Introduction
The use of plant extracts or phytobiotics for enhancing fish growth and health is an important concern in aquaculture nutrition. Phytobiotics are rich in various bioactive compounds such as alkaloids, essential oils, flavonoids, terpenoids, saponins, tannins, and steroids, which have biochemical properties to enhance growth and act as an immunostimulant, antiviral, antibacterial, and antiparasitic agent (Jana et al., 2018). For instance, Abd-Elaziz et al. (2023) observed growth promoting, immunostimulatory and ammonia-N stress resistance effects of herbal extracts (Moringa oleifera, Ginkgo biloba, Silybum marianum, Myristica fragrans and Astragalus membranaceus) for Pangasianodon hypophthalmus fingerlings. Walnut (Juglans regia) leaves extract was found to have immunomodulatory, gene expression responses and disease resistances in Oreochromis niloticus (Yilmaz et al., 2023). Chakraborty et al. (2014) demonstrated that application of phytochemicals as feed additives are potential growth promoters and endocrine modulators in fish culture. Moreover, use of phytobiotics in aquaculture is cost-effective, more biodegradable, and has less bacterial resistance and residual accumulation in tissues (Abdel-Latif et al., 2023; Abdul Kari et al., 2022; de Rezende et al., 2021).
Black pepper (Piper nigrum Linn) is a crucial medicinal remedy that contains phenols, flavonoids, alkaloids, volatile oils, steroids, terpenes, proteins, carbohydrates, fibers, polysaccharides, amides, and lignans (Meghwal & Goswami, 2013). It also contains 3.5% of essential oils, which constitutes abinene, pinene, limonene, linalool, and phellandrene (Murlidhar & Goswami, 2012), and a variety of phytoconstituents viz. piperine, piperamine, piperamide, pipereidine, pipericide, piperettine, tricholein, sarmentine, sarmentosine and carvone (Ghosh et al., 2014). Black pepper as a whole or its secondary metabolites characterize therapeutic effects, including anti-inflammatory, growth stimulatory, chemopreventive, and immunomodulatory activities. Piperine is the primary bioactive alkaloid in several pepper species, mainly in black pepper (Srinivasan, 2007). Black pepper contains 3%–8% of piperine and its isomers; isopiperine, chavicine, and isochavicine (Mohammed et al., 2016).
Piperine has various pharmacological potencies, including immunomodulatory, antioxidant, anti-inflammatory, antibacterial, antifungal, antihyperlipidemic, antitumor, and anti-apoptotic actions (Ahmad et al., 2012). Piperine was found to enhance the activity of phagocytic cells, complement activity, stimulate the natural killer cells, and proliferate lymphocytes, antibody and lysozyme responses in fish (Bae et al., 2010). Piperine manifests anti-inflammatory activities by inhibiting production of TNF-α, lipopolysaccharide-induced endotoxin shock, and leukocyte accumulation (Bae et al., 2010). Along with the array of pharmacological effects, piperine has been found to improve the bioavailability of drugs (rifampicin, tetracycline, and sulfadiazine) and other nutritive substances such as carotene, pyroxidine, curcumin, glucose, and amino acids (Gorgani et al., 2017). Furthermore, piperine has been shown to stimulate digestive enzyme activities in fish, improve digestion, and reduce gastrointestinal food transit time (Platel & Srinivasan, 2000). Several studies have assessed the pharmacological effects of black pepper, its extracts, or its primary bioactive compound, piperine on growth and health aspects of aquatic species (Matiullah et al., 2016; Nilavan et al., 2017). Dietary piperine favorably affected the haemato-immunological responses in Indian major carp (Labeo rohita), with enhanced growth and resistance against Aeromonas hydrophila (Nilavan et al., 2017). Punitha et al. (2008) observed that piperine could improve innate immunity, disease resistance against Vibrio harveyi infection and growth indices in grouper (Epinephalus tauvina). Moreover, piperine was found as a potential antiparasitic agent for controlling Argulus spp. parasite on goldfish (Carassius auratus) (Kumar et al., 2012).
In aquaculture, using black pepper or piperine as an immuno-stimulant is a new frontier alternative to complementary therapies for curing an array of diseases. However, there is a dearth of information on the effect of dietary piperine on aquatic animals. Further, it needs to quantify dose-dependent stimulatory effects on fish growth, immunity, and physiology. Red seabream (Pagrus major) is a widely cultured marine fish species in Korea. To our knowledge, no studies have investigated the supplementary effects of dietary piperine on immune response, growth and health characteristics of red seabream. Therefore, this study aimed to determine the effects of dietary piperine as a natural immuno-stimulant additive in formulated diets for red seabream.
Materials and Methods
Juvenile red seabream were obtained from a hatchery (Daedong susan, Gimhae, Korea) and acclimated to the experimental conditions for 15 days, rearing in circular fiberglass tanks while being fed a commercial diet (containing 87.5% dry matter, 52% crude protein, 12% crude lipid and 10.8% ash; Suhyup Feed, Seoul, Korea). During the acclimation, feed was supplied three times per day (8:30, 12:30, and 17:30 h) until apparently satiation. The photoperiod was maintained at 12 h:12 h light/dark condition daily. An automated flow-through system delivered filtered seawater (4 L/min) continuously to the tanks. The rearing water was continuously aerated to maintain optimum dissolved oxygen. The Institutional Animal Care and Use Committee of Jeju National University authorized the experimental protocols for the feeding trial (approval number-2018-0052).
Six experimental diets with 45% crude protein and 15% crude lipid were formulated as isonitrogenous and isolipidic. Diets were supplemented with piperine at 0.0, 0.25, 0.50, 1.00, and 2.00 g/kg levels (Con, P25, P50, P100, and P200, respectively) or black pepper powder at 1.0 g/kg (BP100). Piperine and black pepper powder were provided by Synergen, Bucheon, Korea. At the mixing process a premix was prepared with carboxymethyl cellulose (CMC) and piperine at a ratio of 1:0.2 which was equivalent to P200 diet. For each diet an adequate amount of premix was provided to fulfill the required piperine level. According to the formulation, the CMC required to equal 1% CMC of each diet was provided. The dry ingredients, distilled water (15%) and fish oil were measured according to the formulation and thoroughly stirred together using a food mixer (NVM-14, Daeyung, Seoul, Korea); each wet dough was pelleted to 3 mm diameter. Pellets were dried for 8 h at 25°C to achieve a moisture content of < 10%. Diets were stored at 25°C under vacuum conditions. Standard techniques were used to measure the diet dry matter, protein, and ash contents (AOAC, 2005). Lipid content was analyzed as described by Folch et al. (1957). The formulation and proximate composition of the experimental diets are shown in Table 1.
7) Mineral premix (g/kg mixture): MgSO4·7H2O, 80.0; NaH2PO4·2H2O, 370.0; KCl, 130.0; Ferric citrate, 40.0; ZnSO4·7H2O, 20.0; Ca-lactate, 356.5; CuCl2, 0.2; AlCl3. 6H2O, 0.15; Na2Se2O3, 0.01; MnSO4·H2O, 2.0; CoCl2·6H2O, 1.0: Korea Thumb Vet, Iksan, Korea.
8) Vitamin premix (g/kg mixture): L-ascorbic acid, 121.2; DL-α tocopherol acetate, 18.8; thiamin hydrochloride, 2.7; riboflavin, 9.1; pyridoxine hydrochloride, 1.8; niacin, 36.4; Ca-D-pantothenate, 12.7; myo-inositol, 181.8; D-biotin, 0.27; folic acid, 0.68; p-aminobenzoic acid, 18.2; menadione, 1.8; retinyl acetate, 0.73; cholecalciferol, 0.003; cyanocobalamin, 0.003: DSM, Heerlen, Netherland.
Juvenile fish (7.6 ± 0.1 g) were randomly stocked into 18 circular tanks (220 L) with 30 fish per tank density. Triplicate groups of fish were assigned to each experimental diet and fed to apparently satiation three times (8:30, 12:30, and 17:30 h) daily. After 30 min of each feeding, uneaten diets were siphoned out and dried. The dry weight was subtracted from the amount fed to calculate feed intake. Filtered seawater was continuously supplied to the tanks by a flow-through system (4 L/min rate) and aerated to maintain optimum dissolved oxygen level. The growth trial was conducted for 8 weeks. The rearing water quality was maintained as follows: temperature 23.1 ± 1.7°C, salinity 31 ± 1 ppt, dissolved oxygen 7.65 ± 0.91 mg/L, pH 8.28 ± 0.25, and ammonia 0.062 ± 0.006 mg/L. A 12 h:12 h (light/dark) photoperiod was maintained daily.
After the 8 weeks of the feeding trial, feeding ceased 24 h before weighing and sampling to lessen the stress on fish. Fish count in each tank and individual weight were obtained to determine growth indices; average final body weight (FBW), weight gain (WG), specific growth rate (SGR), condition factor (CF), hepatosomatic index (HSI), feed conversion ratio (FCR) and protein efficiency ratio (PER). Four fish were randomly captured from each tank and anesthetized using 2-phenoxyethanol (Sigma-Aldrich, St. Louis, MO, USA, 77699) 200 ppm concentration solution. Blood samples were taken from the caudal vein, and clotting was allowed at room temperature for 30 min. Then samples were centrifuged for 10 min at 5,000×g to separate the serum. Another set of blood samples were taken from heparinized syringes and centrifuged for 10 min at 5,000×g to separate plasma (Hasanthi et al., 2023). Plasma and serum samples were stored at –80°C for biochemical and immune analysis. For histological observations, 1 cm length anterior intestine samples (1 cm posterior to pyloric caeca) were taken and stored in Bouins’ solution to fix the tissues. For gene expression analysis, liver samples were obtained in a sterile condition, subsequently immediately frozen in liquid nitrogen, and stored at –80°C.
Serum myeloperoxidase (MPO) activity was determined as described by Medagoda & Lee (2023). Using the technique outlined in Anderson et al. (1992), phagocyte oxidative radical generation during respiratory burst was quantified using the nitro blue tetrazolium (NBT) test. Whole blood samples were used for the NBT assay. A turbidimetric assay proceeded to analyze serum lysozyme activity. Turbidity decrease due to the lysis of the Micrococcus lysodeikticus bacteria was measured, as mentioned in Hasanthi & Lee (2023). According to the Ellis (1990) method, the serum antiprotease activity was assessed by its capacity to inhibit trypsin activity. The method described in Siwicki et al. (1994) was used to determine the levels of plasma total immunoglobulin (Ig). The enzyme activity of serum superoxide dismutase (SOD) was measured using an assay kit (Sigma-Aldrich, 19160). The microhematocrit technique determined hematocrit by centrifuging the whole blood in capillary tubes at 650×g for 10 min. Hematological parameters of hemoglobin (using whole blood), plasma glucose, plasma triglyceride, plasma cholesterol, and plasma total protein, were determined by a blood analyzer (SLIM, SEAC, Florence, Italy).
Fixed intestinal samples were dehydrated using ethanol series. Following the embedding of sample segments in molten paraffin wax, the resultant blocks were put on a microtome and sectioned to a thickness of 5 µm. Slices were attached to microscope slides, then rehydrated after being xylene-deparaffinized. For general histological analysis, samples were stained with Harris hematoxylin and 0.5% eosin. Using the ImageJ 1.44 analysis program, the villus length was measured.
The liver samples were treated with the TRIzol reagent (Sigma-Aldrich, T9424) to extract the total RNA. The extracted RNA concentration was determined at 260 nm using a NanoDrop spectrophotometer (Thermo Scientific, USA). Total RNA of 2.5 μg was reverse transcribed using a Prime Script ™ first-strand cDNA synthesis kit (TaKaRa, Japan), diluted 40-fold, and then stored at −80°C. For the qPCR analysis, a reaction mixture containing 0.5 μL of the primer (10 pmol/μL), 5 μL of TaKaRa Ex Taq™ SYBR premix, 3 μL of diluted cDNA and 5 μL of dH2O in a total volume of 10 μL was assessed. The 18S rRNA (GenBank accession no. AY550956) was considered a housekeeping gene. Based on the NCBI GenBank, the gene primer sequences were created (IGF-I, accession no: CM040953.1). The PCR program was as follows: one cycle at 95°C for 10 s, followed by 45 cycles at 95°C for 5 s, 58°C for 20 s, and 72°C for 20 s. According to Pfaffl (2001) [2−ΔΔCT; threshold cycles (CT)], relative mRNA expression values were calculated.
Data were analyzed by one-way analysis of variance (ANOVA) in SPSS version 22.0 (SPSS, Chicago, IL, USA). The Tukey’s HSD test (p < 0.05) was used to compare the mean values when ANOVA revealed differences between the groups. Data were presented as mean ± SD, and all the percentage data were arcsine transformed before the analysis.
Results
The supplementary effects of piperine and black pepper on growth performance and feed utilization are shown in Table 2. Supplementation of dietary piperine (0.25‒2.0 g/kg) significantly increased (p < 0.05) the FBW, WG, and SGR compared to the Con group. A significantly lower (p < 0.05) FCR and higher PER were observed with 0.5‒2.0 g/kg dietary piperine compared to the Con diet. Higher (p < 0.05) CF was observed in fish fed with dietary piperine or black pepper. HSI was decreased (p < 0.05) in fish fed with BP100 compared to the Con group. Liver IGF-I mRNA expression (Table 3) and fish survival rate did not affect (p > 0.05) by either piperine or black pepper.
2) Weight gain (WG, %) = 100 × [Final body weight (g) – Initial body weight (g)] / Initial body weight(g).
3) Specific growth rate (SGR, % day–1) = 100 × (ln final body weight − ln initial body weight) / Days.
4) Feed conversion ratio (FCR) = Dry feed intake (g) / [Final body weight (g) – Initial body weight (g)].
IGF-1 | |
---|---|
Con | 1.00 ± 0.26 |
P25 | 1.32 ± 0.64 |
P50 | 0.86 ± 0.24 |
P100 | 0.95 ± 0.21 |
P200 | 0.82 ± 0.18 |
BP100 | 1.08 ± 0.45 |
Piperine supplementation enhanced innate immunity and antioxidant enzyme activity (Table 4). Compared to the Con group, dietary piperine significantly increased (p < 0.05) the MPO and antiprotease activities, while the highest values were observed at the 1.0 g/kg level. Lysozyme activity and Ig levels were increased (p < 0.05) with the BP100 diet and piperine at 0.5–2.0 g/kg levels, while the maximum values were observed with the BP100 group. Similarly, SOD activity exhibited significantly higher (p < 0.05) values at piperine 0.25‒2.0 g/kg levels and with the BP100 diet.
Hematological parameters of total protein and glucose did not significantly alter by the dietary piperine or black pepper (Table 5). Although plasma hematocrit and hemoglobin values did not show significant difference (p > 0.05) between Con and BP100 groups, lower levels of hematocrit and hemoglobin were observed in fish fed BP100 diet compared to con diet. However, plasma cholesterol was significantly decreased (p < 0.05) by the dietary piperine 0.5‒2.0 g/kg or BP100 diet.
The comparative analysis of the fish intestine morphometrics is shown in Table 6 and Fig. 1. Intestinal villi height and mucus-secreting goblet cells were increased when supplementing black pepper or piperine into the diets. A significantly higher (p < 0.05) goblet cell count was shown in the fish fed with piperine at 0.25 g/kg or BP100 diet. Dietary piperine 0.25–1.0 g/kg levels significantly increased (p < 0.05) the intestinal villi length of red seabream.
GC1) | VL2) | |
---|---|---|
Con | 571 ± 344c | 722 ± 33d |
P25 | 2,069 ± 458a | 1,543 ± 277a |
P50 | 1,038 ± 458bc | 1,144 ± 111b |
P100 | 957 ± 27bc | 1,041 ± 138bc |
P200 | 725 ± 120c | 944 ± 101bcd |
BP100 | 1,311 ± 189b | 811 ± 40cd |
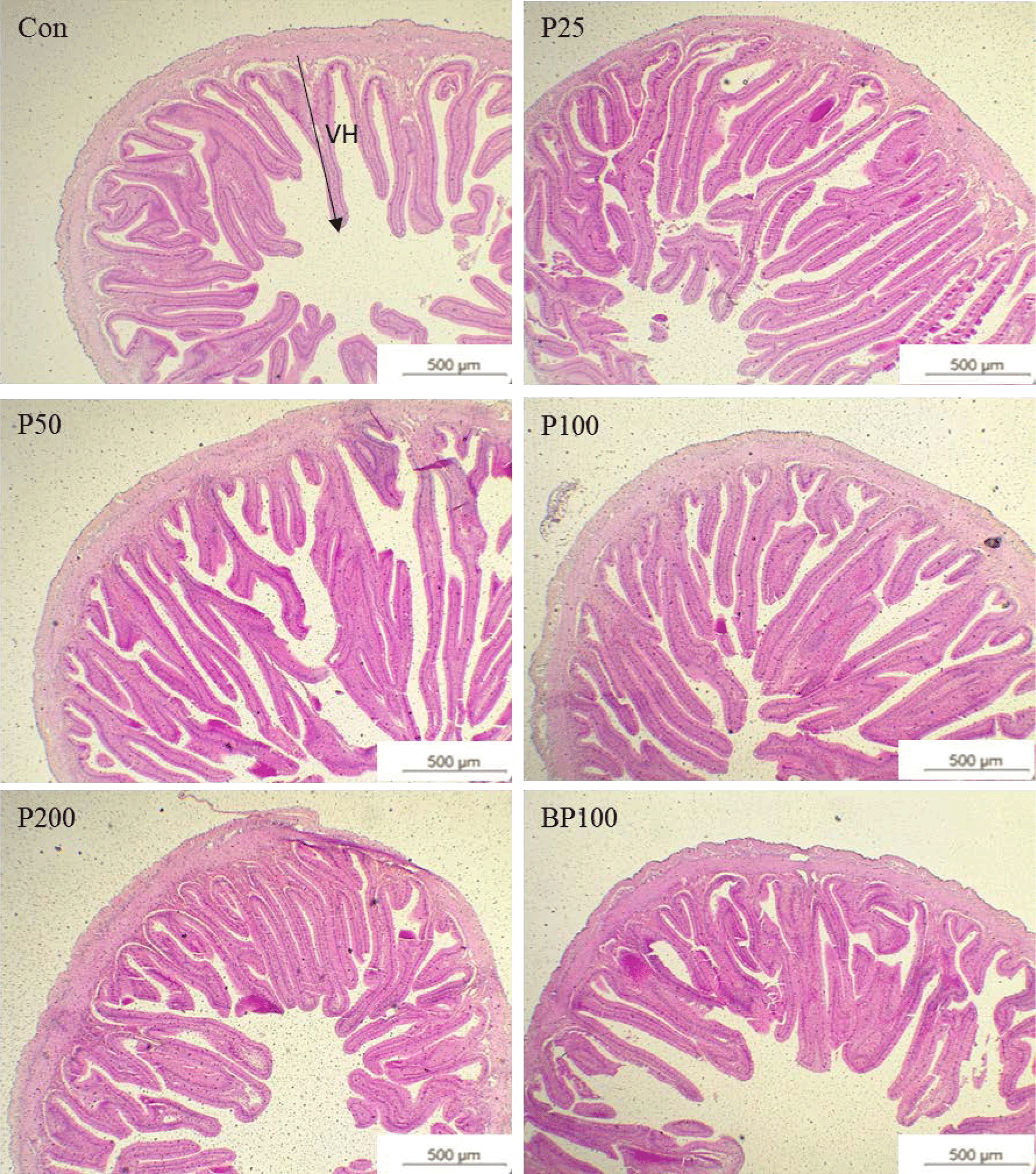
Discussion
The present study revealed that black pepper and its major bioactive compound, piperine, favorably affected to improve the growth performance, innate immunity, hematological parameters, and intestinal morphology in red seabream. Similarly, in several studies, black pepper and piperine were recognized as growth promoters when administered to cultured fish. For example, dietary supplementation of black pepper enhanced the growth and feed utilization efficiency in Indian major carp, whereas the 0.5% level exhibited the highest growth performance (Matiullah et al., 2016). Similarly, Nilavan et al. (2017) observed that dietary inclusion of piperine improved the WG, SGR, FCR, and PER in rohu carp and found higher performance at 0.2% level. A diet containing plant extract of long pepper (Piper longum), bermuda grass (Cynodon dactylon), ginger (Zingiber officinalis), coat buttons (Tridax procumbens), and stonebreaker (Phyllanthus niruri) improved the WG by 41% in grouper (E. tauvina) (Punitha et al., 2008).
In previous studies, piperine and black pepper have been found to increase nutrient digestibility and bioavailability, resulting in higher protein synthesis, feed conversion, and fish growth (Nilavan et al., 2017; Shin et al., 2023). Piperine has been found to improve the gastrointestinal functions of fish (Meghwal & Goswami, 2013). It increases pancreatic digestive enzyme secretion (lipase, trypsin, chymotrypsin, and amylase), stimulating nutrient digestion (Meghwal & Goswami, 2013). Furthermore, piperine ingestion was found to increase gastric secretions, saliva, and intestinal mucosa in mice studies (Bajad et al., 2001; Capasso et al., 2002). Ahmad et al. (2012) demonstrated that piperine activated the jejunum epithelial cells and membranes, enhancing nutrient absorption in rats. Khajuria et al. (2002) reported that piperine improves enzyme activities due to its protein folding ability, thereby modifying the ultrastructure of enterocytes and increasing intestinal villi length. Moreover, it has been shown that piperine and black pepper alter the passive permeability and fluidity of the brush border membrane, which is connected to the lengthened microvilli and the intestine’s absorptive surface.
Moreover, piperine and black pepper were evidenced to change brush border membrane fluidity and passive permeability properties, associated with the increased microvilli length and the absorptive surface of the intestine (Srinivasan, 2016). Similar results were observed in the present study, along with previous studies, which increased intestinal microvilli length and mucus-secreting goblet cells. Thus, improved growth, feed utilization efficiency, and intestinal morphology in this study are possibly due to the effect of the piperine. However, the mechanism which contributes to nutrient bioavailability has not been distinguished. It is hypothesized that the mechanism attributed to enhanced absorption may be caused by modulation of the enzyme secretion and altered membrane lipid dynamics in the intestine.
Plasma cholesterol is regulated through de novo synthesis and bile acid formation in the liver. Our study observed reduced plasma cholesterol and HSI in fish fed piperine and black pepper. Vijayakumar & Nalini (2006) reported that piperine has a stimulatory effect on the excretion of cholesterol as bile acids implying its hypocholesterolemic effect. Increased cholesterol excretion as bile acids may be attributed to reducing plasma cholesterol in fish fed with piperine. It may be caused by decreased liver cholesterol production and increased 7α hydroxylase activity in the liver. Moreover, dietary piperine supplementation showed a high cholesterol 7α hydroxylase activity, inhibiting cholesterol biosynthesis in rat liver (Srinivasan & Sambaiah, 1991). Thus, decreased cholesterol biosynthesis and increased cholesterol excretion may lower total plasma cholesterol and HSI in fish fed with piperine and black pepper. Furthermore, in this study, supplementation of black pepper showed a slight reduction of plasma hemoglobin and hematocrit compared to Con diet. Ullah et al. (2021) showed contradictory results an increase in red blood cells, total leukocyte count and lymphocytes in Labeo rohita with black pepper leaf extract supplementation. Hassan et al. (2023) showed that red pepper oil supplementation significantly increased the red blood cells count and hemoglobin in broilers. Therefore, based on our results of decreasing hemoglobin and hematocrit we cannot directly assume that black pepper can reduce red blood cells in fish, and further studies are recommended for more investigations.
The results of this study demonstrated that dietary piperine and black pepper could enhance antioxidant enzyme activity and innate immunity of fish. Various in vitro and in vivo experimental findings have demonstrated the radical scavenging and antioxidative properties of black pepper and its active constituents (Andrade & Ferreira, 2013; Hu et al., 2022). It is reported that black pepper has antioxidative and DNA damage protective activities due to the presence of phenolic compounds and flavonoids (Andrade & Ferreira, 2013). It favorably inhibits lipid peroxidation, prevents high-fat diets induced oxidative stress, quenching free radicals of superoxides and hydroxyl anions, and inhibits lipoxygenase and carcinogenesis (Hu et al., 2022). Moreover, it was found that black pepper or piperine reduces thiobarbituric acid-reactive substances and maintains the SOD, catalase, glutathione (GSH) levels, GSH S-transferase and GSH peroxidase antioxidative enzyme activities in rats fed with high-fat diet (Gorgani et al., 2017; Vijayakumar et al., 2004). Piperine exhibits liver protective activity mainly due to its antioxidative mechanism. Studies conducted in vivo and in vitro have demonstrated that piperine could reduce lipid peroxidation and alleviates the degradation of GSH and total thiols (Khajuria et al., 1999). Sabina et al. (2010) reported that the chemoprotective activity of piperine functioned against liver damage in mice showing hepatoprotective effect due to its radical scavenging ability. Nahak & Sahu (2011) demonstrate that black pepper is rich in glucose-6-phosphate dehydrogenase and GSH peroxidase enzymes, which can protect against oxidative damage. Moreover, Zarai et al. (2013) also revealed that black pepper and its active piperine were examined for antioxidative potencies comparable to α-tocopherol.
The present study found that oral administration of piperine has caused the fish immunostimulant by increasing lysozyme activity, MPO activity, antiprotease activity, and plasma Ig. Lysozyme is an antimicrobial enzyme that modulates host immune responses to infections causing bacterial cell lysis (Ferraboschi et al., 2021). MPO is a peroxidase enzyme released by neutrophils to defend against invading pathogens and inflammations (Frangie & Daher, 2022). Antiproteases prevent inflammatory damage caused by excess proteases (Meyer & Jaspers, 2015). Ig are antibodies and function to recognize and bind to specific antigens, such as bacteria and viruses, leading to their destruction (Woof & Kerr, 2006). Piperine is known to have a therapeutic effect on inflammation and innate immunity. Vijayakumar et al. (2004) demonstrate that piperine attenuates TNF-α induced IkB kinase activity and blocks phosphorylation and degradation of IkBα. Further, the administration of piperine could inhibit the collagen matrix invasion of melanoma cells and reduce the pro-inflammatory cytokines of IL-6, IL-1β, and TNF-α (Singh & Duggal, 2009). Piperine administration inhibited type 1 interferon production and lipopolysaccharide-induced endotoxin shock in mice, considering it could be a functional gastrointestinal anti-inflammatory agent (Bae et al., 2010). In several studies, piperine has been shown to improve the innate immunity of aquatic animals (Nilavan et al., 2017; Shin et al., 2023). For example, dietary piperine supplementation at 0.2% showed a stimulatory effect on haemato-immunological responses, increasing respiratory burst activity, hemoglobin level, lysozyme, and phagocytic activity along with the improved resistance against A. hydrophila infection in Labeo rohita (Nilavan et al., 2017).
Black pepper and piperine have been investigated in various studies and confirmed their antibacterial and antiparasitic effect against different pathogenic species. Blessy et al. (2015) showed the antibacterial property of P. nigrum against Staphylococcus spp. Gupta et al. (2013) observed that black pepper extract exhibited potent antibacterial activity against Escherichia coli. Further, antibacterial activity of the black pepper oil was discovered to be functional in inhibiting the biofilm formation of Pseudomonas aeruginosa on Penaeus monodon (Swathy et al., 2018). Zarai et al. (2013) revealed that the antibacterial potency of the P. nigrum could be highly related to the containing phenolic compounds and flavonoids. The mechanism of antibacterial action was found to be loss of cell membrane permeability and inhibition of bacterial biofilm and efflux pump (Karsha & Lakshmi, 2010). Antifungal properties of pepper species, especially in piperine, were determined mainly by the lysis of the cytoplasmic membrane and fungal cell wall (Pundir & Jain, 2010). In this regard, Kumar et al. (2012) showed the antifungal and antiparasitic effect in which in vivo and in vitro effects of piperine resulted in 100% mortality of Argulus spp. at 9.0 mg L–1, on goldfish. Several studies have demonstrated the clinical effect of piperine on innate immunity, antioxidant activity, and anti-inflammatory and antiparasitic activities in rat models and fish species (Derosa et al., 2016; Dogra et al., 2004; Nilavan et al., 2017); however, the underlying mechanism has not yet been comprehensively understood, and further detailed studies need to be performed for more thorough investigations.
In conclusion, the optimum level of dietary piperine for the fish diet would be 0.25‒1.0 g/kg. Dietary piperine supplementation could increase innate immunity, antioxidant enzyme activity and growth performance in fish. In this regard, black pepper and piperine can be potent immunostimulants for aquatic animals. Piperine could increase the intestinal microvilli length and consequently improve the feed utilization efficiency of the fish.