Introduction
Somatic growth in fish broodstock candidate entering the puberty period is more directed towards the development of reproductive organs (testes or ovaries) which is stimulated by light, temperature and rainfall in the aquatic environment (Harvey & Hoar, 1979; Taranger et al., 2010). This external stimulation causes the secretion of reproductive hormones as endogenous (internal) stimulants that regulate (at least in part) the seasonal reproduction of fish (Mañanós et al., 2008). The effect of temperature on fish reproductive activity can increase or decrease gonad weight. Water temperatures that are too low may cause fish reproductive activity to slow down, while high temperatures cause low fish spawning, as a consequence of inhibiting the spermatogenesis process (Peñaranda et al., 2016; Sundararaj, 1981).
Endogenous factors that are influenced by environmental temperature and are involved in sperm density and Spz development are related to growth hormone (GH), steroid hormones (testosterone, T) which induce sperm cell proliferation and spermatocyte maturation (Baroiller et al., 2014). This indication shows that the regulation of GH expression (including CgGH insertions in transgenic catfish) cannot be separated from the influence of environmental temperature. Optimum temperature can increase GH expression levels and has implications for the induction of T levels and Spz density. Previous research results on transgenic G5 mutiara catfish formed through a broodstock breeding program (Buwono et al., 2021, 2023, 2024) showed the potential for broodstock gonad growth after induction of stocking density and light. However, not much is known about the role of temperature in the gonad maturation of male G5 transgenic mutiara catfish, which involves CgGH expression which leads to increased T levels and sperm density. The optimum level of temperature that drives CgGH expression, T levels and Spz density in G5 transgenic mutiara catfish broodstock needs to be evaluated to increase the number of gonad mature male fish broodstock as brood stock ready to be spawned in mass production of superior catfish fingerlings.
Materials and Methods
The G5 male catfish used came from previous research (results from the spawning of the parent pair of G4 transgenic mutiara catfish) with transmission of CgGH inserts in G5 of 89% (Buwono et al., 2024). Furthermore, 18 transgenic fish broodstock candidate and 18 nontransgenic fish broodstock candidate were used in the temperature difference treatment for 60 days. Temperature treatment applied to transgenic catfish (A: 24°C, B: 28°C, C: 32°C) and nontransgenic catfish (A*: 24°C, B*: 28°C, C*: 32°C) with 6 repetitions. Each broodstock rearing tank contains 6 G5 male fish (as replicates) for both transgenic and non-transgenic fish rearing tanks which are equipped with a water heater to regulate the treatment temperature. The volume of water filled in the round cylinder tank for temperature treatment is 700 L and the male fish are 5 months old with an average initial weight of 400–500 g for transgenic fish and 350–400 g for nontransgenic fish. During 60 days of maintenance, the test fish were given commercial food hiprovite-781 (33% protein), 2 times a day (3% biomass weight) with 50% water changes every week. Identification of G5 transgenic fish was carried out before treatment with a polymerase chain reaction (PCR) test and using primers GH-F (5’-ATGGCTCGAGTTTTGGTGCTGCT-3’) and GH-R (5’-CTACAGAGTGCAGTTGGAATCCAGGG-3’) (Zhang et al., 2009).
Isolated RNA for amplification of CgGH (600 bp) using fish tail fins (around 10–25 mg), and then processed using a Quick RNA Miniprep Plus Kit (Zymo Research, Irvine, CA, USA). Several studies have shown that transgene inserts (including CgGH) can be found along the head to tail area in transgenic rainbow trout, Oncorhynchus mykiss (containing sPRL-lacZ) and coho salmon, Oncorhynchus kisutch transgenic (insert OnMTGH1) (Uh et al., 2006; Uzbekova et al., 2003) and is stably inherited in offspring. RT-PCR CgGH was carried out using My Taq OneStep RT-PCR (Bioline, London, UK) with the program: 48°C (20 min) ; 40 cycles of 95°C (1 min), 95°C denaturation (10 sec), 60°C (30 sec), and 72°C (30 sec); and 72°C (5 min).
The role of temperature during maturation of male broodstock for seed production in spawning activities can be represented by the density of sperm numbers rearing broodstock at the appropriate temperature. Induction of optimum temperature leads to an increase in GH which works together with steroid hormones (T) which is involved in sperm cell proliferation (Schulz et al., 2012). The effect of temperature on transgenic fish can be represented by measuring sperm density and measuring T levels in the blood serum of test fish.
Counting the number of Spz in G5 fish (transgenic and nontransgenic) used a Neubaurer type haemacytometer (GrayMed, Victoria, Australia). A total of 1 μL of fish semen was diluted with 200 μL of 0.9% NaCl solution and then transferred with a micropipette into the haemacytometer counting chamber under a microscope. The multiplication factor for calculating Spz density is 32,000 which is obtained from 16 (the number of small boxes in the haemacytometer counting chamber) × 10 (0.1 mm haemacytometer slit thickness) × 200 (dilution with 0.9% NaCl) (Buwono et al., 2019). Blood sampling was carried out after the fish were anesthetized with 2-phenoxyethanol, and approximately 1.0 mL of blood was taken. from the tail artery using a 2 mL heparinized syringe. Blood samples were centrifuged at 664×g at room temperature for 20 min. Serum was stored at –20°C until hormone levels were analyzed. T levels in blood serum were measured using the ELISA method (Fish Estradiol ELISA kit, Bioassay Technology Laboratory, Shanghai, China), following the manufacturer’s protocol.
CgGH gene expression in G5 fish (transgenic and non-transgenic) after temperature treatment was carried out to analyze the effect of temperature on GH-transgenesis, density and histological development of fish Spz. RNA samples taken from brain tissue from two male G5 fish were taken and pooled for replicates of each transgenic fish treatment and 6 brain tissue samples were pooled for each nontransgenic fish treatment. Total RNA isolation using Quick-RNA miniprep plus kit (Zymo Research). The ReverTra Ace qPCR RT Master Mix kit with gDNARemover (TOYOBO, Osaka, Japan) was used for cDNA synthesis (as a template in rt-qPCR). CgGH gene expression analysis used an Agilent AriaMX Real-time PCR machine (Agilent Technologies, Santa Clara, CA, USA) with the reaction formula: 2× SensiFAST SYBR® NO-ROX (Bioline), with a final concentration of 100 ng/μL cDNA; and primers qCgGH-F and qCgGH-R to copy the CgGH fragment (Table 1). As an internal control for expression, primers CgβAct-Fw and CgβAct-Rv were used to copy the Clarias gariepinus β-actin gene and to normalize expression levels (Raghuveer & Senthilkumaran, 2010). The rt-qPCR reaction mixture and thermal profiles were prepared by quantification using 3 μL of cDNA sample in a final reaction volume of 20 μL containing 10 μL 2× SensiFast SYBR Lo-ROX Mix, 400 nmol of each primer, and 5.4 μL ddH2O. Real-time PCR program settings for reaction mixture: 95°C (120 s), 40 cycles of amplification phase (5 s at 95°C, 30 s at 56°C, and 20 s at 72°C), and melting program (30 seconds at 95°C, 30 seconds at 60°C, and 30 seconds at 95°C). CgGH mRNA expression levels were analyzed using the 2−ΔΔCT method after normalization with the C. gariepinus β-actin gene (Pfaffl, 2001; Schmittgen & Livak, 2001).
Primers | Sequence (5' → 3') | Amplicons (bp) | References |
---|---|---|---|
qCgGH-F | GTCTGATCGAGTCATGGGAGTT | 200 | Designed from genebank acc no MN249238.1 using Primer3 |
qCgGH-R | CTCAGGGTCTGGTAGAAA TCCTC | ||
CgβAct-Fw | ACCGGAGTCCATCACAATACCAGT | 200 | Raghuveer & Senthilkumaran (2010) |
CgβAct-Rv | GAGCTGCGTGTTGCCCCTGAC |
The number of G5 fish testes for Spz histology of transgenic and nontransgenic fish was six samples (3 samples of transgenic fish and 3 samples of nontransgenic) representing each temperature treatment of 24°C, 28°C, and 32°C. After taking the test fish’s testicles, the sample was sliced 3–5 mm thick using a microtome with an area of 1 cm3 and soaked in Bouin’s solution. Furthermore, serial washing with alcohol was carried out with concentrations of 70%, 80%, 90%, 96% and absolute alcohol. The next stage was clearing work using Toluol solution and continued with the infiltration process in an oven (55°C) and making paraffin blocks using paraffinum solidum. The paraffin block is then cut thinly and attached to a glass object and dripped with Mayer’s albumin until a thin film is formed. The final stage is staining by dipping the glass object containing the specimen into Xylol I, II, III solutions for 5 min in a row or using hematoxylin-eosin. Histological photo documentation of G5 fish sperm using a Trinocular CX31 Microscope (Olympus, Tokyo, Japan).
Results
Identification of transgenic G5 catfish (containing CgGH) using PCR and primers GH-F and GH-R resulted in 18 potential transgenic male catfish parents (Fig. 1).

The PCR test showed that the CgGH gene insert was contained in the transgenic fish (Fig. 1) and the resulting amplicon was 600 bp in size, indicating that the GH-F and GH-R primers could copy CgGH in G5 fish samples. Previous research results on transgenic mutiara catfish G1, G2, G3, G4 showed that the size of the GH amplicon in four generations was 600 bp (Buwono et al., 2021, 2023, 2024). Consistency in the size of the CgGH insert was also shown in male G5 transgenic mutiara catfish of 600 bp (Fig. 1).
Low temperature (24°C) caused an increase in serum T levels in G5 transgenic mutiara catfish which was significantly higher than the temperature of 28°C and 32°C among transgenic fish, whereas the temperature of 28°C in nontransgenic fish was higher than the temperature of 24°C and 32°C (Fig. 2A). Significant differences in T levels occurred at temperatures of 24°C and 32°C and temperatures of 28°C and 32°C in nontransgenic fish, and were consistently followed by Spz densities in nontransgenic G5 catfish that were significantly different between temperatures of 24°C and 32°C and at temperatures of 28°C and 32°C (Fig. 2B). There was no difference in Spz density in nontransgenic fish between temperatures of 28°C and 32°C. Meanwhile, among G5 transgenic catfish, the density of Spz at 24°C was higher than at 28°C and 32°C and in the three temperature treatments there was a significant difference in Spz density and was consistent with the results of the measured T levels (Fig. 2A and 2B).
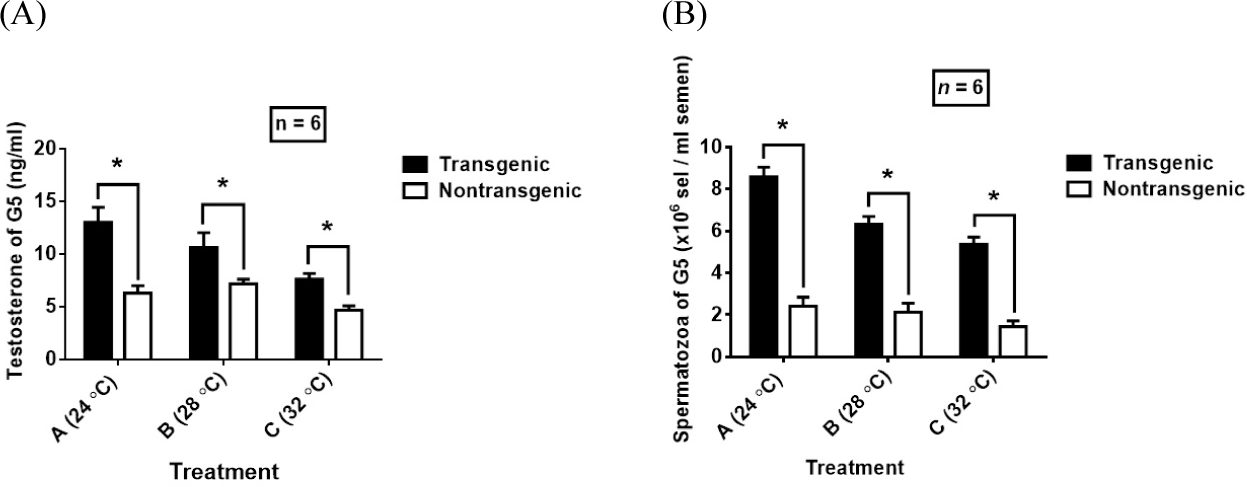
High temperature (32°C) caused an inhibitory effect on CgGH expression which led to a decrease in the number of Spz and the spermatogenesis process in G5 fish (Fig. 3A and 3B). In contrast, the optimum temperature (24°C) caused a higher increase in CgGH expression levels than 28°C and 32°C among G5 transgenic catfish (Fig. 3A and 3C) and was consistent with the T levels and Spz density produced (Fig. 2A and 2B). This indication shows that both the increase in GH expression, T levels and Spz density in transgenic and non-transgenic G5 catfish were influenced by the temperature of 24°C.
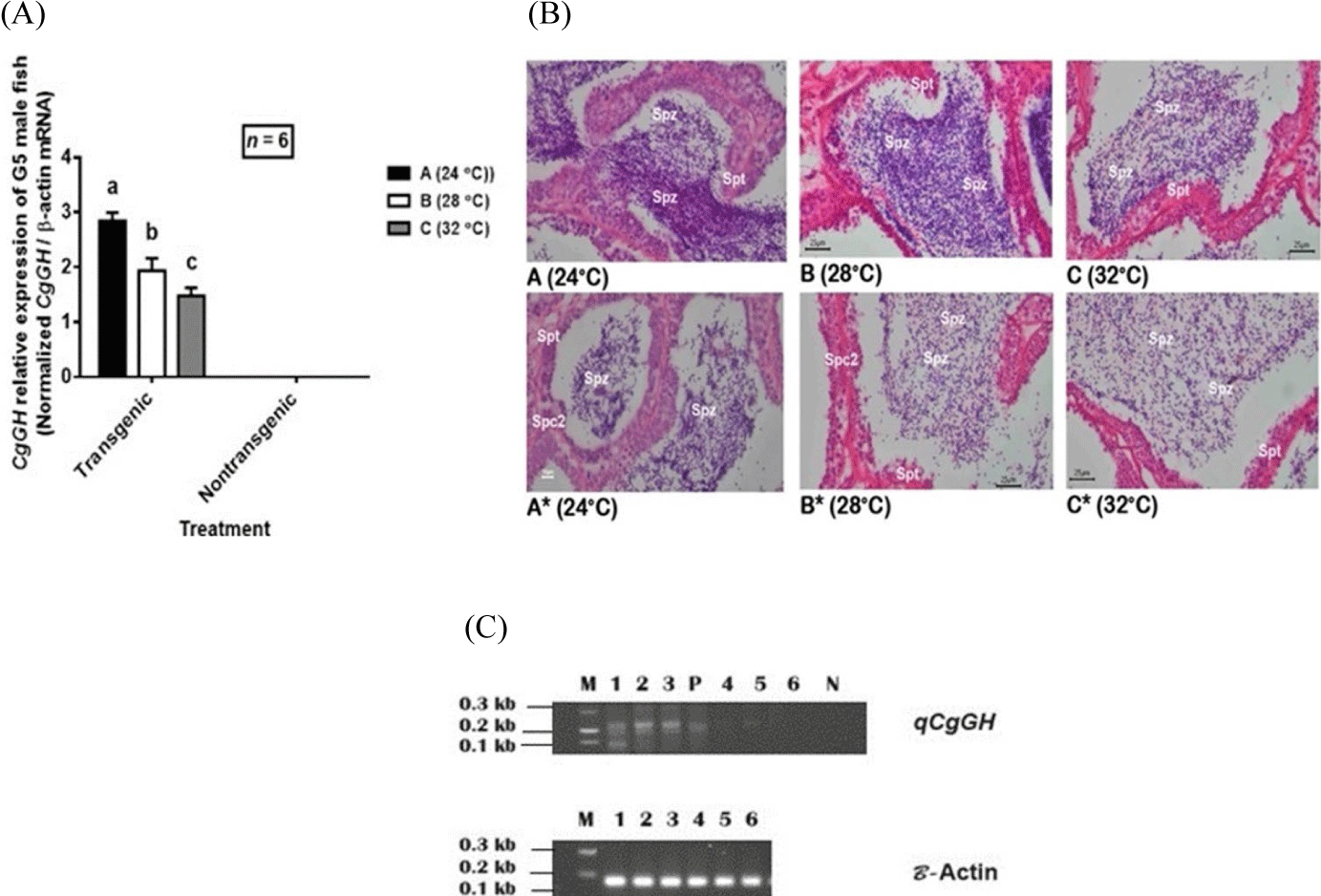
In line with the expression of GH (including CgGH), there was an indication that relatively low temperature (24°C) increased the number of Spz (Fig. 3B) compared to high temperature (32°C) and 28°C. These results indicate that high temperature causes suppression of CgGH expression levels and leads to a decrease in the number of Spz (Fig. 3A and 3B).
Discussion
Identification of G5 transgenic mutiara catfish male broodstock using PCR testing showed 18 transgenic male fish (containing CgGH, 600 bp) and 18 nontransgenic fish (negative CgGH) to be used as test fish in the temperature difference treatment. Previous research results showed that CgGH transmission in G5 transgenic catfish had reached 89% (Buwono et al., 2024), indicating stable transgene inheritance in each generation. Stability of transgene inheritance is required for the production of transgenic fish offspring, as has been observed in mud loach (Misgurnus mizolepis) transgenic fish (carrying CMV-H2B-GFP) to produce 50% homozygous G3 progeny (Nam et al., 2000). The presence of the CgGH gene in G5 transgenic male catfish broodstock needs to be evaluated to test the effect of temperature differences on GH-transgenesis. Apart from that, it is necessary to compare nontransgenic catfish in testing T levels and sperm density to determine the optimum temperature that increases CgGH expression and leads to an increase in T levels and sperm density in G5 fish. The results of research on male transgenic G1 mutiara catfish at a rearing temperature of 26°C indicated that the T levels and sperm density of transgenic G1 catfish increased 1.78 times (11.25/6.32 ng/mL) with a sperm density of 1.57 times (8.60 / 5.46 × 106 cells/mL semen) from nontransgenic fish (Buwono et al., 2019). This indication shows that GH-transgenesis has the potential to increase T levels and fish sperm density when induced by optimum temperature.
The addition of foreign gene inserts (CgGH) has biological benefits related to increasing T levels and sperm count, leading to improvements in the quantity of sperm production in male fish and has implications for improving the reproductive quality of male fish. The results of research on catfish, Clarias batrachus, show that the GH receptor (GHR) found in Leydig cells plays a role in binding GH which is important in regulating steroidogenesis. GH stimulates the growth factor IGF-1 (insulin like growth factor-1) of Leydig cells. Next, Leydig cells are activated to secrete T which maintains the spermatogenesis process of spermatocyte cells until Spz are formed (nee Pathak et al., 2015). This explanation shows that GH (including CgGH) has a positive effect on improving the reproductive performance of male fish and the effect of GH-transgenesis in transgenic fish is more beneficial than in non-transgenic fish. Transgenesis makes it possible to introduce beneficial traits or phenotypes that cannot be realized by conventional selective breeding (Hallerman et al., 2007).
Among exogenous cues, water temperature has a major influence on gametogenesis of fish, especially tropical fish species and has implications for the spawning of brood fish during the reproductive season. In addition, research has shown that higher water temperatures cause inhibition of spermatogenesis, considering that the regulation of spermatogenesis stages is controlled by steroids (T), the production of which depends on the activity of enzymes involved in steroid production and whose activity is influenced by temperature. Maximum T production is obtained when the temperature is in the range of 22°C–30°C in Sarotherodon mossambicus Tilapia fish (Kime & Hyder, 1983). Like the results shown in Tilapia fish, in transgenic G5 mutiara catfish, temperature treatment determined the T levels produced over 60 days (Fig. 2A), indicating that a temperature of 24°C increased T levels higher than temperatures of 28°C and 30°C. in transgenic fish and nontransgenic fish. The optimum temperature (24°C) has a higher effect on T levels in G5 transgenic catfish than other treatments, related to the temperature range that induces enzyme activity involved in converting the precursor 17,20β-dihydroxypregn-4-en-3-one into T such as which was studied in male fish (Scott et al., 2010). On the other hand, high temperatures (32°C) had the effect of reducing T levels, both shown in transgenic and nontransgenic G5 mutiara catfish. These results confirms that the effect of temperature which produces maximum T levels in Tilapia fish was in the range of 22°C–30°C, while temperatures above 30°C reduce T levels as shown in test fish. Besides that, it can be shown that the use of GH-transgenesis in G5 transgenic catfish provides higher average T levels compared to nontransgenic fish in all temperature treatments. This indication explains that exogenous GH (insertion CgGH) plays a role in increasing Leydig cell activity in T production, where the effect is enhanced higher at 24°C than other temperature treatments. This indication has been shown in research on the fish C. batrachus, that GH is a mediator for Leydig cells for T production (nee Pathak et al., 2015). Previous studies in tilapia showed that increasing GH levels increased Leydig cell activity for T production (Tokalov & Gutzeit, 2005; Yamaguchi et al., 2006).
A decrease in T levels of 6 month old African catfish (C. gariepinus) to 0.73 ng/mL was found at a temperature of 34°C (Okoye et al., 2018). Confirmation of these results was also shown by the effect of high temperature treatment (32°C) causing a decrease in T levels in both transgenic G5 catfish (average 7.60 ng/mL) and nontransgenic (average 4.66 ng/mL), indicating a negative effect of temperature. high levels reduced the T levels of test fish (Fig. 2A). As explained previously, it shows that androgen (T) production is regulated by enzymes involved in T production (11-ketotestosterone, 11 KT and DHP, 17α,20β-dihydroxy-4-pregnen-3-one) and maximum work activity. depending on the optimum temperature (Kime & Hyder, 1983; Quirino et al., 2021; Schulz et al., 2010). The results showed that the optimum temperature of 24°C increased the T levels of G5 transgenic mutiara catfish (average 12.99 ng/mL) higher than other temperature treatments between transgenic and nontransgenic fish, but in non-transgenic fish, the temperature of 28°C increased the T levels. (average 7.17 ng/mL) was higher than the temperature treatment among nontransgenic fish. This difference is likely due to the activity of enzymes involved in the production of maximum T levels in nontransgenic fish found at a temperature of 28°C.
The density of Spz produced by male fish determines the number of larvae produced, because the number of sperm produced contributes to the success of fish spawning (Miura et al., 2011). The process of Spz formation cannot be separated from the induction of the steroid T which maintains spermatogenesis. The correlation between T levels and Spz density could indicate that steroid hormones play a role in spermatocyte cell proliferation and this correlation is influenced by water temperature during the maturation of male broodstock (Miura et al., 2011; Yamaguchi et al., 2006). The results of research on transgenic G5 mutiara catfish showed that a temperature of 24°C increased Spz density higher than temperatures of 28°C and 32°C between transgenic fish and nontransgenic fish (Fig. 2B). These results indicate that the optimum temperature affects transgenesis-GH in G5 transgenic male parents induces an increase in T levels, and GH works together with the steroid hormone T to activate testicular tissue to stimulate the production of male gametes (sperm) which are needed for fish spawning activities (Maestro et al., 1997; Miura et al., 2011). A temperature of 24°C increased the number of Spz in G5 transgenic catfish higher (an average of 8.64 × 106 cells/mL semen) than other treatments, which is the optimum temperature that maximizes the effect of exogenous GH (CgGH) to stimulate sperm cell proliferation as compensation. Spz density of G5 transgenic fish (Fig. 2A and 2B). In contrast, in nontransgenic catfish, at both temperatures of 24°C and 28°C, the Spz density was lower (2.42 × 106 cells/mL semen and 2.13 × 106 cells/mL semen) than in transgenic catfish, indicating that the presence of CgGH inserts in the fish increased the density. Spz. This temperature-influenced GH activity effect in sperm production and T steroids was similarly demonstrated in studies of Fundulus heteroclitus fish and zebrafish (Danio rerio) (Negatu et al., 1998; Nóbrega et al., 2015), explaining the role of temperature in T production and male fish sperm production.
Over-expression of GH in male transgenic G1 mutiara catfish mediated by IGF-1 causes an increase in the number of Spz due to the influence of exogenous GH (CgGH) inducing sperm cell proliferation (Buwono et al., 2019). The GHR located in Leydig cells suggests a potential function of GH in the gonads of male fish (Yamaguchi et al., 2006). The interaction of GH with T steroids was also demonstrated in the testes of catfish, C. batrachus increasing T levels produced by Leydig cells. The steroidogenic action of GH on testicular tissue causes an increase in sperm cell proliferation (nee Pathak et al., 2015). CgGH expression levels in G5 transgenic catfish showed that high expression was found at 24°C treatment among transgenic fish, consistent with the temperature effect shown by T levels and Spz density of transgenic fish (Fig. 2A and 2B). This confirmation is closely related to the optimum enzyme activity at 24°C, as studied in male tilapia (Baroiller et al., 2014), increasing T production and Spz density. Previous research on G1 transgenic male mutiara catfish at a temperature of 26°C (a relatively close temperature), showed that increasing CgGH expression caused G1 transgenic T levels to be higher than in G1 nontransgenic fish (Buwono et al., 2019). Exogenous GH expression in male transgenic catfish caused CgGH expression levels in the testes of transgenic fish to be higher than in non-transgenic fish and T production in transgenic males was higher than in non-transgenic fish. This consistency was shown from the results of research on G5 transgenic catfish, that the expression level of CgGH at 24°C (average 2.85 times higher) than at temperatures 28°C (average 1.93 times) and 32°C (average 1.48 times) than nontransgenic fish (Fig. 3A), explaining that GH-transgenesis in transgenic fish is more beneficial for increasing the number of Spz and T levels compared to nontransgenic fish. These results confirms previous studies that optimum temperature regulation induces an increase in exogenous GH levels which leads to increased GHR expression in tilapia Leydig cells and T production also increases (Tokalov & Gutzeit, 2005; Yamaguchi et al., 2006).
The microanatomy of G5 fish Spz shown in Fig. 3B explains that in all temperature treatments in G5 transgenic catfish, the number of Spz formed was higher than in nontransgenic fish, indicated by the density of the number of Spz that filled the lumen of the seminiferous tubules. The multiplication of Spz shown by the histology results of testicular tissue of test fish at 24°C (Fig. 3B) was higher than at other temperature treatments and nontransgenic fish, consistent with the results of Spz density measurements (Fig. 2B). Likewise, the number of Spz at 28°C in transgenic fish was greater than at 32°C and nontransgenic fish. Research on catfish, Lophiosilurus alexandri, shows that a temperature of 23°C increases the number of sperm cells compared to a temperature of 27°C with the proportion of Spz dominating the seminiferous tubules (Melo et al., 2022). The sperm development stage in the test fish moreover showed an abundance of Spz at a temperature of 24°C, followed by temperatures of 28°C and 32°C. The density of Spz of transgenic fish was higher than that of nontransgenic fish as shown by the abundance of Spz in Fig. 3B, indicating that high temperature (32°C) reduced T levels and as a consequence suppressed the number of spermatocytes that developed into Spz. Histologically, it was shown that in nontransgenic fish (not containing CgGH), the development of secondary spermatocytes was still found at 24°C and 28°C treatments, indicating that the effect of GH-transgenesis in transgenic fish stimulated faster spermatocyte development in both treatments. temperature compared to nontransgenic fish (Fig. 3B). The 24°C temperature treatment had a positive impact on the development of spermatocytes into Spz in G5 transgenic fish in abundance, as indicated by the dominance of Spz development which filled the entire lumen space of the seminoferous tubules. These results, indicate that GH-transgenesis increases the number of Spz and increases the formation of Spz.
Increasing the temperature above 30°C causes inhibition of androgen formation in the biosynthesis of T which is shown in the testes of S. mossambicus (Kime & Hyder, 1983). In line with the effect of a temperature of 32°C on the testes of G5 transgenic mutiara catfish, it caused a decrease in T levels and as a result the number of Spz decreased (Fig. 2A and 2B). The results of the analysis of calculating the proliferation of tilapia testicular cells (Oreochromis niloticus) at a temperature of 35°C, showed a decrease in the proliferation index of somatic testicular cells (both Leydig cells and Sertoli cells) leading to a decrease in sperm count compared to a temperature of 30°C. Based on this study, it can be explained that the decrease in sperm count treated at 32°C in this study is similar to the discussion at 35°C in O. niloticus (de Alvarenga & França, 2009).
The decrease in GH expression levels (including CgGH) in transgenic catfish at 32°C (Fig. 3A) followed by a decrease in T levels (Fig. 2A) indicated the inhibitory effect of GH at the pituitary level. Regulation of GH secretion by this T negative feedback mechanism was also demonstrated in trout testes when spermatogenesis had reached the final stage of spermatid formation (Le Gac et al., 1992). This confirmation shows that the high temperature (32°C) in this study caused an inhibitory effect on T secretion (T levels decreased) and T steroids provided negative feedback to the pituitary which suppressed CgGH expression and as an acceleration the number of Spz also decreased.