Introduction
The Asian Moon Scallop (Amusium pleuronectes) is a bivalve mollusc of the Pectinidae family. The genus Amusium belongs to the family Pectinidae Rafinesque, 1815, subfamily Pectininae Rafinesque, 1815, Tribe Amusiini Ridewood, 1903. Five genera were included in the Tribe Amusiini: Amusium Röding, 1798; Dentamussium Dijkstra, 1990 and Ylistrum Mynhardt & Alejandrino, 2014 were distributed in the West Pacific; Euvola Dall, 1898 and Leopecten Masuda, 1971 were distributed in the East Pacific. The genus Amusium is monospecific, A. pleuronectes. This scallop is native to the western Pacific Ocean, particularly the coastal waters of Japan, China, Korea, Russia, and Indonesia.
According to the World Register of Marine Species, several species known from Amusium genus, but these have been accepted as different genus such as A. balloti, A. andamanense, A. fenestratum, A. japonicum, A. laurenti, A. taiwanicum. A.balloti accepted as Ylistrum balloti, Amusium andamanense accepted as Propeamussium andamanense. Asian moon scallops or A. pleuronectes is a benthic organism that can swim or glide to avoid predators or to find a suitable habitat. This swimming or gliding bivalve lives in 6–160 m depth on a sandy-mud substrate, widely distributed in the Indo-West Pacific, from the Red Sea to the West Pacific (Indonesia, Philippines, Vietnam, Japan, Taiwan, and Australia). A. pleuronectes was found in Central Java, Bali, Sulawesi, and Maluku (Hardianto & Satriyo, 2023). For seasonal migration or reproduction, A. pleuronectes is able to glide at speeds ranging from 37–45 cm/second, even recorded at 73 cm/second, while average swimming time was 8–10 second, covering 10 m distance (Morton, 1980). A. pleuronectes boasts one of the most striking swimming abilities compared to other scallops despite having the smallest genome size (Li et al., 2022).
The main destination countries for Indonesian shellfish exports are Malaysia and Thailand, followed by the United States, East Asia, and Canada. The volume of Indonesian shellfish exports in 2019 was 13.57 thousand tons, valued at $ 17.3 million. The projected trend is to increase shellfish production by 12% annually, namely around 87 thousand tons in 2020 to 137 thousand tons in 2024 (Rahman, 2000). Aquaculture is one source of seafood production in addition to fishing; in the past, production increased considerably. The world production from aquaculture of fish and shellfish in 1997 was 36 Mt, where scallops were 3% (Naylor et al., 2021). Furthermore, in their review of the global production of fish and shellfish from aquaculture in 2017, the authors reported that 112 Mt increased the total production, where there was a scallop of 3%.
The Indonesian government has proposed and applied several strategic initiatives to advance shellfish cultivation and fisheries, including scallops, emphasizing both sustainable development and economic growth. Several key efforts include establishing and managing Fisheries Management Areas (WPP), increasing shellfish production, supporting business growth, enhancing investment and market access, and developing infrastructure and regional connectivity. Additional priorities focus on managing fish health and environmental quality, improving human resource competencies, advancing science and technology, and reinforcing institutional and stakeholder commitment. To support these initiatives, a co-management approach that incorporates the expertise of academia, business, government, and the community (ABGC model), has been implemented to promote scallop cultivation in Brebes Regency, Central Java (Susilowati et al., 2008). Other approaches, such as spatial modelling, have also been employed to identify and map suitable scallop habitats, for example, by analyzing the environmental characteristics of scallop habitats in the Brebes Regency (Sahri et al., 2014). Together, these efforts are means to develop sustainable scallop aquaculture.
To support the further development of the scallop industry, this article reviews currently available scientific findings on the biology, fisheries, and aquaculture of A. pleuronectes and other scallop species. The review elaborates on the reproductive cycles and farming systems of A. pleuronectes and other scallop species. Additionally, we also elaborate on the influence of environmental conditions and disease. By consolidating existing knowledge, this review article aims to provide a comprehensive overview of the current status and to highlight key areas for future research and improvement in scallop fisheries and aquaculture, particularly for the Asian moon scallop.
Reproductive Cycle
Asian moon scallop is an important artisanal fishery in South-East Asian countries such as Indonesia (Susilowati et al., 2008), Thailand (Wells et al., 2021), and Philippines (Bobiles & Soliman, 2018; del Norte, 1988). Overfishing (Bobiles & Soliman, 2018) and accidental catch (Fauziah et al., 2022) lead to the diminishing natural stock. To restore natural stock population, regulation of Asian moon scallop fishing and establishment of marine culture is of utmost importance. Information about the reproduction cycle will help to regulate the no-fishing period (Huang et al., 2015) and establish breeding programs (Dukeman et al., 2005).
The Asian moon scallop has a functional hermaphrodite gonad, both testes and ovarian tissues develop and mature at the same time (Coe, 1943). The testes tissue occupies the base and ventral part of the gonad, while the ovarian tissue is located in the dorsal and anterior part of the gonad. There is no definite separating line between both sex tissues and there is no mixing between the germ cells inside each sex tissue (Reddiah, 1962). The testes tissue is white, while the ovarian tissue is orange. Both sex tissues turn to bright, creamy white or creamy orange and become bulkier when the gonad is mature. The stages were determined by the shapes and colors of the gonads, as follows Stage 0 (Immature), Stage I (Developing), Stage II (Differentiated gonad), Stage III (Recovering), Stage IV (Filling), Stage V (Half-full), and Stage VI (Full) (Mason, 1958).
Based on monthly collection of gonad samples, the qualitative gonad index of A. pleuronectes hovered above 2.0 throughout the year. The value indicated that in each month, most Asian moon scallops are ripe and ready to spawn (del Norte, 1988). Protrandric hermaphrodite was confirmed through macroscopic observation of gonads. The spawning stage in testes is more noticeable compared to the spawning stage in the ovary and coincides with the ripeness cycle of the ovary (del Norte, 1988).
The main factor influencing spawning for scallops is temperature (Cropp, 1993). Depending on the species, a decrease or increase in temperature provides an environmental cue for spawning. The majority of tropical scallop’s spawn during the higher water temperature while the minority spawn in colder water temperature (Cabiles & Soliman, 2019). For Asian moon scallops, spawning occurred when seawater temperature decreased (del Norte, 1988). In the Philippines, scallops with spawning stage gonad were captured throughout the year. In order to develop the specific production of juveniles in the stock, knowledge about the local structure of the scallop population, the health situation, the reproduction cycle and hatchery performance is mandatory (Magnesen & Christophersen, 2008). The majority of gonad with spawning stage peaked at different months depending on the location. In higher latitudes such as Lingayen Gulf, the majority of the spawning stage was captured in February. The number dropped abruptly from February until May, stagnated until October, and fell to the lowest number in November (del Norte, 1988). In lower latitudes such as the Visayan Sea, the number of scallops with spawning stage peaked a month earlier compared to higher latitudes but followed by nearly similar spawning patterns as in higher latitudes (Llana & Aprieto, 1980).
Media
Scallops, bivalve molluscs from the taxonomic family Pectinidae, have been exploited around the world. For instance, Argopecten irradians has been cultivated in the Sishili Bay, North Yellow Sea, China (Sun et al., 2023). This species has been introduced to Newfoundland, Canada to be cultivated with native scallop Placopecten mageelanicus (Parsons & Robinson, 2006). Nodipecten nodosus that can be found in the East Coast of America has been exploited mainly in Brazil (da Silva et al., 2022). Meanwhile, in Europe, Mimachlamys varia or black scallop is wild captured in Biscay Bay (Zorita et al., 2022). The saucer scallop Amusium balloti is exploited through recreational fishing or even bycatch in Queensland, Australia (Fisheries Queensland, 2011; Richards et al., 2015). In the South China Sea, the Asian moon scallop A. pleuronectes has been exploited by the Southeast Asia region countries, for instance, Phillipines (Bobiles & Soliman, 2018; Rice et al., 1994), and Indonesia (Prasetya et al., 2010). The Asian moon scallop A. pleuronectes has the potential to meet the demand for seafood in Asia. In Indonesia, scallop production through fisheries can reach up to 68.27 ton per year during the sampling year between 2007–2009 from one coastal district in Northern Java (Prasetya et al., 2010).
Various methods have been suggested and performed for scallop husbandry at the sea and landbased facilities around the world. With regard to sea-based husbandry, in general, there are five methods, for instance surface lines, subsurface lines, rafts, racks, and sea ranching. Scallops are placed in a confined compartment such as plastic mesh bag (Fisheries Queensland, 2011), plastic basket or pots (Fig. 1; Zorita et al., 2022), and even stacked of plastic baskets which are called the lantern nets (Fig. 2A; da Silva et al., 2022; Han et al., 2013). The rearing cage then is covered with 4 mm nylon mesh for protection from grazing fish (Rice et al., 1994).
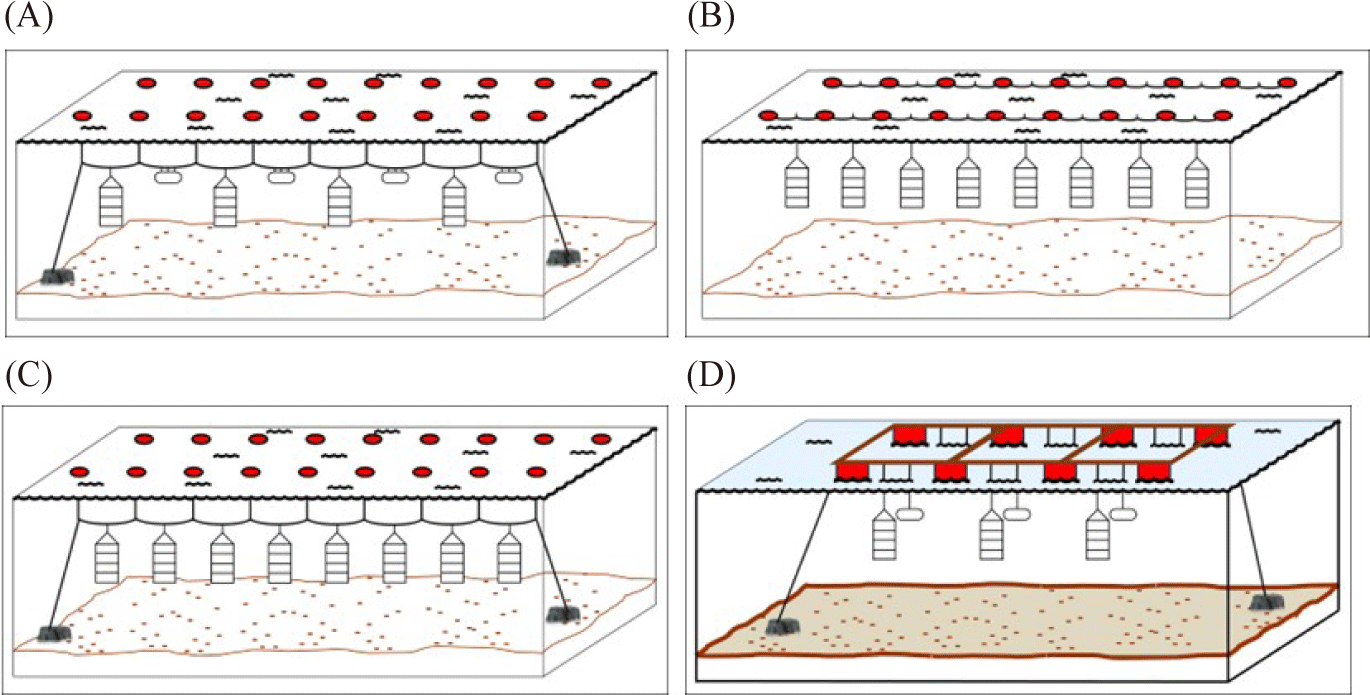
The surface line or longline has a single ‘backbone’ rope with buoys at each end and in between (Fig. 2B and 3A). The rope is then anchored at each end on the sea floor. The scallop rearing cage then is suspended from the ‘backbone’ rope using drop line (Fig. 2C; da Silva et al., 2022; Fisheries Queensland, 2011; Han et al., 2013; Zorita et al., 2022). The suitable depth for the rearing cage range between 8–20 meter where the highest phytoplankton biomass occurred. The rearing cage (the lantern nets or pots) are placed 1 meter apart from another rearing cage. Additionally, it has been suggested that the rearing cage should be 4–6 m above the seafloor (Han et al., 2013). The subsurface line is similar to the surface line. However, the buoy is below the surface to avoid boat traffic disturbance (Fig. 3B; modified from Fisheries Queensland, 2011).
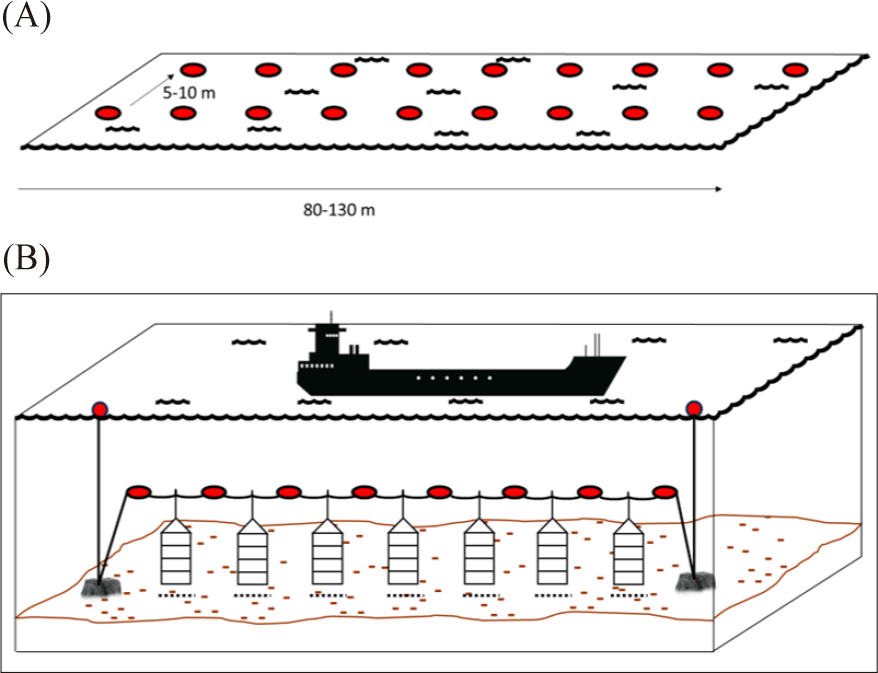
Meanwhile, the raft utilizes a rigid frame structure that is placed on the top of buoys to float above the sea surface (Fig. 2D). The rearing cage then is hung from the raft frame (Zorita et al., 2022). Another rearing structure is the rack. The rack utilizes a rigid frame structure where it is submerged and plugged into the seafloor in the intertidal zone (Fig. 4A). The rearing cage is hung below the sea surface from a line between the frame structure (Fisheries Queensland, 2011). Lastly, the sea ranching or bottom culture where scallops are placed on the seabed using pipe from the boat or hands when diving (Fig. 4B). This method requires no cage and structure during the rearing period and the area is left undisturbed (Fisheries Queensland, 2011; Parsons & Robinson, 2006).
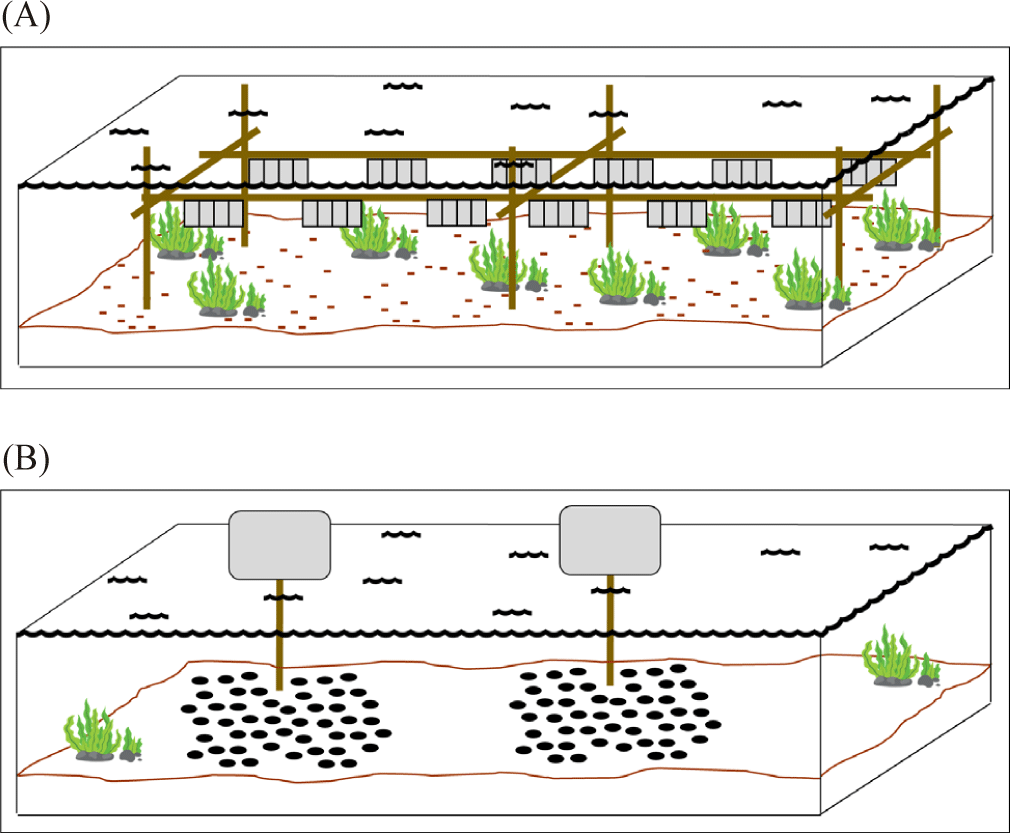
Several aspects should be considered during site selection for the sea-based facilities. The scallop husbandry structure should be placed in the bays or sheltered bays to avoid strong and damaging wave and current (da Silva et al., 2022; Han et al., 2013; Sun et al., 2023; Zorita et al., 2022). Also, the structure should avoid locations where the risk of disturbace is high. Locations such as boat traffic lines, mega marine fauna foraging/migration routes, marine tourism activities, coastal hydraulic impact, polluted area, and even indigenous cultural site should be avoided (Fisheries Queensland, 2011). During the rearing period, the sea based rearing structure should be maintained from fouling organisms that might compete with scallops or hinder water flow that brings phytoplankton through the rearing cage (Parsons & Robinson, 2006). Scallop sea ranching or farming is prone to the predation risk particularly from starfish, crab, or other pradator (Fisheries Queensland, 2011; Parsons & Robinson, 2006). Therefore, to guarantee the success of scallop farming location with low or without presence of predator and fouling organism should be preffered.
Furthermore, due to the filter feeding regime, scallops should be reared where the phytoplankton biomass is the highest (Lodeiros et al., 1998). Phytoplankton density in the rearing area should be around 11,000-14,500 cell.l-1 (Sahri et al., 2014). Previous studies reveal that from the moon scallop gut content analysis, their habitat mainly consists of Bacillariophyta and small amount of Cyanophyta (Taufani et al., 2016). Moreover, during site selection the presence of certain harmful algae should be considered bacause certain algae might contain phycotoxin that harmful to shellfish itself or even humans, particulary during the event of Harmful Algae Blooming (Parsons & Robinson, 2006).
Additionally, the impact of the scallop rearing structure should be limited in order to maintain sustainability of the grow out and husbandry practices. Intensive sea-based scallop culture could potentially alter coastal hydraulic. A massive underwater rearing structure might hinder water mixing and promote stratification. This stratification might induce the formation of hypoxia due to oxygen depletion for respiration by the scallops and other marine organisms (Sun et al., 2023). In addition, shellfish, including scallops, are susceptible to ocean acidification in the future due to sensitivity of biomineralization of the shell to reduce pH seawater. Thus, in the future, hatchery and grow out activities are suggested to be held in the aquaculture facilities so the water quality can be controlled (Richards et al., 2015). Table 1 provides an overview of the cultural systems of several countries.
Species | Culture systems | Countries | Ref. |
---|---|---|---|
Mimachlamys varia | Rearing cages attached to longlines or rafts | Spain | Zorita et al. (2022) |
Chlamys farreri, Argopecten irradians | Rearing cages attached to longlines | China | Han et al. (2013) |
Chlamys farreri | Rearing cages attached to longlines located inshore and offshore | China | Zhang et al. (2011) |
Nodipecten nodosus | Co-culture with seaweed Kappaphycus alvarezii on suspended longlines | Brazil | da Silva et al. (2022) |
Patinopecten yessoensis | Suspended-hanging and bottom-sowing culture systems | South Korea | Uddin et al. (2007) |
Pecten fumatus | Suspended culture with disk culture methods | Australia | O’Connor et al. (1999) |
Placopecten magellanicus | Bottom culture (bottom cages) and suspended culture (pearl nets, lantern nets, suspended cages, and ear-hanging methods) | Canada, US | Coleman et al. (2021); Morse et al. (2020); Parsons & Robinson (2006) |
Placopecten magellanicus | Polyculture of scallops with Atlantic salmon. Suspended culture with pearl nets | US | Parsons et al. (2002) |
Pecten maximus | Suspended culture with rearing nets or cages | Norway | Grefsrud & Strand (2006) |
Pecten maximus | Fertilized shallow seawater pond | Norway | Andersen & Naas (1993) |
Chlamys farreri | Earthen ponds in polyculture with Japanese sea cucumber | China | Ren et al. (2012) |
Pecten maximus | Land-based raceway for spat nursery | Norway | Magnesen & Christophersen (2008) |
Argopecten purpuratus | Closed and recirculating culture systems for larvae | Chile | Merino et al. (2009) |
Feed
Scallops or other bivalves are filter feeders that feed on particulate organic matter (POM) including organic debris and microorganisms. Some of these POMs might come from terrestrial, intertidal, and pelagic (Alma et al., 2023; Ishaq et al., 2023). From the inhalant current of sea water, particulate matters are screened and retained by the cirri on gill filaments. Particles that are more than 4 µm will be retained on cirri and trapped with mucus. This mucus mass then is cilia transported through the oral groove on labial palp toward the mouth opening (Beninger et al., 1990, 1991; Jørgensen, 1996).
Microorganisms that are fed by scallops includes phytoplankton, and its quality and availability drive growth performance of scallop (Poitevin et al., 2022; Velasco, 2007). In some locations particularly in Java Sea, A. pleuronectes had been described to mostly ingest on Coscinodiscus spp., Rhizosolennia spp. and Chaetoceros spp. with index of preponderance (IP) at 27.20%, 23.56%, and 18.43% respectively (Taufani et al., 2016). Improved shell growth had been recorded over the phytoplankton blooms period (Poitevin et al., 2022). This includes harmful algae species, for instance Alexandrium minutum, Alexandrium catenella, Gymnodinium catenatum, and Pyrodinium bahamense (Wang et al., 2021). However, toxin from harmful algal bloom could be incorporated and bioaccumulated in scallop during the filter feeding process (Wang et al., 2021).
Environmental and Disease
Aquatic organisms have varied habitats depending on their physiological characteristics. Type A. pleuronectes and Amusium balloti are spatially distributed and influenced by several environmental factors including depth, substrate, temperature, turbidity, salinity, ocean currents, availability of food, and oxygen content (Brand, 2006; Salgado-García et al., 2023). Several studies revealed the effects of environmental factors in scallops (Table 2).
Species | Factors | Effect | Ref. |
---|---|---|---|
Patinopecten yessoensis | High temperature | TCA cycle was inhibited | Yang et al. (2023) |
High temperature | Enhancing glycolysis pathway of glycogen | Jiang et al. (2023) | |
Nodipecten subnodosus | High temperature & low oxygen | Increased respiratory response | Salgado-García et al. (2023) |
In batch- and flow-through culture and algal diet | Increased in growth | Nava-Gómez et al. (2022) | |
Argopecten purpuratus | Low pH | Changed shell properties increasing microhardness | Córdova-Rodríguez et al. (2022) |
Nodipecten nodosus | Different microalgal diets | Increased in growth | Velasco (2007) |
Argopecten nucleus | Different microalgal diets | Increased in growth | Velasco (2007) |
Pecten maximus | Hydrodynamic cues with controlling water flow | Increased the size of larvae | Tremblay et al. (2020) |
Placopecten magellanicus | Hydrodynamic cues with controlling water flow | Increased the size of larvae | Tremblay et al. (2020) |
Chlamys nobilis | Acidification | Adverse effects on growth rate and survival rate | Zheng et al. (2022) |
Cadmium | Accumulation in viscera and gills | Liu et al. (2023) | |
High stocking density | High mortality, slow growth, induce immunosuppression | Feng et al. (2023; Li et al. (2020) | |
Nodipecten nodosus | Temperature; bacteria | Reduce scallop productivity | Thompson et al. (2023) |
Scallops in the waters of Brebes, Central Java live in a soft or soft mud substrate on the larger Wentworth scale, which is above scale 6 (ҩ > 6; Sahri et al., 2014). This is consistent with similar studies which state that scallop shells are found on various sedimentary substrates in the form of silty loam and silty clay loam with a clay composition of 13.92%–37.76%; Sand 0.08%–0.24%; 62%–86% and organic matter content of 14.25%–16.62% (Taufani et al., 2016). The scallops found in the waters of the Lingayen Bay and Visayan Sea, Philippines are in silty sand to muddy substrate at a depth of 12–40 m (Cabacaba et al., 2018). Hard-shelled clams or bivalves prefer soft and smooth textured substrates such as mud, this is related to their feeding habits and the availability of organic matter derived from the decomposition of organisms that have died and mixed with the sediment substrate. The organic matter content in mud-textured sediments is higher than in sand and rock substrates. This is different from the habitats Pecten maximus which can be found on the seabed with a substrate of hard sand, shell sand, sandy fine gravel, and sometimes in the form of a mixture of while Decatopecten radula or flat ribbed scallops who prefer muddy sand substrates (particles < 63 µm) and fine to coarse sand (particles > 63 µm) accompanied by coral fragments and shells (Cabacaba et al., 2018; Lavaud et al., 2014). Mussels are aquatic biota that are filter feeders so that they can filter food suspended in water in the form of seston, including various types of plankton and detritus deposits measuring less than 1 micron (Ernawati et al., 2017; Lavaud et al., 2014). Substrates containing organic matter such as silt with a darker color are preferred because of making it easier for clams to find food sources. Soft sediment texture is also preferred by benthic creatures such as clams because they contain lots of plankton as a source of nutrition (Sahri et al., 2014). Mussels can filter out micro-particles of nutrients using hundreds of narrow filaments found inside ctenidium or gills while non-nutritious particles will be excreted in the form of pseudo feces (Lavaud et al., 2014).
Habitat-related studies scallop on a more detailed scale, the distribution of individuals and groups are also influenced by the role of habitat structure such as the presence of polychaete niches, aquatic plants/hydroids, sponges, macroalgae, others shellfish, availability of substrate for attachment of shellfish/spat larvae thereby reducing the chances of being eaten by predators, accelerated growth by looking for positions in the water column that have an abundance of quality food and avoid being trapped in soft sediments (Mendo et al., 2014). The role of function of the habitat structure will still be needed until the scallops are juvenile and adult because the characteristics of the habitat will affect the level of predation. Juwana scallops of Argopecten radiate are safer from the threat of predators if they are in the canopy of the Zostera marina. Better protection against predators is obtained by New Zealand scallop because it has a complex habitat structure with large numbers of shells, sponges, and horses, ascidians more species will reduce the visibility of starfish and gastropod predators (Mendo et al., 2014).
Parameters of water quality greatly determine the survival of aquatic biota in it, especially temperature which is a key factor for the growth of ectothermic aquatic biota such as scallops. Observations made by researchers in Brebes, Central Java, stated that scallop shells were found in waters with temperatures between 29.92°C–30.06°C (Sahri et al., 2014). Other studies have shown that there are variations in the temperature of the scallop habitat in Brebes from February to April, namely 28.9°C–35.8°C (Taufani et al., 2016). Meanwhile, research conducted in the Philippines proved that the stability of the maintenance water temperature affected the survival rate (SR) A. pleuronectes where the temperature of 28°C–29°C is the best temperature with an SR value of 90%. The water temperature of 30°C–31°C gives the lowest SR , which is 48% ± 6.48% (Cabacaba et al., 2020). High temperatures will increase the metabolic activity of aquatic biota including A. pleuronectes that live in tropical waters with active swimming properties (Morton, 1980) if the water temperature is high, the interactive energy will easily run out and ultimately reduce their survival ability (Cabacaba et al., 2020). The optimum maintenance temperature A. pleuronectes is in the range of 26°C–29°C and is not recommended at temperatures above 31°C. Water temperatures that are higher than the ambient temperature will reduce the growth rate of scallops (Aguirre-Velarde et al., 2019).
Temperature plays an important role in the physiological processes of the body of aquatic biota to assist important metabolic activities, including enzymatic reactions such as increased activity of hepatopancreatic superoxide dismutase, catalase (CAT) and decreased activity of acid phosphatase, and lysozyme (LSZ) activities and cell membrane permeability (Jiang et al., 2016). Experiments on Patinopecten yessoensis scallops proved that a gradual increase in temperature up to 20°C increased oxygen consumption and nitrogen excretion rate. These two values are indicators of energy and protein use (Jiang et al., 2016).
Changes in seawater temperature in the scallop’s habitat will affect the spawning process. Temperatures that tend to be warmer than usual will encourage the gonadal maturation process and eventually, the female mussels release their eggs and reduce energy for growth (Aguirre-Velarde, 2016).
Recent studies have revealed that scallops from the Pectinidae family, namely P. yessoensis which are exposed to high temperatures up to 8°C from an ambient temperature of 15°C experience obstacles in nutrient absorption caused by increased proliferation of pathogenic microorganisms in their intestines, such as Tenacibaculum and Mycoplasma and impact on death (Kong et al., 2023). The gut and its microbiotas are the first line of defense for an organism in facing environmental stress, so it can be seen that temperature can change feeding behavior, energy metabolism instability, and immune regulation in molluscs. The impact of this response will lead to a decrease in SR, growth and reproductive efficiency (Jiang et al., 2016; Kong et al., 2023; Liu et al., 2021; Salgado-García et al., 2023; Yang et al., 2023).
A. pleuronectes is more commonly found in waters that have an average salinity value between 31.85‰–32.65‰ based on observations in Brebes waters (Sahri et al., 2014) while higher salinity was observed in scallop shell habitat in Brebes waters in February–April namely 33‰–36‰ (Taufani et al., 2016). This is supported by data that scallop shells are more common in remote areas i.e. on average more than 5 km from the estuary. The salinity of the waters will affect the process of osmoregulation of scallops. The experiment about maintaining water salinity reduction in Argopecten purpuratus’s rearing was conducted to determine the tolerance of this biota in terms of growth, O/N ratio (comparison of oxygen uptake and N-ammonia excretion), oxygen uptake, and excretion rate. Salinity is positively correlated with an increase in the excretion rate, O/N ratio, and growth, which means that decreasing salinity will reduce the excretion rate, O/N ratio, and inhibit growth (Mozanzadeh et al., 2021). Research on scallop’s species Mizuhopecten yessoensis in the Sea of Japan, Russia showed that there is a relationship between temperature and salinity on the daily growth of shells. The optimal temperature and salinity to support the growth of scallops are not too low and not too high. The optimum temperature range for shell growth of M. yessoensis is 8°C–16°C and 32.5‰–33.5‰ for optimal salinity (Silina, 2023). Cultivation of scallops for rearing larvae was carried out in the Philippines using a water salinity of 34‰ (Cabacaba et al., 2020).
The water quality parameter that is important to observe for the survival of aquatic biota is the balance of Hydrogen ions in water (pH). Scallops are more tolerant at a pH of 7.3–7.4 (Sahri et al., 2014) where this value is still within the pH range studied in Brebes waters, Central Java in February–April, namely 6.55–8.16 (Taufani et al., 2016). The acidity of seawater greatly affects the life cycle of shelled organisms such as clams. Sea water which tends to be more acidic can reduce the SR, shell growth, calcification balance which ultimately affects the thickness and strength of the shells. The formation of bivalve shells is influenced by the content of carbonate ions if the pH of seawater drops to acid, carbonate ions in the water will decrease and result in slow, thin, and easily broken shell growth. The fragile shell makes it easier for predators to prey on scallops and reduces their abundance in a habitat (Lavaud et al., 2014). Low pH can trigger stress in A. purpuratus due to physiological performance disturbances such as respiration, metabolism, acid-base balance, and biomechanical disturbances (Aguirre-Velarde, 2016; Melzner et al., 2011). Decreasing the pH of seawater can interfere with enzyme activity and metabolic processes and ultimately have an impact on slowing the growth of scallops (Melzner et al., 2011). Larval development in clams P. maximus was disturbed by a decrease in the pH of seawater (Andersen et al., 2013). Acidification of body fluids can disrupt the acid-base balance and physiological functions of the scallop’s body (Waldbusser et al., 2015).
Total suspended solids (TSS) in water mean that there are many solid particles in the water and if the concentrations are too high, they harm aquatic organisms. Research conducted in Brebes waters shows that the value of TSS in the habitat A.pleuronectes/scallop shells ranged from 0.219–0.437 mg/L (Taufani et al., 2016). This is consistent with observations of scallop’s habitat in Brebes waters which is less than 3.61 mg/L. This change in TSS concentration is related to suspended particles in the water column which are carried by physical oceanographic activities, sea waves, and sediment particles from the mainland which are carried by rainwater and then flow toward the river estuary (Sahri et al., 2014). Scallops are tolerant in waters with TSS values that tend to be low as described in a study on P. maximus which experienced changes in behavior such as more frequent shell clap movements after being placed in aqueous media with high TSS values 200–700 mg/L (Szostek et al., 2013). The shell clap mechanism or the movement of fully closing the clamshell is a mechanism Covered equally to clean the mantle from excess sediment that enters due to the high TSS value (71 mg/L) (Last et al., 2011).
Too many suspended particles will make it difficult for the scallops to obtain food because the scallops will filter out organic particles suspended in the water column so that their intake and growth are disrupted (Coleman et al., 2021; Ribó et al., 2021). Solid particles from suspended sediments in the water column can also reduce the dissolved oxygen in the water.
The solubility of oxygen in water or DO plays an important role in the survival of the biota in it. The oxygen content in water is influenced by two factors, namely physical processes such as aeration and diffusion and biological processes such as photosynthesis and respiration. Dissolved oxygen content can experience depletion or decrease triggered by changes in temperature that are influenced by the seasons; primary production by photosynthetic activities carried out by phytoplankton and the presence of atmospheric input as the main source of dissolved oxygen and respiration in the water column such as respiration by microbes which are the main consumers of oxygen in water. Warmer temperatures reduce the ability of water to hold gas solubility in water and increase microbial respiration so that the use of dissolved oxygen increases. This causes a decrease in dissolved oxygen levels in seawater, such as what happened at the A. irradians mussel cultivation site in the North Yellow Sea, China (Yang et al., 2021).
Marine organisms often face changes in the combination of fluctuating temperature and dissolved oxygen levels, especially after the emergence of the global warming phenomenon. These events include warming seawater temperatures followed by a decrease in DO, if the value is less than 5 mg/L (DO < 5 mg/L) it is called moderate hypoxia, and if the value is less than 2 mg/L it is called severe hypoxia (DO < 2 mg/L). Meanwhile, the event of an almost complete decrease in oxygen levels is called anoxia and a series of events that decreases DO can affect the behavior, distribution, and abundance of marine organisms (Salgado-García et al., 2023). The response of marine organisms in facing hypoxia is specific based on their body tissues, some of which function as a ventilator, and oxygen transport will increase the rate of ventilation and circulation to support oxygen supply and meet the increased oxygen demand at higher temperatures (Salgado-García et al., 2023). Aerobic metabolism and several physiological processes of the scallop’s body such as growth, energy for movement, and reproduction processes where these processes require a lot of energy will be hampered and endangered by limited oxygen intake in the body and the environment. Hypoxia conditions in water will reduce the availability of food particles in the water column thereby reducing the feeding rate of scallops. The stress level of scallops increases with limited oxygen, thereby reducing their immunity and ultimately being susceptible to disease (Yang et al., 2021). Research on scallop habitat in Brebes waters showed DO values were in the range of 5.25–16 mg/L (Taufani et al., 2016).
The currents of the scallop’s habitat in Brebes are greater than 0.06 m/s as was observed around Brebes more scallops were found on the bottom of the water with current speeds between 0.07–0.09 m/s this relates to the nature of shells as sediment-feeder or the behavior of clams that use ocean currents to take food from seafloor sediments. In addition, ocean currents also play a role in the distribution of food reserves from one location to another (Sahri et al., 2014). Benthic-feeder organism requires ocean currents at the right speed that carry benthos so that food needs will be met. Ocean currents also affect the concentration of phytoplankton and the rate of cleaning (clearance rate) that decreasing sea currents (15–5 cm/sec) results in an increase in the rate of cleaning scallops P. magellanicus by 30% compared to the treatment of increasing sea currents (5–15 cm/sec) with a constant concentration of phytoplankton (Pilditch & Grant, 1999). Lower current speeds will increase feeding efficiency for small clams which often take advantage of crevices in the seafloor to escape predators.
The needs of ocean currents in scallops depend on their life stage and body size. The early life of A. irradians prefers habitats with low seagrass densities due to high ocean currents but adults have low survival in habitats with high ocean currents. Ocean currents are also related to the success rate of predators preying on shellfish and the like where the speed of ocean currents will affect the chemosensory predators to find clams and clams to detect and avoid predators. The success between predator and prey depends on who is most affected by the speed of the ocean currents.
Research on the habitat of filter-feeder organisms in the shallow waters of New Zealand shows that these organisms are not found in habitats with too large shallow ocean currents such as > 0.1 m/s because it makes it difficult for them to settle on the seabed. Ocean currents that are too strong will also encourage sediment resuspension events, especially in sediments that have large enough or coarse particles (Ribó et al., 2021).
Filter feeder organisms such as scallops usually inhabit the subtidal zone, which is part of the littoral zone (shallow waters in coastal areas), which is always inundated by water and does not experience sea tides (Dewi et al., 2020; Yulianda et al., 2020). Scallops are one of the shells that can swim well over long distances. Its habitat can be found in rather shallow waters, namely 10–40 m in North Australia, the Philippines, and Brebes, Indonesia (Aguirre, 2017; Sahri et al., 2014; Taufani et al., 2016). Research shows that scallop shells are more commonly found at depths of 21-30 m as seen from the highest catches in Tegal waters (Ernawati et al., 2017). Increasing the depth of the mussel habitat is related to the availability of food such as phytoplankton where the deeper the more limited the number is so scallop shells prefer shallow waters (Taufani et al., 2016). Meanwhile, in other studies, it was said that scallops do not like habitats that are too deep related to seabed temperatures where the deeper the seabed the lower the temperature (Sahri et al., 2014). Research on filter-feeder organism in New Zealand showed that the depth of the seabed is a limiting factor in the distribution of its habitat in addition to other factors such as the slope of the seabed and the type of sedimentary substrate. The maximum depth where scallops are still found in New Zealand’s shallow waters is 32 m on soft sediment substrate types (Ribó et al., 2021).
Research states that as filter feeders, bivalves are also selective in choosing phytoplankton as food based on type, size, shape, mobility, viscosity, toxicity, and nutrient composition. The feeding mechanism of shellfish is quite complex, such as the retention and preference of phytoplankton depending on the region, season and environment (Li et al., 2022; Pales Espinosa et al., 2016; Rosa et al., 2017). The physico-chemical characteristics of the surface of microalgae are useful for mussels in distinguishing between microalgae species because their characteristics are unique to each species. The physico-chemical of microalgae surfaces properties that are often used as differentiators are the degree of wettability and negative zeta potential which will determine the fate of the microalgae whether it can be ingested or rejected by the shells. Microalgae particles that tend to have organic surface chemical properties with many hydrophobic groups are preferred by mussels and oysters (Rosa et al., 2017).
Scallop shells have relatively shallow depths (18–31 m) so that in this euphotic zone, sufficient solar intensity is obtained for primary producers such as phytoplankton and other aquatic plants to carry out photosynthesis. The abundance of plankton that is often found in scallop habitat includes plankton from class Bacillariophyceae (Diatoms) like genus Chaetoceros, Nitzschia, and Skeletonema. A study of scallop habitat in Brebes waters, Central Java showed that scallop prefers a water column that has a fairly high plankton density of 11,001–14,500 ind/L. The density of plankton in the waters is influenced by the availability of sufficient water nutrients to support plankton growth (Sahri et al., 2014).
Scallop shells have a habitat on the seabed or sediments where denitrification occurs, namely a chemical process in water that converts dissolved nitrogen into nitrogen gas that can be used for plant growth. Nitrogen has an important function in aquatic ecosystems besides being a limiting factor as well as a driving factor for eutrophication. This denitrification process is assisted by organisms that live in sediments such as mollusc, tube worms, to marine microorganisms. These organisms play a role in the process of breaking down and transforming organic matter and releasing associated nutrients, facilitating their recycling, dispersal, and transfer through food webs (Thrush et al., 2017).
The concentration of nitrates (NO3) had an average range of 0.2766–0.3756 µg-atoms/L while nitrites (NO2) had an average of 0.0370–0.0417 µg-atoms/L. For the phosphate analysis, the concentration was not detected using the UV spectrophotometer (Cabacaba et al., 2018).
Ammonia is part of the water nutrients in the pores of marine sediments which are toxic, especially in non-ionized form (NH3). Ammonia comes from the degradation process of organic nitrogen in microalgae, microbenthos, and other aquatic organisms and then plays a role in the nitrification and denitrification processes by microbes through the reduction reaction of nitrite and nitrate into nitrogen gas. Meanwhile, in anoxic conditions (without oxygen) the Nitrogen transformation reaction will stop until the formation of ammonia which has high solubility in (Batley & Simpson, 2009). Exposure to high concentrations of NH3 can have a negative impact on the survival of bivalves. Research on Austrovenus exposed to NH3 under hypoxic conditions (low oxygen solubility) of 0.040 mg/L for 45 days gave sublethal and chronic lethal effects in the form of decreased regulation of NH3 production and increased NH3 excretion thereby reducing survival (Salmond & Wing, 2022). Exposure to un-ionized NH3 of 0.056 mg/L on Chlamys asperrima scallop larvae harmed larval development (Adams et al., 2008).
As filter feeders, scallops are generally susceptible to contamination in their natural environment, which can result in diseases not only for the scallops themselves but also for people who consume contaminated scallops. Asian moon scallops live on the seabed, where various contaminants tend to sink and form sediments. Although they can move from one area to another by propelling themselves, this swimming mechanism requires a significant amount of energy, making it challenging for them to avoid contaminated water. Diseases and treatment of scallops are shown in Table 3.
Species | Disease | Treatment | Ref. |
---|---|---|---|
Patinopecten yessoensis | Vibrio splendidus | Fed supplementary diets | Liu et al. (2013); Mi et al. (2022) |
Vibrio anguillarum | Immunoprotection of cinnamaldehyde | Shan et al. (2021) | |
Polydora | No treatment | Zhang et al. (2020) | |
Argopecten purpuratus | Vibrio bivalvicida | Bacterial probiotic addition | Muñoz-Cerro et al. (2024) |
Vibrio splendidus | No treatment | González et al. (2017) | |
Vibrio chagasii | No treatment | Urtubia et al. (2023) | |
Chlamys nobilis | Black shell Polydora haswelli & Polydora lingshuiensis Vibrio parahaeomlyticus | No treatment Stocking density control |
Zhang et al. (2024)
Li et al. (2020) |
Argopecten irradians | Paralytic shellfish toxin | Detoxifying agents (SMC) | Guo et al. (2023) |
Apicomplexa | No treatment | Pales Espinosa et al. (2023) | |
Patinopecten yessoensis | Vibrio anguillarum; Micrococcus luteus | No treatment | Zou et al. (2015) |
Placopecten magellanicus | Polydora concharum | No treatment | Sato-Okoshi & Okoshi (1993) |
Argopecten ventricosus | Vibrio alginolyticus | Antibiotics treatment | Mazón-Suástegui et al. (2017) |
Pecten maximus | Polydora hoplura | No treatment | Almón et al. (2022) |
Apicomplexan parasites | No treatment | Soares et al. (2021) | |
Vibrio coralliilyticus | Probiotic treatment | Kesarcodi-Watson et al. (2012) | |
Chlamys farreri | Acute viral necrosis virus | No treatment | Chen et al. (2011) |
Specific diseases for A. pleuronectes have not been extensively studied yet. However, there are several well-known factors that can lead to health issues in scallops (family Pectinidae) in general, with the primary culprits being marine biotoxins from bacteria, viral infections, and parasitic infestations. These major threats can lead to various effects, including immunological, physiological, and bioenergetic responses, such as altered growth and reproduction (Getchell et al., 2016). When the concentration of these health-altering organisms exceeds their natural threshold, it can result in mass mortality.
Among various classes of bacteria, Gram-negative Vibrionaceae and Rickettsiales are recognized as major culprits when it comes to bacterial infections in scallops (Getchell et al., 2016). Interestingly, these types of organisms tend not to be particularly harmful to adult scallops, as their pathogenicity appears to decrease as the scallops mature (Lauckner, 1983). However, infections caused by these bacteria can pose a significant threat to scallop larvae, especially in hatchery conditions. For example, there have been reports of a significant decline in cultured larvae for several scallop species, such as Argopecten ventricosus and P. maximus. These declines were attributed to Vibrio infections triggered by high-density environments and sudden changes in water quality in the hatchery (Luna-Gonzalez et al., 2002). Culture of scallop at high density not only leads to higher mortality and slower growth, but also delays the expression of CnGal mRNA in the gill under bacterial challenges (Li et al., 2020). While lethal infections are rare in the adult stage, previous study reported a fatal disease characterized by lesions on the adductor muscle of adult yesso scallops (P. yessoensis) in northeastern China, caused by Vibrio splendidus (Liu et al., 2013).
The susceptibility of scallops to bacterial diseases can also occur in their natural environment. Previous study documented a major mortality event in sea scallops (Placopecten magellanicus) in Narragansett Bay, Rhode Island, caused by intracellular prokaryotic infections from the order Rickettsiales. Another instance of gill infection by Rickettsiales bacteria was reported in A. irradians and P. magellanicus along the northeastern coast of the United States (Leibovitz et al., 1984). However, there were no indications of mass mortality among adult scallops, and their larval and juvenile stages remained free of infection.
Another pathogenic bacterium that commonly infects scallops is Francisella halioticida, a gram-negative bacterium firstly reported in Japan that caused 84% mortality of the giant abalone (Haliotis gigantea) (Kamaishi et al., 2010). This bacterium was detected in the abscess lesions of Yeso scallops in Japan (Kawahara et al., 2018). This bacterium can cause high mortality and abscess lesions in cultured Yesso scallops, especially in periods with higher temperatures (Kawahara et al., 2018).
Viral infections have been documented worldwide in connection with significant mortality outbreaks among cultured scallops. In the northern coast of China, Acute Viral Necrosis Virus (AVNV) outbreaks have posed a recurring and severe threat to the zhikong scallop (Chlamys farreri) farming industry in the region, occurring almost every summer since the mid-1990s. These outbreaks have resulted in massive mortality rates, with up to 90% of 1- to 2-year-old scallops succumbing within 5–8 days of the virus’s first appearance (Ren et al., 2013; Song et al., 2001). This AVNV is known to induce necrotic lesions in vital organs such as the gills, kidney, mantle, and digestive gland, leading to loss of responsiveness to stimuli, excessive mucus production in the viscera, and a shrunken mantle (Wang et al., 2007). Experts have proposed that a range of factors, such as elevated summer temperatures, a decline in water quality, and excessively dense culture conditions, play a role in triggering these viral outbreaks (Tang et al., 2010).
More recent instances of mass mortality caused by viral infections have been reported in the southern part of Korea, resulting in the devastating loss of 90% of 5- to 10-day-old A. irradians larvae (Kim et al., 2019). Despite the absence of specific clinical symptoms such as ciliate adhesion and velum necrosis on the deceased larvae, previous study concluded that the primary cause of these catastrophic events in 2011 and 2017 was caused by Ostreid Herpesvirus-1 µVar (OsHV-1 µVar) (Kim et al., 2019). While this virus has been reported as pathogenic to larvae, spat, and juvenile stages, it does not pose a significant threat to adult bivalves. However, vertical infection from infected broodstock has been noted as a cause of mass mortality among cultured seed (Arzul et al., 2002).
Parasitic infestation poses a significant and widespread challenge in the scallop industry, leading to various detrimental effects such as tissue damage, increased mortality rates, and even potential setbacks for the entire scallop farming sector. Among the known parasites affecting bivalves, those from the Apicomplexa group within the Alveolata category are particularly noteworthy, as they have been identified in equatorial to subtropical regions like Europe and North America, causing severe diseases in marine mollusks (Archibald et al., 2017; Kristmundsson et al., 2011, 2015). Numerous scallop species, including Aequipecten opercularis, A. irradians, Chlamys islandica, P. maximus, and P. magellanicus, have exhibited symptoms such as quality degradation in the adductor muscle, renal tissue damage, and increased mortality as the intensity of parasitic infestation worsens (Kristmundsson et al., 2011, 2015; Pales Espinosa et al., 2023; Soares et al., 2021).
Another group of eukaryotic parasites, specifically the Ascetospora, which encompasses Marteiliida and Balanosporida, as well as Ciliates, poses a substantial threat to scallop culture. Marteilia, for instance, was responsible for mass mortality in the calico scallop Argopecten gibbus during the late 1980s to early 1990s (Moyer et al., 1995). Meanwhile, Balanosporida has been implicated in major mortality events affecting bay scallops A. irradians and yesso scallops Haplosporidium nelsoni in China (Shumway & Parsons, 2016). On the other hand, many ciliates typically exist as harmless commensals, but they can transform into pathogens under specific conditions such as elevated population densities or environmental stressors. Such as those from the trichodinid group, which have been linked to tissue damage and mortalities in scallops of M.(P.) yessoensis and Chlamys sp. (Lauckner, 1983).
Furthermore, several other organisms, including platyhelminthes, polychaetes, crustaceans, gastropods, and algae, have been reported as potential parasites of scallops. These parasites can exert various impacts on scallops, ranging from damaging the host’s reproductive tissue and shell deterioration to causing gill and mantle damage, reducing viscera quality, inducing stunting, and even leading to mortality (Getchell et al., 2016).
Disease treatment in scallop aquaculture holds paramount importance in sustaining the health and productivity of this economically significant marine resource. However, like all aquaculture endeavors, scallop farming is susceptible to various diseases and infections that can jeopardize both individual scallop health and entire farming operations. Addressing these diseases is essential not only for safeguarding the well-being of the scallops themselves but also for ensuring a stable and reliable supply of this seafood delicacy to meet the demands of consumers worldwide. Moreover, responsible disease treatment practices in scallop aquaculture play a pivotal role in maintaining the ecological balance of marine ecosystems, minimizing the environmental impact of aquaculture operations, and upholding the sustainability of this vital industry.
Bacterial infection in cultured scallop, especially in larval stage, pose a serious threat to the sustainability of this business. Despite of offering a short-term solution for this problem, the use of antibiotics is not recommended for flow-through systems or as a chronic prophylactic measure due to the risk of antibiotic resistance (Getchell et al., 2016). Regulatory restrictions further limit their application, with some antibiotics like chloramphenicol already banned for use in organisms intended for human consumption (Torkildsen et al., 2002). Recommended solution to prevent this infection is by feeding supplementary diets, giving probiotic treatment, optimizing water quality and maintaining rigorous monitoring of hatchery facilities (Brown & Russo, 1979; Mi et al., 2022; Muñoz-Cerro et al., 2024). Understanding the sources of pathogens, which may include contaminated water sources and the transfer of bacteria from parents to larvae, is critical for adopting effective prophylactic measures (Sainz-Hernández & Maeda-Martínez, 2005). Promisingly, probiotic bacteria offer potential solutions for controlling bacterial proliferation in scallop larvae, which could reduce the reliance on costly and potentially environmentally hazardous chemotherapeutic agents in commercial scallop culture (Jorquera et al., 2001; Kesarcodi-Watson et al., 2012).
Conclusion
This review summarized recent knowledge on the scallop farming system and problem in several countries. Varieties of the farming system were explained from South Korea, China, Spain, Brazil, Norway, Chile, Canada, Norway, Australia and America. These varieties of farming system can be considered as a farming system for Asian moon scallops in Indonesia. Despite the recommended farming system, treatments to decrease the potential disease in Asian moon scallops farming were also explained. It is suggested that the potential of Asian moon scallop farming in Indonesia is extremely high, but it is necessary to expand research on technology and innovation of aquaculture for scallops such as Integrated Multi-Trophic Aquaculture.