransmission (Carr & Vissers, 2013). Most teleost fish species cannot synthesize AA because of the lack of L-gulono-1,4-lactone oxidase that catalyzes the final step of the AA synthesis pathway (Dabrowski et al., 1990). Therefore, optimum dietary levels of AA should be supplied through the diet to maintain the normal growth and health of fishes (Lee & Dabrowski, 2004; Lee et al., 1998). The optimum dietary AA requirements differ widely among species. For example, the AA requirements of several fish species are as follows: 23.8 mg/kg for large yellow croaker (Pseudosciaena crocea) (Ai et al., 2006), 107 mg/kg for juvenile red seabream (Pagrus major) (Ren et al., 2008) and 1,000 mg/kg for Nile tilapia (Oreochromis niloticus) (Mustafa et al., 2013).
Citrus by-product (CBP) comprises of peel, rag, pulp and seeds. Processed CBP was investigated as an ingredient in the diets for terrestrial farm animals because of its nutritional and medicinal values (Bampidis & Robinson, 2006; de Evan et al., 2020). Although CBP has been assessed for terrestrial farm animals, limited information is only available for carnivorous aquaculture species (Azizi et al., 2018; Lee et al., 2015). The replacement of AA with CBP in aquafeeds might be a sustainable approach since the required dietary AA amount is comparatively low in most aquatic species and CBP contains a considerable amount of AA (Lim et al., 2024; Zhou et al., 2012). Giger-Reverdin et al. (2002) reported that citrus pulp contains a moderate buffering potential, which is beneficial for regulating gut pH. Citrus peel is a digestive enhancer and a traditional remedy for various pathologies (Choi et al., 2007). Jeju, Korea, is famous for citrus farming and processing and this industry generates 40,000 MT of citrus peel by-products annually (Kang et al., 2006). Red seabream is a predominant mariculture species in East and Southeast Asia (Yi, 2019). There is great potential for CBP to subsidize or replace synthetic AA and to provide additional nutritional and health benefits for red seabream. Therefore, this study was designed to evaluate the potentiality of replacing dietary synthetic AA with CBP and the effects on growth performance, innate immunity and disease resistance against Streptococcus iniae in red seabream.
Materials and Methods
Six semi-purified experimental diets were prepared and diet formulations are presented in Table 1. Three diets were formulated incorporating 30, 90, and 300 mg/kg AA (AA30, AA90, and AA300, respectively) and the other three diets were formulated replacing AA with CBP to contain the same levels of AA in the diets (CBP30, CBP90, and CBP300, respectively). AA was purchased from Sigma-Aldrich (purity 99%; CAS number, 50-81-7; St. Louis, MO, USA) and CBP was provided by Agricultural ILHAE (Jeju, Korea). The AA content of CBP was 4,500 mg/kg (4.5%). The analyzed AA contents in the AA30, AA90, AA300, CBP30, CBP90, and CBP300 diets were 55.9, 100.9, 207.6, 67.6, 117.1, and 300.7 mg/kg, respectively. Vitamin-free casein (Sigma-Aldrich) and defatted fishmeal were used as the main protein sources and squid liver oil was used as the main lipid source for the diet formulation (45% crude protein and 15% crude lipid). Initially, dry ingredients were thoroughly mixed and squid liver oil and distilled water were added to produce a wet dough. Then, it was pelleted through a pelletizer (SP-50, KumKang Engineering, Daegu, Korea) in 3 mm diameter size, dried with an electric drier (SI-2400, Shinil General Drier, Daegu, Korea) at 25°C for 8 hours and stored at –24°C until use. The moisture and ash contents of the diets were analyzed using the AOAC standard procedures (1995). The Kjeldahl method (N × 6.25) was used to analyze crude protein content and the method described by Folch et al. (1957) was used to determine crude lipid content.
3) Vitamin Mix (ascorbic acid-free, g/kg mixture): retinyl acetate, 1.0; cholecalciferol, 0.05; menadione, 0.2; thiamine hydrochloride, 4.0; riboflavin, 4.4; D-pantothenic acid hemicalcium, 14.5; pyridoxine hydrochloride, 4.0; cyanocobalamin, 0.01; niacinamide, 30.0; folic acid, 0.48; D-biotin, 0.2; myo-inositol, 40.0; a-tocopherol, 10.0.
The authors complied with all the applicable national and international guidelines for animal welfare and the experimental protocols were approved by the Institutional Animal Welfare and Use Committee of Jeju National University (approval no: 2023-0054). A set of red seabream juveniles was purchased from a private hatchery (Tongyeong, Korea) and transported to the research facility (Marine Science Institute of Jeju National University, Jeju, Korea). Fish were fed a commercial diet (crude protein, 55%; crude lipid, 12%; Suhyup Feed, Korea) for two weeks to acclimate to the experimental conditions. Five hundred and forty fish (initial mean body weight, 30.5 ± 0.4 g) were randomly assigned to eighteen circular polyvinyl tanks (215 L) in triplicates. Tanks were supplied with sand-filtered continuous seawater flow at a flow rate of 3 L/min and with aeration to maintain sufficient dissolved oxygen level. During the feeding trial, water temperature, dissolved oxygen, salinity, pH and ammonia levels were 17.0 ± 2.0°C, 9.67 ± 0.07 mg/kg, 31 ± 1 ppt, 8.2 ± 0.3 and 0.063 ± 0.007 mg/kg, respectively. A photoperiod of 12:12 h light/dark was maintained using fluorescent lights. The experimental diets were fed to apparent satiation twice daily for 12 weeks (at 08:00 and 17:00 h) at a 3%–5% body weight feeding ratio. Uneaten feeds were collected after 30 min of feeding, air-dried and weighted to calculate the exact feed intake. Fish were weighed once every three weeks and were starved for 24 h prior to weighing.
At the end of the feeding trial, fish in each tank were counted and bulk-weighed to measure growth parameters, including final body weight (FBW), protein efficiency ratio (PER), feed conversion ratio (FCR) and survival. Three fish per replicate tank (nine fish per dietary treatment) were randomly selected and anesthetized with 100 ppm 2-phenoxyethanol (122-99-6, Sigma-Aldrich). Blood samples were drawn from the caudal vein using a heparinized syringe to determine respiratory burst activity. Another set of blood samples was collected from nine fish per dietary treatment using non-heparinized syringes to separate the serum. These samples were allowed to clot at room temperature for 30 minutes and centrifuged at 5,000×g for 10 minutes. The separated serum samples were stored at –70°C to determine non-specific immune responses, including superoxide dismutase (SOD), lysozyme, antiprotease and myeloperoxidase (MPO) activities.
Total AA contents in the test diets and liver samples were analyzed following the 2,4-dinitrophenyl hydrazine (DNPH) colorimetric method described by Dabrowski & Hinterleitner (1989). Bone collagen content in the vertebral columns was determined following the method described by Wilson & Poe (1973) with slight modifications suggested by Ai et al. (2006).
Phagocytic activity was determined by nitro-blue tetrazolium assay (NBT) using whole blood as described by Anderson & Siwicki (1995). The turbidimetric method described by Sankaran & Gurnani (1972) was used to assess serum lysozyme activity. MPO activity was determined according to the method described by Quade & Roth (1997). The antiprotease activity in fish serum was measured as reported by Ellis (1990). SOD activity was determined using a commercial SOD assay kit (DoGen, Seoul, Korea).
Following the 12-week feeding experimental period, fish remaining in each treatment were pooled. 45 fish per treatment were randomly captured and redistributed into fiberglass tanks (15 fish per 240 L tank) to be triplicated treatments for the challenge experiment. The water temperature, dissolved oxygen level, pH and salinity were 17.0 ± 2.0°C, 9.01 ± 0.57 ppm, 7.9 ± 0.2 and 31 ± 0.2 ppt during the challenge study period. For the challenge test, Streptococcus iniae (Korean Collection for Type Cultures, Jeongeup, Korea) bacteria was used as the bacterial pathogen. The two-week LD50 bacteria density was determined in a preliminary test using different bacteria concentrations. Bacteria were cultured in tryptic soy broth (BD, Sparks, Philadelphia, MD, USA) culture medium for 48 h at 25°C shaking at 140 rpm. Cultures were grown until they developed an optical density of 1.2 at 540 nm, resulting in a concentration of 1 × 108 CFU/mL, identified as the optimum LD50 dose in the preliminary test. All the fish were intraperitoneally injected with 0.1 mL of S. iniae suspension (1 × 108 CFU/mL). Fish behavior and mortality were monitored daily and recorded for the following 16 days. Fish were fed two times a day (08:00 and 17:00 h), monitoring the actual reduced feed intake. The liver homogenates of dead fish were streaked on modified selective agar to confirm the pathogenicity of S. iniae.
A randomized complete block design was applied at the feeding trial and challenge test to control the extraneous variabilities and isolate the treatment effect. Data are presented as mean ± standard deviation and percentage data were arcsine transformed before statistical analysis. Data from each treatment were subjected to one-way analysis of variance (ANOVA), two-way ANOVA and correlation analysis where appropriate. When ANOVA identified that overall mean differences of treatments were significant (p < 0.05), the Fisher’s least significant difference test was used to compare the mean values between individual treatments. Statistical analysis was performed using the SPSS (version 24.0, IBM, Chicago, IL, USA) statistical software.
Results
Following the 12-week feeding trial, no significant differences (p > 0.05) in FBW, FCR, PER, and survival were observed among all the dietary treatment groups (Table 2). AA level or AA source did not influence those parameters and there was no interaction between ascorbic level and source. Bone collagen concentration (Table 3) was not significantly affected (p > 0.05) by the dietary treatments. Neither inclusion level nor source of AA significantly affected (p < 0.05) bone collagen content. Liver AA levels in AA90 and AA300 groups were significantly higher (p < 0.05) than in other fish groups and the highest levels were observed in AA300 group. These results showed that dietary AA level and source significantly affect (p < 0.05) liver ascorbic level. Moreover, there was a significant interaction (p < 0.05) between dietary AA level and source.
AA30, AA90, and AA300 were 30, 90, and 300 mg/kg of ascorbic acid incorporated diet groups and CBP30, CBP90, and CBP300 were citrus by-products incorporated diets replacing ascorbic acid levels in the AA diets.
The results of non-specific immune parameters, phagocytic activity and antioxidant capacity are shown in Table 4. Lysozyme activity was significantly higher (p < 0.05) in fish fed AA300 diet than in other groups, except for the CBP300 group. Results suggested that dietary AA level affected the lysozyme level but not the source. Fish fed AA300 and CBP-supplemented diets showed significantly higher (p < 0.05) SOD activity than fish fed AA30 diet. Here, both dietary AA level and source influenced the SOD activity, with no interaction between the two factors. NBT activities of fish fed AA90, AA300, and CBP90 diets were significantly higher (p < 0.05) compared to those fed AA30 diet. Results showed a significant interaction between dietary AA level and source for NBT activity. MPO and antiprotease activities were not significantly affected (p > 0.05); however, the CBP groups showed numerically higher values. Survival data for fish challenged against S. iniae for 16 days were 44%, 58%, 53%, 33%, 55%, and 72% in AA30, AA90, AA300, CBP30, CBP90, and CBP300, respectively (Fig. 1). However, no significant differences were found among treatments in the survival percentages of fish during the challenge test.
AA30, AA90, and AA300 were 30, 90, and 300 mg/kg of ascorbic acid incorporated diet groups and CBP30, CBP90, and CBP300 were citrus by-products incorporated diets replacing ascorbic acid levels in the AA diets.
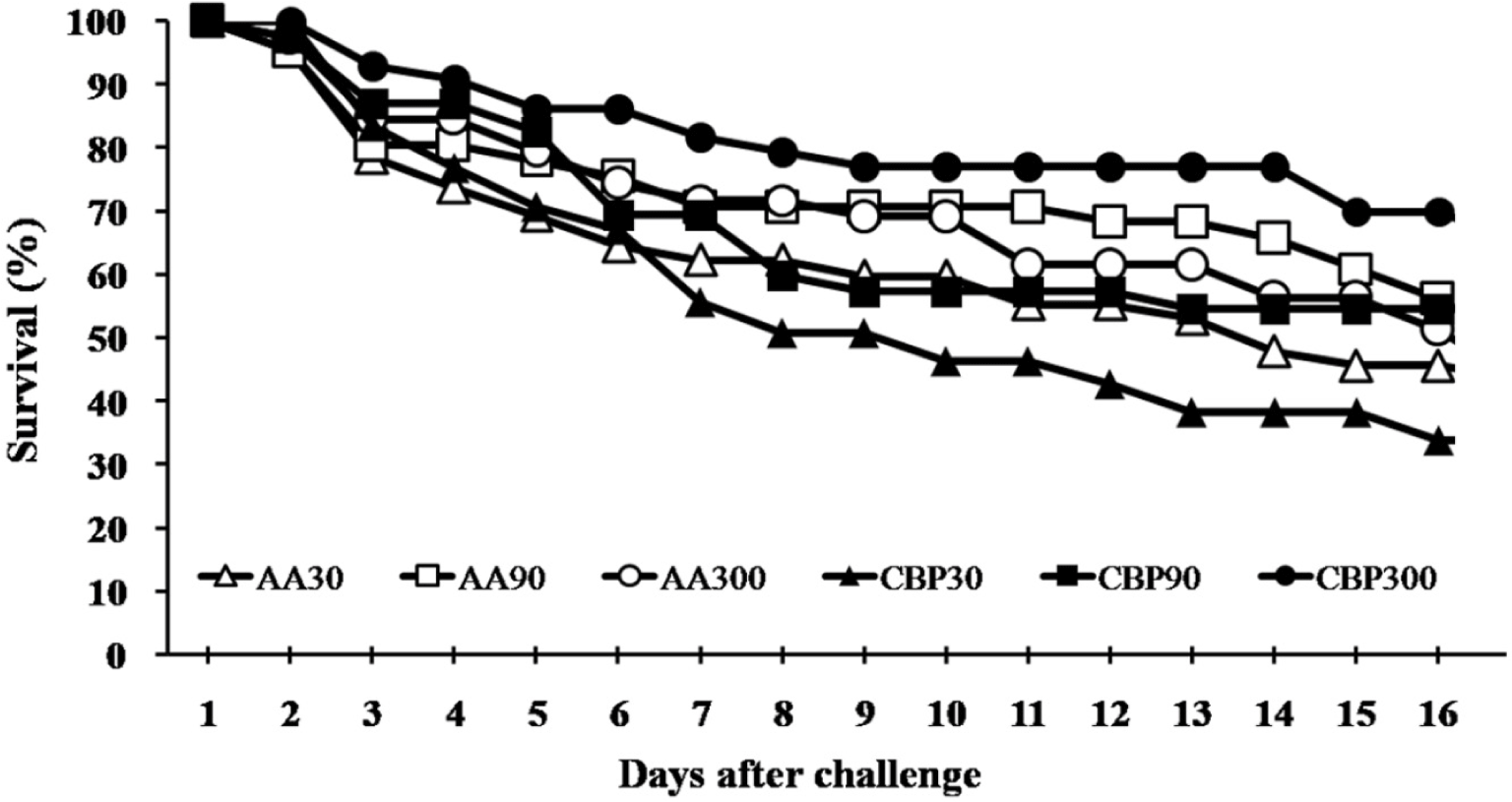
Discussion
Growth performance and feed utilization, including FBW, FCR, PER, and survival, were not significantly affected in any experimental groups. Previous studies showed that dietary AA is essential for the growth of fish (Marik, 2020). However, increasing dietary AA levels did not show any significant difference in the growth parameters of some fish species, including gilthead seabream (Sparus auratus L.) (Henrique et al., 1998) and Atlantic salmon (Salmo salar L.) (Thompson et al., 1993). Henrique et al. (1998) showed that increasing dietary AA levels enhanced liver and spleen AA deposition in gilthead seabream. Furthermore, they suggested that deposited hepatic AA can fulfill the AA requirements for growth during dietary AA deprivation. In the present study, feeding the fish to apparent satiation with even the lowest AA levels of diets (55.9 or 67.6 mg/kg for AA30 and CBP30, respectively) may have deposited enough AA deposition in the liver and maintained, which may have released adequate AA amounts to sustain normal metabolic activities for the growth. The different dietary forms of AA did not significantly affect growth performance and feed utilization. Similar results were reported by Lee et al. (2015) and Lim et al. (2024). Pei et al. (2020) suggested that flavonoids in CBP increase AA deposition in specific organs by enhancing AA absorption. In the current study, the fish in all dietary groups showed no AA deficiency symptoms during the 12-week feeding trial. Therefore, the results demonstrated that CBP is a potential AA replacer in the diet for red seabream.
The liver is an important organ for AA regulation, maintenance of AA homeostasis and AA distribution in the body (Lindblad et al., 2013). In the present study, liver AA deposition was increased linearly with increasing AA levels but not with increasing CBP levels. Previous studies showed that liver AA levels were increased with increasing dietary AA levels (Ai et al., 2006). Synthetic AA and natural plant-derived AA are chemically identical; however, their bioavailability can be reduced by the fiber content and phytochemicals contained in plant sources, allowing AA to be excreted through feces (Carr & Vissers, 2013). Vissers et al. (2011) showed that serum concentrations below saturation level drastically reduced the liver, serum, heart, whole-blood and kidney AA concentrations in gluconolactone gene knocked-out mice, which display genetic characteristics similar to red seabream. Therefore, low liver AA deposition in fish fed the CBP-containing diet might be attributed to the retardation of AA absorption by the relatively high dietary fiber and flavonoid contents in CBP. However, high hepatic depositions do not exert adverse effects because AA can be efficiently excreted from the body owing to its higher water solubility (Gordon et al., 2020).
Procollagen is a precursor of collagen, essential for forming connective tissue, bone matrix and scar tissue (Canty-Laird et al., 2012). AA acts as a cofactor in the hydrolysis of proline and lysine to hydroxyproline and hydroxylysine in collagen synthesis. In the present study, no significant difference was observed in the bone collagen content of red seabream fed different levels of AA and CBP. NRC (2011) reported that lack of AA in the diet impaired collagen synthesis, leading to lordosis, scoliosis, altered pigmentation and broken back syndrome in fish species including, Indian major carp (Cirrhinus mirigala), Nile tilapia, red drum (Sciaenops ocellatus) and Japanese sea bass (Lateolabrax japonicus). In the present study, no significant differences in bone collagen content or signs of deficiency were observed in any of the six treatment groups. Thus, CBP might be a viable alternative to replace AA in red seabream diets without affecting collagen synthesis. Notably, the analyzed dietary AA content in AA diets was lower than that in CBP diets (Table 1), which may be attributed to the oxidation of synthetic AA during diet making and storage. The higher CBP inclusion levels (CBP90 and CBP300) showed no deviations between analyzed AA content and inclusion levels in the diets compared to synthetic AA-included diets. This might be because plant sources are more resistant to AA degradation.
The different dietary forms of AA showed no significant difference in lysozyme, NBT, MPO, and antiprotease activities between the treatments; however, the dietary levels affected lysozyme, SOD and NBT activities. Previous studies showed that dietary AA supplementation at optimum or overdose levels boosts innate immunity and antioxidant enzyme activities in red seabream (Ren et al., 2008). In the present study, red seabream fed AA300 and all CBP diets showed significantly higher SOD activity than fish fed the AA30 diet. Salem et al. (2019) observed similar trends in gilthead seabream and suggested that serum antioxidant capacity may have been increased due to the chemical constituents present in the orange peel, such as flavonoids. Mousavi et al. (2019) reported that dietary AA and flavonoids significantly enhance phagocytic cell proliferation and serum antioxidant and lysozyme activities. They suggested that this phenomenon could be due to enhanced phagocytic cell proliferation induced by AA and flavonoids. Phenolic compounds in fruits and vegetables can increase serum total antioxidant capacity (Kruk et al., 2022). In the present study, the presence of polyphenols, flavonoids, AA and other vitamins in CBP may have influenced the serum lysozyme and antioxidant enzyme activities in red seabream. Besides replacing AA in the diet, the increased health status of fish with CBP is an additional benefit.
Dietary AA can act as an antimicrobial, antiviral, antifungal and antiparasitic agent in animals (Mousavi et al., 2019). Cobia (Rachycentron canadum) and yellow croaker fed diets supplemented with AA showed significantly increased disease resistance against Vibrio harveyi (Ai et al., 2006; Zhou et al., 2012). In the present study, increasing levels of synthetic AA in the diet did not significantly affect survival during the challenge test. However, replacing synthetic AA with CBP increased the survival. Citrus fruits are rich in various health-promoting phytochemical compounds (Baba et al., 2016). Salem et al. (2019) observed a significant reduction in intestinal bacterial populations of Staphylococcus aureus, Vibrio, Salmonella and Shigella in gilthead seabream fed a diet containing dried orange peel. Pei et al. (2020) demonstrated that flavonoids in citrus fruits have a high potential to boost fish health and reduce mortality by inhibiting bacterial infections. The higher survival of CBP groups than AA groups may be attributed to flavonoids and AA in CBP.
In conclusion, CBP showed great potential to replace AA in the diet for red seabream without affecting the growth performance, feed utilization, bone collagen content, liver AA deposition and innate immune responses.