Introduction
Beche-de-mer or dried sea cucumber (Holothuria sp.) is a luxury seafood that dominates more than 90% of the sea cucumber trade in the world. Sea cucumbers have long been used as health food (ethnic tonic food) by people in Asia and the Middle East for thousands of years. According to data from UN Comtrade, the recorded export volume of sea cucumbers (Holothuria sp.) in fresh, frozen, and dried form from Indonesia in 2020 was 1,295,710 kg, with a total value of US$13,083,026. Three of the most valuable species among the 70 utilized species of sea cucumbers are Holothuria fuscogilva (white teatfish), Holothuria Lessoni (golden sandfish), and Holothuria scabra (sandfish). Various functional foods from sea cucumbers including instant sea cucumbers, sea cucumber capsules and sea cucumber caplets were reported to be on the market by Zhang et al. (2016). High amounts of protein (almost 90% of dry matter), vitamins, minerals, and a component called glycosaminoglycan (GAG) are contained in sea cucumbers.
Holothurian GAGs is a sulfated polysaccharide isolated from the body wall of sea cucumbers. It includes chondroitin sulfate and sulfate fucan (Siddiqui et al., 2022). GAG sulfate from sea cucumbers has a diverse and complex structure, as well as potential bioactivity as an antiviral (Song et al., 2020), antithrombotic (Liu et al., 2016), and anticoagulant (Chahed et al., 2020). GAG sulfate derived from sea cucumbers has the potential to treat thrombolytic disorders in COVID-19 patients (Dwivedi et al., 2021), as it can act as an anticoagulant agent with a reduced risk of bleeding and be used as an antithrombotic agent (Luo et al., 2013). According to Wu et al. (2012), the anticoagulant activity of sea cucumber sulfateted GAGs is 348 IU/mg. The anticoagulant potency of GAG sulfate is directly proportional to its sulfate content, which should reach at least 53% (Pomin, 2014). The anticoagulant effect increases as the sulfate content rises. For instance, GAG sulfate from the sea cucumber Isostichopus badionothus has a high sulfate content of approximately 95% and contains 2,4-di-sulfate and 3,4-di-sulfate units, which contribute to its potent anticoagulant effect (Chen et al., 2012).
Dried sea cucumbers are commonly soaked in water several times until they reach their normal size before being processed for food or medicine. Rehydration is a complex mass transfer process that involves various physical mechanisms such as water absorption, surface convection, and relaxation of the solid matrix (Mulet et al., 2003). A previous report suggests that the traditional rehydration method for dried sea cucumbers involves boiling the sample 2–3 times and then soaking it in cold water at 4°C for two days (Xiang et al., 2007). Zhang et al. (2016) reported that one of the main issues with traditional drug processing in China is inefficient rehydration, which can lead to changes in the quality of bioactive components. Long rehydration times can also cause damage to these components. Ultrasound-assisted rehydration (UAR) can improve this process by reducing internal resistance and influencing product microstructure (Ricce et al., 2016). This method has been successfully applied to various food products, including brown rice (Cui et al., 2010), beans, and sorghum (Ghafoor et al., 2014). The underlying mechanism involves cavitation waves that enhance mass transfer, accelerate diffusion, and minimize damage to active components (Wang et al., 2018). In fact, Santos et al. (2021) found that UAR can shorten the process time by 14% and reduce energy consumption by 19% in dry food processing.
Zhang et al. (2016) discovered that applying UAR with varying frequencies and powers can increase the rehydration capacity of sea cucumbers by up to 12-fold while promoting a faster swelling rate and a looser structure. Notably, there were no adverse effects observed on the textural properties of the product. Dhanapal et al. (2013) have also reported that ultrasound treatment can effectively inhibit enzymatic reactions and enhance the sensory quality of food products, leading to improved final product quality.
Despite these promising findings, there is still ample room to explore the effect of temperature as an additional factor for improving the rehydration process and quality of dried sea cucumber. Therefore, the main objective of this study was to investigate the effect of UAR at different temperatures on the quality of dried sea cucumbers (H. scabra). Specifically, the study aimed to evaluate the impact of ultrasound treatment on the rehydration behavior, moisture distribution, physicochemical properties, microstructure, and GAG properties of the rehydrated sea cucumber.
Materials and Methods
The raw material for this experiment was dried sea cucumbers (H. scabra) supplied by a local fisherman on Batu Karas Beach, Pangandaran, West Java, Indonesia. The materials were transported to the lab in plastic bags packed in an insulation can without water under a temperature of 15°C–20°C within 8 h. The sea cucumbers were all dried using the same process and all had the same size and shape under standard commercial conditions according to the National Standard of Indonesia. They had a weight of 35.57 ± 5.62 g, a length of 7.9 ± 0.53 cm, a moisture content of 13.76 ± 0.04%, a fat content of 0.80 ± 0.06%, a protein content of 65.68 ± 0.14%, and a sulfate content of 5.97 ± 0.31%. Various chemical materials were used in the study, including mineral water, distilled water, NaOH (40%), ethanol (70%), buffered neutral formalin (10%), and papain enzyme with a pH of 6 and an activity of 10,000 u/g. These chemicals were purchased from Chem Mix Pratama Industry (Yogyakarta, Indonesia) and were of analytical grade. Several instruments were used to conduct the experiments, including an ultrasonic water bath (KQ-500Z, Kunshan Shumei Ultrasonic Instrument, Jiangsu, China), a precision weight balance of 300 g with a sensitivity of 1 mg (AIK Instruments, Jiangsu, China), a colorimeter (Shenzhen Wave Optoelectronics Technology, Guangdong, China), a water activity meter (WA-60A, Guangzhou Landtek Instruments, Guangzhou, China), a scanning electron microscope (SEM) (Phenom ProX Desktop SEM, Thermo Fisher Scientific, Waltham, MA, USA), an energy meter (Model PCM-282 EU, Gain Express, Shenzhen, China), and Fourier transform infrared spectroscopy (FTIR) (Model SP-IR990, Wincom Company, Hunan, China).
The rehydration process of dried sea cucumber was fixed with a sample/water ratio (1:6 v/v) according to Xiang et al. (2007) and Zhang et al. (2016) with minor modifications. Rehydration profiling data were collected without ultrasound at 15°C for 48 hours, with weighing every 2 hours. The changes in the moisture content of the sample were assessed using a mass balance method. The sample was taken out from the water, drained, blotted with absorbent paper to remove surface water, weighed, and then returned into the water. A reference time range was established when a constant weight was achieved for two consecutive weighing between 0–48 hours, which was used for the main method of sea cucumber rehydration. To reduce error, all tests were performed in triplicate. The rehydration experiments were conducted at two different temperatures: 27°C and 15°C and the methods were performed under four different conditions:
-
a) KV27°C = conventional rehydration at 27°C
-
b) KV15°C = conventional rehydration at 15°C
-
c) UAR27 + KV15°C = a combination of ultrasound rehydration at 27°C and conventional rehydration at 15°C
-
d) UAR15 + KV15°C = a combination of ultrasound rehydration at 15°C and conventional rehydration at 15°C
To analyze the rehydration process, the water absorption capacity (Wa) was evaluated as a percentage increase in weight and was determined using Equation (1), which is based on the measurement of the weight difference of the sample before and after rehydration.
where, Wa is water absorption (dry basis (d.b.) %), Wf is weight of sea cucumber after rehydration (g) and Wi is weight of sea cucumber before rehydration (g).
The capacity of dried products to regain their moisture is typically denoted by the rehydration rate (RR), as defined by the equations proposed by Krokida & Marinos-Kouris (2003).
This rate expresses the extent to which the material can regain its moisture content, represented as X (expressed in g of moisture per g of dry sample) during rehydration. When X equals zero, the initial moisture content of the dry material (measured in g of moisture per g of dry sample) is denoted as Xi.
The characterization of rehydrated sea cucumbers comprises various parameters. These parameters include proximate composition, sulfate content (AOAC, 1998), color, energy measurement (Uju et al., 2018), and tissue microstructure observed by SEM (Zhang et al., 2016). Sulfated GAGs can be characterized by extracting sulfated GAG (Dong et al., 2014) and identifying functional groups using FTIR spectrophotometry.
The research was conducted using analysis of variance with four treatments of three replications each. The treatments comprised of differences in rehydration methods with four levels: conventional rehydration at 27°C and conventional rehydration at 15°C (KV27°C and KV15°C, respectively), combined ultrasound and conventional rehydration treatments (UAR27 + KV15°C and UAR15 + KV15°C, respectively). The data was analyzed using Statistical Product and Service Solutions (SPSS) IBM statistics product version 25.0.0 (Armonk, NY, USA) at a 95% confidence level (α = 0.05). The validity of the data was assessed by testing the assumptions that underlie the analysis of variance through normality tests and homogeneity tests. If the results obtained are significantly different (p < 0.05), then the Duncan multiple range test is performed.
Results
Assessing the rehydration process of sea cucumbers is critical for evaluating water penetration efficiency into their micropores after dehydration. This process can significantly impact the ultimate quality of the product. Thus, four conditions were used on dried sea cucumbers to investigate the effectiveness of various rehydration techniques, as illustrated in Fig. 1.
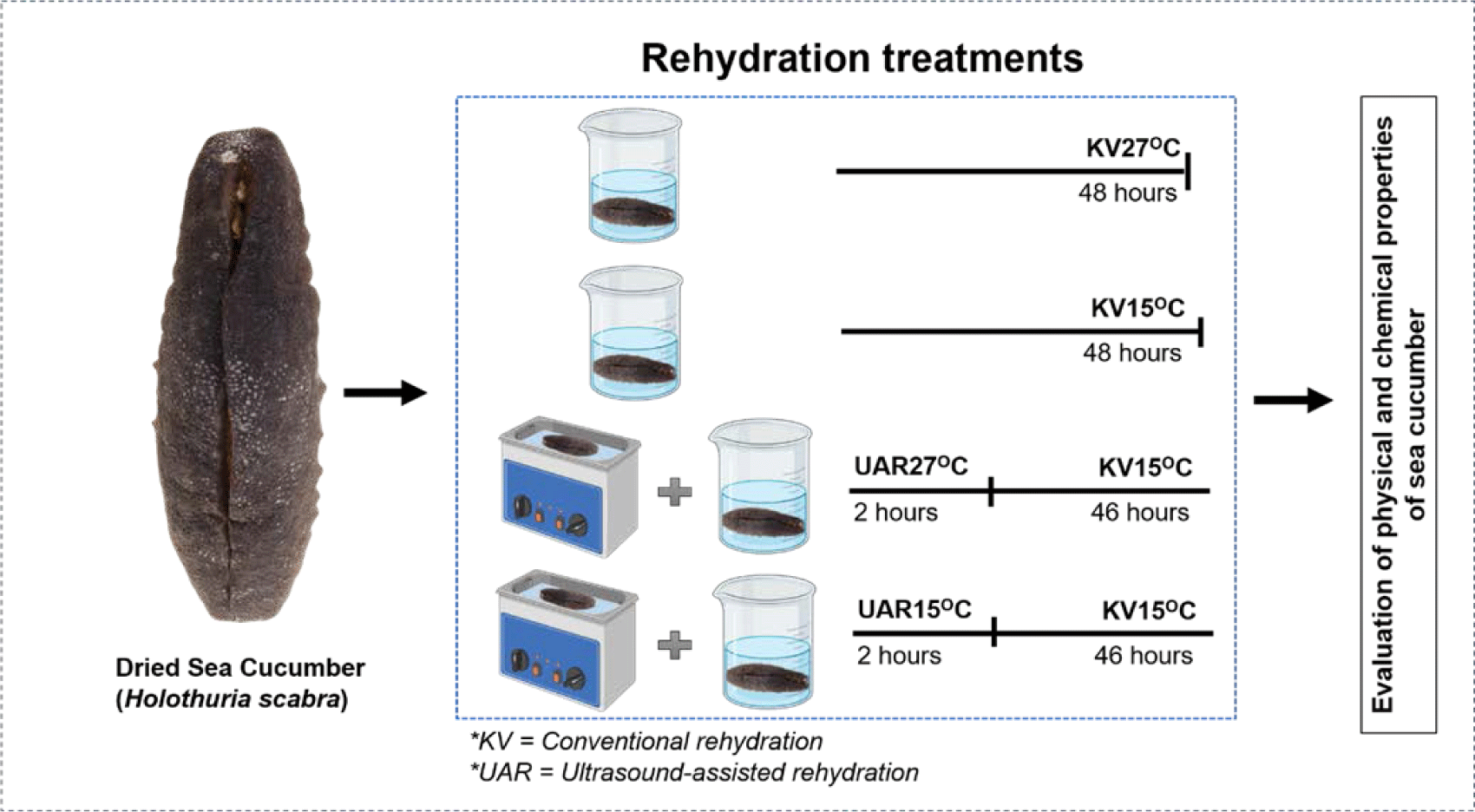
Fig. 2A depicts the water absorption properties of dried sea cucumber, a critical parameter in determining the optimal rehydration time and conditions to achieve preferred moisture levels in the rehydrated product. Prolonged rehydration periods significantly improve the water absorption capacity of dried sea cucumbers. The standard hydration level was 1.44, which was achieved after reaching the same moisture content in at least three measurements. The sea cucumber treated with UAR15 + KV15°C exhibited the longest water absorption time, reaching maximum hydration after 48 hours. In contrast, the UAR27 + KV15°C and KV15°C treatments achieved a similar water level after 28 and 32 hours of rehydration, respectively. Notably, the sea cucumber treated with KV27°C displayed the shortest water absorption time, lasting only 12 hours compared to the other treatments.
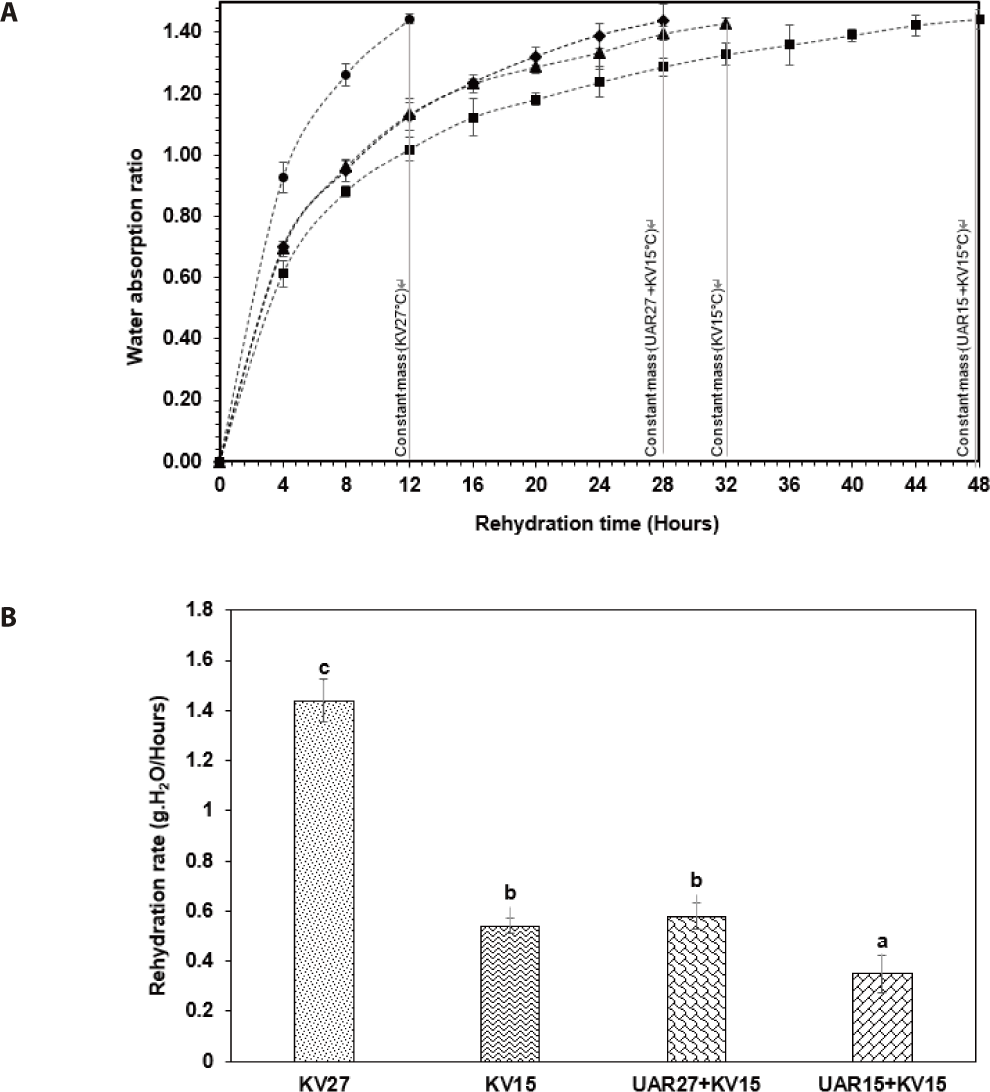
The RR refers to the amount of water absorbed by a product in a specific time and can be used to calculate the lost water per hour per mass of the product. Fig. 2B presents the RR for the sea cucumber, with the KV27°C treatment exhibiting the highest RR at 1.44 ± 0.85 gH2O/hour. In contrast, the UAR15 + KV15°C treatment showed the lowest RR at 0.35 ± 0.075 gH2O/hour. Statistical analyses revealed that the rehydration methods used had a significant impact on the RR of the sea cucumber (p < 0.05), particularly with the KV27°C and UAR15 + KV15°C treatments. However, there was no significant difference (p > 0.05) in the RRs of sea cucumber treated with UAR27 + KV15°C and KV15°C at 0.58 ± 0.53 gH2O/hour and 0.54 ± 0.03 gH2O/hour, respectively.
The quality characterization of rehydrated sea cucumber is conducted based on several parameters, including proximate composition, sulfate content, color, microstructure, sulfated GAGs, and energy measurement. The characterization of rehydrated sea cucumber quality is a critical step in identifying the optimal rehydration method and determining the quality of the resulting product. Rehydration times for the different treatments were determined based on the prior water absorption results. The KV27°C treatment was rehydrated for 12 hours, while the UAR15 + KV15°C treatment required 48 hours for rehydration. Likewise, the UAR27 + KV15°C and KV15°C treatments were rehydrated for 28 and 32 hours, respectively, to achieve the same final moisture content of ~67% (1.44 kg water/kg dry mass).
Table 1 presents the proximate composition of the rehydrated sea cucumber. The results show that they all have a significantly high protein content, ranging from 76.47 ± 1.06% to 82.18 ± 0.63% (p < 0.05). UAR27 + KV15°C has a higher protein content than the others, whereas KV27°C has the lowest. Furthermore, UAR27 + KV15°C has a higher carbohydrate content than the other treatments. On the other hand, the fat content is relatively low in all of them. However, there are no significant differences (p > 0.05) in the fat and carbohydrate contents between UAR and KV treatments.
Fig. 3 depicts the sulfate content of rehydrated sea cucumbers. The sulfate content in sea cucumber has attracted attention because it can affect the binding of polysaccharides and other bioactive compounds, which could lead to the development of new pharmaceuticals and nutraceuticals. The sulfate content of the UAR and KV treatments was significantly different (p < 0.05), ranging from 76.8 mg/g to 90.5 mg/g. The UAR27 + KV15°C treatment exhibited the highest sulfate content of 90.5 ± 0.48 mg/g, while the lowest sulfate content was observed in KV27°C, which was 76.8 ± 0.5 mg/g. Notably, all rehydrated sea cucumbers had a higher sulfate content than dried sea cucumbers.
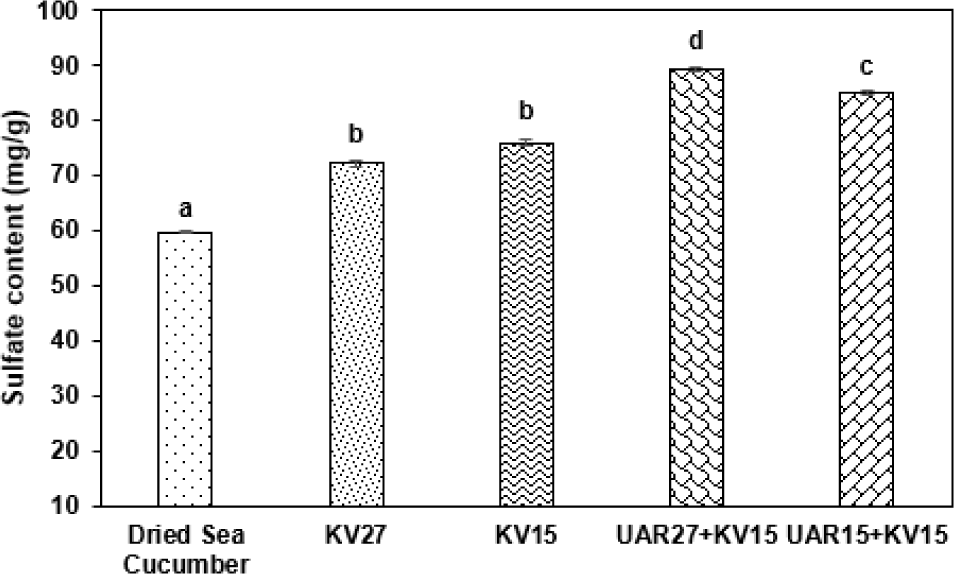
Table 2 displays the effect of different rehydration treatments on the color (L*, a*, b*, and total difference [ΔE]) of the rehydrated sea cucumber. L* indicates the gradient from darkness to brightness, with values ranging from 0 to 100. While a* symbolizes the spectrum from greenness to redness, with values ranging from –128 to +127, and b* represents the spectrum from blueness to yellowness, ranging from –128 to +127. The color of rehydrated food plays a critical role in determining its acceptability among consumers, serving as a direct indicator of the underlying biochemical and physiological changes occurring in the muscle tissue. As shown in Table 2, significant differences (p < 0.05) were observed in all color parameters between the UAR and KV treatment. The L* of sea cucumber treated with UAR was higher than that of sea cucumber treated with KV. The L* values, from lowest to highest, were KV27°C, KV15°C, UAR27 + KV15°C and UAR15 + KV15°C. The total color difference followed the same trend, with UAR15 + KV15°C exhibiting a higher total color difference than all sea cucumbers with rehydration treatment. Although the UAR27 + KV15°C did not obtain the highest total color difference, the shortest processing time and the fastest RR were obtained at this condition. Importantly, the values of the color parameters for the rehydrated sea cucumber from the UAR treatments differed from those of the dried sea cucumber samples, suggesting that the UAR treatment significantly affected the color of the product.
Fig. 4 illustrates the microstructures of the rehydrated sea cucumber samples treated with KV27°C, KV15°C, UAR27 + KV15°C, and UAR15 + KV15°C. The analysis showed that the KV treatments resulted in a compact and organized tissue structure, indicating that the tissue remained thick and packed even after rehydration. In contrast, the UAR treatments resulted in a more depolymerized tissue structure with increased inter-fibre space, higher porosity, and numerous cavities.
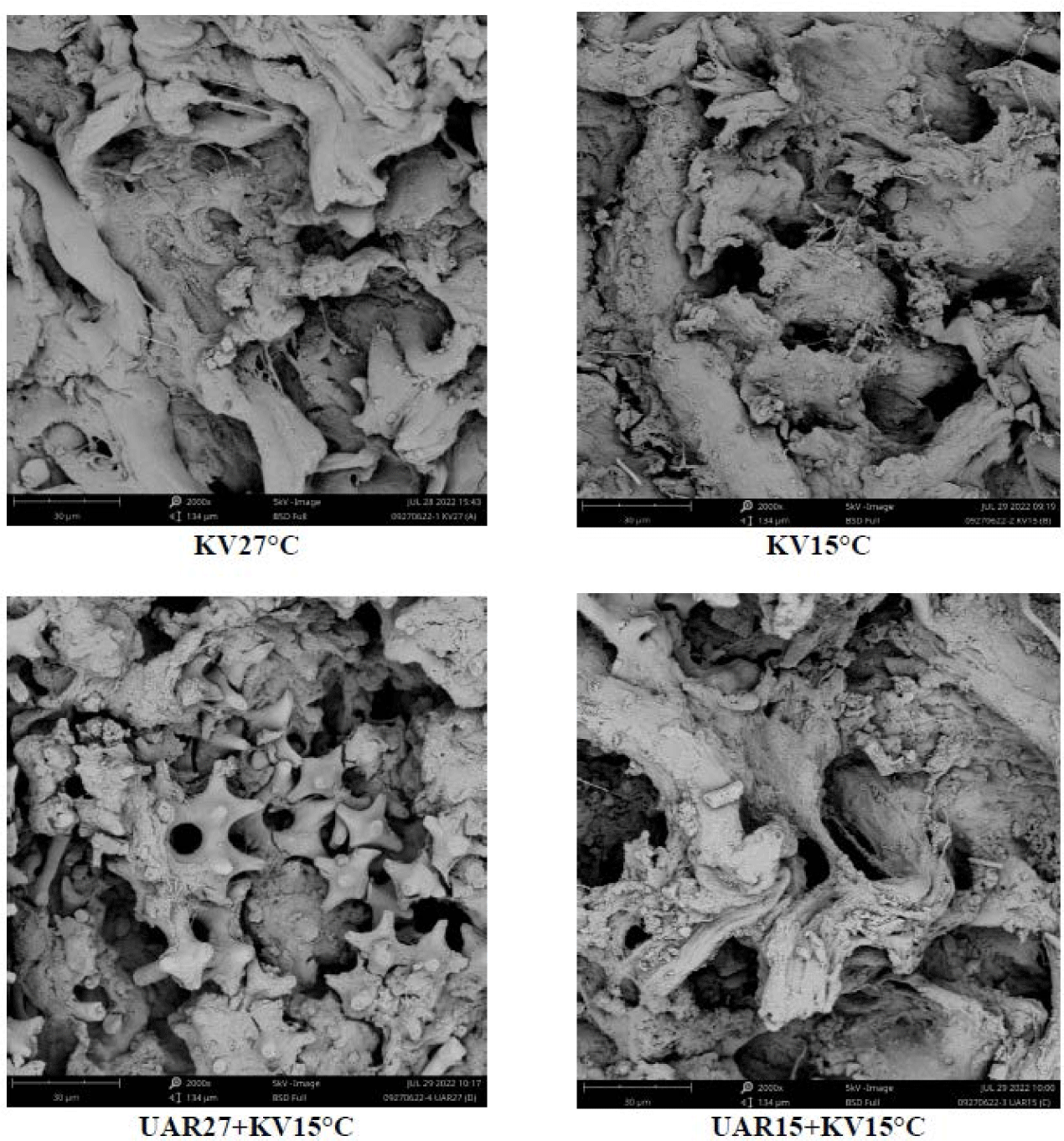
Fig. 5A presents the yield of GAG sulfate extract from sea cucumbers subjected to four different rehydration treatments. Our results demonstrate that the use of the UAR treatment significantly increased the yield of GAG sulfate extracts from sea cucumber, with an average enhancement of approximately 2.9 times compared to the KV treatment (p < 0.05). Notably, the UAR27 + KV15°C treatment yielded the highest amount of 52.8 ± 0.46 µg/g, while the KV27°C treatment produced the lowest yield of 25.3 ± 0.37 µg/g. Moreover, the infrared (FTIR) spectrum of the sea cucumber rehydrated using the four treatments (Fig. 5B) displayed absorption bands indicative of specific functional groups. The signal at 2,964 cm−1 corresponded to the fucose methyl group, while the absorption band at 600–586 cm−1 confirmed the presence of SO2 vibration, and the bands at 1,269–1,253 cm−1 and 870–822 cm−1 verified the presence of sulfate. The absorption at 830 cm−1 indicated the 2.4-O-disulfate fucose or 6-O-sulfated GalNAc group, while the signal at 855 cm−1 showed the presence of 4-O-sulfate Fuc or GalNAc. Additionally, the signal at 1,651 cm−1 represented the asymmetric stretching vibration of C = O from N-acetyl galactosamine and glucuronic acid, and the signal at 1,409 cm−1 indicated the symmetric stretching vibration of CO2 with glucuronic acid. The FTIR spectrum confirmed the presence of sulfated GAGs in the rehydrated sea cucumber. Furthermore, the UAR27 + KV15°C treatment exhibited higher intensity than other treatments.
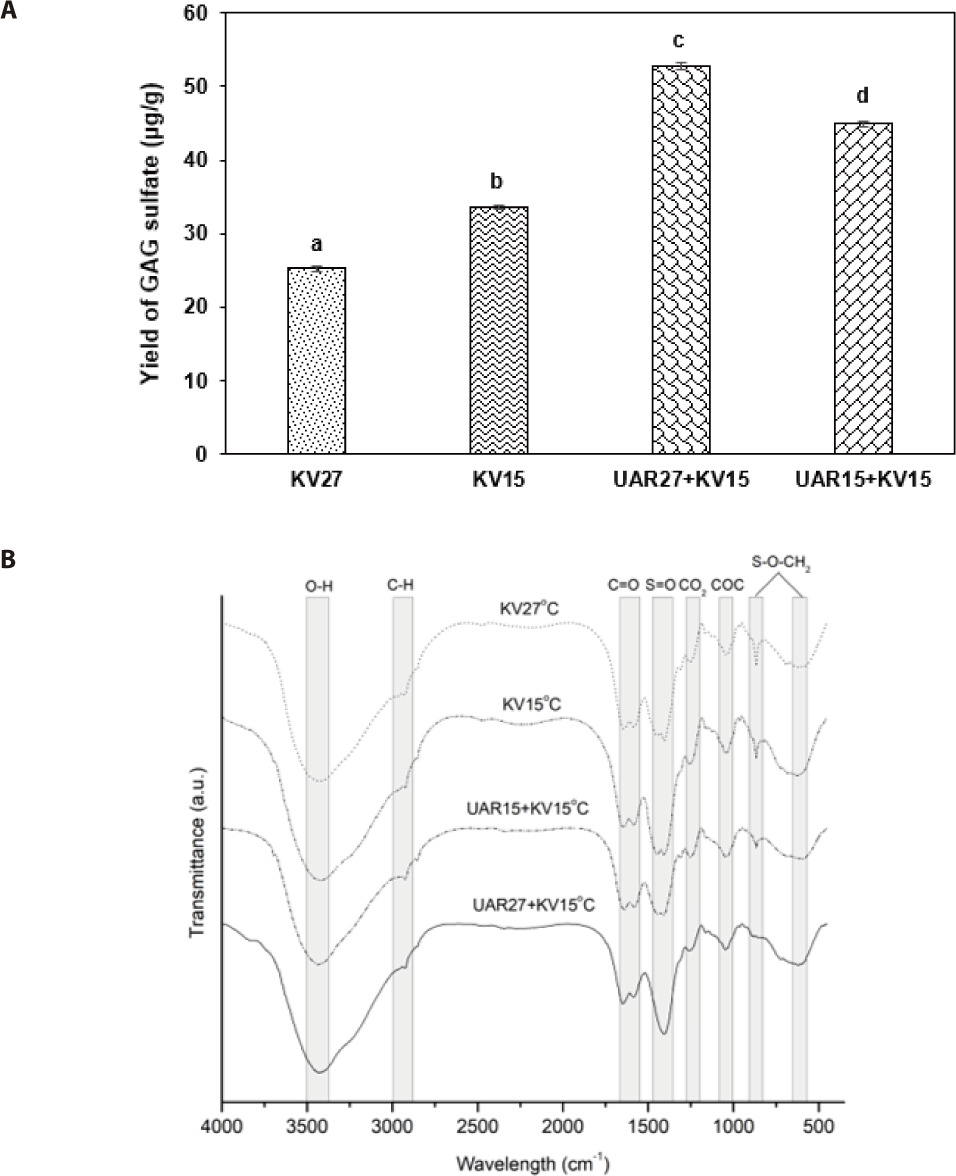
The overall cost of the rehydration process largely depends on the total energy consumed during the operation. Rehydration energy requirements (Wh/g) can be determined by dividing the yield of the sea cucumber (g) by the energy used (Wh) during the process. As presented in Table 3, the total energy consumption during rehydration ranged from 0 to 27.42 Wh/g. Notably, the UAR15 + KV15°C treatment required the highest energy consumption when rehydrated for 48 hours, whereas the KV27°C treatment for 12 hours had the lowest. However, the UAR27 + KV15°C treatment for 28 hours showed a 12.86% reduction in energy consumption compared to UAR15 + KV15°C, followed by KV15°C, which resulted in a 6.01% decrease. It is worth mentioning that even though UAR27 + KV15°C did not accomplish the lowest energy consumption, it yielded the highest rehydration output and produced a higher-quality final product.
Discussion
Generally, the drying and rehydration process can lead to fast water and protein content loss, which can result in a reduction of food quality (Ramadhan et al., 2023). The investigation of the rehydration process is important for optimizing the process and achieving the optimum quality of dried sea cucumber during rehydration. We conducted a simulation using four different rehydration treatments (Fig. 1) to study the changing water absorption ratio of dried sea cucumbers treated with different rehydration methods, as presented in Fig. 2A. The results demonstrate that the moisture content increases exponentially, depending on the rehydration method used. The curves exhibit an increasing rate of rehydration, with accelerated rehydration initially, followed by hydration until a consistent moisture content is achieved (~67% of moisture content or 1.44 kg water/kg dry mass). The time required to achieve a targeted moisture content was 48 hours for UAR15 + KV15°C, 28 hours for UAR27 + KV15°C, 32 hours for KV15°C, and 12 hours for KV27°C. The variation in water absorption among different treatments is attributed to sonication treatment in different temperatures induced by the typical water bond system during food hydration. Our study reveals that the bound water identified in all treatment modalities signifies free water that is confined by the cellular system. UAR15 + KV15°C showed the longest time to achieve the stable moisture ratio, UAR27 + KV15°C then reached the stable moisture ratio after 28 hours. In contrast, the conventional rehydration (KV27°C) revealed the fastest rehydration time, 12 hours. Indicating that the rehydration time is influenced directly by the temperature used, followed by the aids of sonication assisted. This confining action (KV27°C), higher temperature, facilitates more heightened mobility of large molecules, thereby promoting a corresponding cutting of the hydration time. Maintaining a consistent final moisture content in the rehydration process is crucial for ensuring the overall quality and texture of the rehydrated product. This result is consistent with da Silva et al. (2019) finding that the moisture content of approximately 16% (0.19 kg water/kg dry mass) was the same across four ultrasound-drying treatments of nectarines at 60°C. Chen et al. (2016) reported that a consistent moisture content of 41%–53% (d.b.) was achieved for complete dehydration of carrot samples using ultrasound at 65°C. In this simulation, the achieved final moisture ratio was set to investigate whether the quality of final products is influenced by the hydration technique rather than by different moisture content levels. Therefore, different techniques would generate different rehydration times.
Fig. 2B shows that the applied rehydration methods had no significant impact on the RR of sea cucumbers (p > 0.05), particularly with the UAR27 + KV15°C and KV15°C treatments, suggesting that these methods are equally effective in rehydrating the product. However, there was a significant difference (p < 0.05) in the RRs of sea cucumbers treated with KV27°C and UAR15 + KV15°C. Previous studies have reported that food products rehydrated at 27°C exhibit higher RRs than those rehydrated at 15°C (Cox et al., 2012). High temperatures accelerate the degradation of carbohydrate cell walls and increase the rate of hydration (Miano & Augusto, 2018). However, rehydration at 27°C can break internal chemical bonds, decompose collagen fibers, and disrupt the gel structure, which can lead to a decline in quality. The longer the sea cucumbers are rehydrated at 27°C, the faster the quality of sea cucumbers decreases. This damage can be caused by high humidity, which promotes putrefactive microbes (Jiang et al., 2022). On the other hand, a temperature of 15°C has low relative humidity, which slows down bacterial metabolism. Compared to the rehydration performed under KV at 27°C, the UAR27 + KV15°C methods enhance both external and internal mass transfer. UAR is facilitated by cavitation waves that change solid and water pressure and transfer acoustic energy, producing oscillations and microflows, thereby increasing the hydration rate of the material. During the sponge effect, small channels, and cavitation of water molecules in the solid are released (Mulet et al., 2003), aiding in the absorption of moisture by sea cucumbers. Temperature control and ultrasound rehydration time play an important role in maintaining sample quality (Shi et al., 2019). Rehydrating dried sea cucumbers using UAR27 + KV15°C is a recommended option to improve the quality of the final product as it reduces internal resistance while achieving a high RR.
Due to their potential applications in numerous life and medical sciences, the development of marine biological materials is growing significantly (Riyanto et al., 2023). Therefore, it is important to evaluate the quality of rehydrated sea cucumbers for their development as functional food and biomedicine. The present study aimed to characterize the quality of rehydrated sea cucumbers using various analytical methods, including proximate composition, sulfate content, color, microstructure, sulfated GAGs, and energy measurement.
Table 1 shows the proximate composition of rehydrated sea cucumbers treated with different rehydration methods, which showed significant differences (p < 0.05). Notably, the protein content of sea cucumbers treated with UAR followed by low temperature (KV) was higher (UAR27 + KV15°C = 82.18 ± 0.63%, UAR15 + KV15°C = 80.72 ± 0.45%) (d.b), possibly due to the breakdown of cell walls induced by pressure waves generated during UAR and KV treatment. This approach allowed for increased protein extraction without damaging the protein structures. In contrast, no significant differences in carbohydrate and fat contents were found among the samples (p > 0.05). The rehydration method significantly affected the sulfate content of sea cucumbers (p < 0.05). Fig. 3 exhibits that the UAR27 + KV15°C treatment resulted in higher sulfate content, possibly due to the ultrasound waves and cavitation effects at high temperatures, which effectively decomposed the polysaccharide bonds in the material. Additionally, the heating generated by cavitation waves led to the dissolution of polysaccharides, which subsequently broke down the sulfate groups (Li et al., 2019). UAR27 + KV15°C treatment directed to a faster rehydration time compared to UAR15 + KV15°C, resulting in a higher sulfate content. Previous studies have demonstrated that sulfate content affects the binding of polysaccharides to cell wall receptors and that higher sulfate content could potentially enhance the biological activity of sea cucumbers (Mansour et al., 2019). Importantly, the initial results of this study confirmed the potential of UAR, followed by low-temperature rehydration to possess the protein and sulfate content of rehydrated sea cucumbers. These findings highlight the importance of selecting an appropriate rehydration technique to maintain the premium quality of sea cucumbers as raw materials for functional food.
Table 2 presents the color profiles of rehydrated sea cucumbers. Compared to dried sea cucumber, the L*, a*, b*, and ΔE values of the different rehydration treatments were significantly different (p < 0.05). The L*, a*, and b* values increased after processing, regardless of the method used, indicating a lighter, redder, and more yellow hue. The highest L* value was observed in samples treated with UAR15 + KV15°C and UAR27 + KV15°C. Yıldırım & Öner (2015) reported a similar increase in L* when studying the same rehydration methods for chickpeas, with samples dried using UAR, resulting in a lighter color than the conventional method. The total color difference followed the same trend, with UAR treatments exhibiting a higher total color difference than all other rehydration treatments. This may be attributed to the breakdown and removal of pigments and other color compounds by UAR’s cavitation process (Zhang et al., 2016). During rehydration, astaxanthin and melanin can become solubilized and redistributed throughout the tissues, thereby restoring color (Xing et al., 2017). Rehydrated sea cucumber under the UAR27 + KV15°C condition was considered appropriate due to its high total color difference. This treatment significantly impacts the color of the product and could be preferred in industrial or commercial applications that prioritize efficiency and speed of production.
The effect of different rehydration treatments on the microstructural properties of sea cucumbers was observed using SEM (Fig. 4). As shown in Fig. 4, UAR27 + KV15°C and UAR15 + KV15°C displayed a porous structure with large inter-fiber spaces, higher porosity, and numerous cavities due to water penetration during rehydration, compared to KV27°C and KV15°C, which had a more compact and coherent structure, indicating that the tissue remained thick and packed even after rehydration. According to Dong et al. (2014), increased porosity improved the texture and rehydration ability of dehydrated squid fillets compared to products with a compact structure, while Rajewska & Mierzwa (2017) demonstrated that UAR increased the porosity of plant tissues, as observed in this study. As the rehydration temperature of sea cucumbers increased, the pores in their structure gradually expanded (Gao et al., 2019). Continuous high-temperature heating can cause the gel structure of sea cucumbers to decompose, leading to the expansion of pores. Zhang et al. (2016) also reported that continuous ultrasound at high temperatures on the body wall of sea cucumbers could break internal chemical bonds, causing the decomposition of collagen fibers and the gel structure of sea cucumbers. The appearance of gaps between fibers and pores increases the water absorption capacity of sea cucumbers. Sea cucumber absorbs water into its tissues and pores, which is physically adsorbed with high fluidity. The ultrasound sponge effect causes sea cucumbers to swell faster and form a structure with a higher pore density. Capillarity is the main means of water transport in the microstructure, facilitating better and faster water absorption (Dong et al., 2014). The higher the porosity, the more space will be formed inside or outside the myofibril, which is more favorable for bound and adsorbed water. The result suggests that the UAR27 + KV15°C treatment would substantially affect the microstructure of rehydrated sea cucumbers.
Holothurian GAGs is a group of macromolecular long linear polymers derived from disaccharide subunits. It is naturally polyanionic and can interact with functional proteins needed by the body, which are isolated from the body wall of sea cucumbers. The affinity of these molecular complexes is largely driven by electrostatic interactions, in which the sulfation content of GAG plays an important role (Pomin, 2009). The isolated GAGs have been characterized by FTIR (Fig. 5B) and their yield. Sulfate detected in ultrasound and conventional rehydration treatments can absorb SO3 and SO2, with the highest value in the ultrasound rehydration treatment. Differences were also seen in the absorption band treated with ultrasound rehydration, resulting in a higher intensity than without ultrasound. This can be seen from the high transmittance value and the wide distribution band of sea cucumbers. Thus, the crude extract of sulfated polysaccharides with UAR treatment could show the potential of bioactive compounds as better anticoagulant candidates.
In the present study, the different rehydration treatments had a significant effect on the yield of GAG sulfate crude extract of rehydrated sea cucumbers. As shown in Fig. 5A, the UAR27 + KV15°C treatment significantly had the highest yield of GAG sulfate, with an increase of approximately 2.9 times compared to the other treatments (p < 0.05). The fundamental principle of UAR involves utilizing strong cavitation, mechanical vibration, and heating effects generated by ultrasonic waves on the medium to allow the solvent to penetrate the sample under specific conditions (Li et al., 2019). In the case of GAG sulfate extraction, ultrasound was already used as a pre-treatment method before water bath extraction. Therefore, rehydration can facilitate the extraction by pre-hydrating the tissue and enhancing the contact area between the tissue and the solvent, resulting in a higher yield of GAG sulfate within a shorter period for the UAR treatment. The yield of crude extract of sulfated GAG from sea cucumbers varies depending on the process conditions and raw materials used (Hu et al., 2012). The yield is related to the amount of bioactive content. These compounds have various benefits for human life, such as anticoagulant and antioxidant, antibacterial, and anti-inflammatory properties (Zhang & Cha, 2022; Zhu et al., 2020).
The structure of sea cucumber sulfate GAG, formed by disaccharide units, is 4-glucuronic acid (GlcA) and 3-N-acetyl galactosamine (GalNAc) connected by a branch of the α-L-Fuc unit, where the GalNAc unit can mostly be 2,4-sulfate (CS-A) or mostly 6-sulfate (CS-C) (Mourão et al., 2001). The lateral units of the GAG can show different sulfation patterns according to the sea cucumber species. In general, the presence of sulfation patterns and the type of glycosylation are the main components for determining anticoagulant activity (Chen et al., 2012). The high 4-sulfation level in the galactosamine unit, combined with a certain 2-sulfation level in the iduronic acid unit, is a structural requirement of sea cucumber GAG anticoagulants. Other factors that influence anticoagulant activity are molecular weight and composition of monosaccharides (Pomin, 2014).
The UAR27 + KV15°C rehydration treatment for 28 hours required 6.01% lower energy than the other treatments. This is influenced by the faster rehydration time. The output of sea cucumbers in one rehydration process produces a higher yield so that the energy required is lower than the control and other treatments with a value of 14.55 Wh/g.
This, in turn, could lead to the development of a new functional technique of the rehydration method of sea cucumber, which could offer a range of health benefits for consumers. Finally, these current findings may have significant implications for further developing functional food products based on sea cucumber extracts.
Conclusion
The combined effect of UAR and subsequent low-temperature treatment on sea cucumbers demonstrated significant improvements in water absorption ratio, RR, proximate composition, sulfate content, color intensity, tissue microstructure, GAG sulfate yield, functional groups, and energy measurements. Specifically, the UAR27 + KV15°C rehydration treatment with a duration of 28 hours was identified as the optimal method. This novel approach allows for the acceleration of the rehydration process of sea cucumbers while maintaining their physical, chemical, and biological properties.