Introduction
The far eastern catfish (Silurus asotus) is a freshwater fish from the Order Siluriformes and Family Siluridae; it is widely found in rivers, reservoirs, and lakes in South Korea. It is also found distributed abroad in China, Taiwan, and Japan (Kim, 1997). In nature, this species is mainly found in slow-flowing and muddy environments, and feeds at night on fish, fry, and small aquatic insects (Kim & Park, 2002). S. asotus is the most common species in inland aquaculture farms in Korea. Owing to its white color, rich flavor, and nutritional characteristics, it is consumed in soups or as steamed fish by the elderly during pregnancy, postpartum, and illness (Gye et al., 2015; Sung & Shim, 1981).
Following the drop in production of naturally caught S. asotus in Korea from approximately 1,000 tons in 1986 to approximately 300 tons in 1989 due to water pollution and the deterioration of the natural environment, the demand for the development of aquaculture technology has increased (Choi et al., 1992). Therefore, many studies have been conducted, including cytophysiological and genetic studies (Jo & Kim, 1999; Yoon et al., 1995), scattering induction (Choi et al., 1992; Kwon et al., 1996; Lee et al, 1990), disease reports (Han et al., 1993; Kim & Lee, 1993; Park & Kim, 1994), and the use of additives to increase aquaculture production of S. asotus (Lee et al., 1998).
The number of S. asotus fish farms in Korea increased to 227 in 2005 and 239 in 2010; however, the numbers decreased to 199 in 2015 and 146 in 2020 and gradually decreased (KOSIS, 2005, 2010, 2015, 2020). In 2021, S. asotus was the second most farmed fish species in the inland aquaculture industry, following eel (Anguilla japonica) (KOSIS, 2021). Fish farm production of S. asotus exceeded 5,000 tons in 2017 when 5,330 tons were produced. However, production decreased to 3,783 tons in 2021, and this decreasing trend continues. In recent years, attempts have been made to develop a stagnant catfish farming industry through technological development and industrialization of S. asotus farming that combines eco-friendly aquaculture with biofloc technology and asquaponics (Kim et al., 2022; Park et al., 2021).
The number of S. asotus farms in outdoor aquaculture decreased from 227 in 2005 to 146 in 2020; however, proportion of the catfish farms increased from 43.6% (99/227) to 59.6% (87/146) (KOSIS, 2005, 2020). Nevertheless, little is known about managing the main aquaculture methods, such as planting, feeding, and sales index in outdoor fish farming.
Therefore, the primary objective of this study was to monitor all culture processes, including stocking, feeding, and growth rate, along with the associated changes in water quality and selling, in outdoor catfish farms, which constitute the predominant domestic catfish farming method. Further, based on the present study’s findings (based on detailed fish farm data), we aimed to report the current state of inland catfish farming in Korea and provide fundamental data for policymaking and technological development in the catfish aquaculture industry.
Materials and Methods
An outdoor aquaculture farm for S. asotus in Kimje-si, Jeollabuk-do, Korea was monitored directly from June 2018 to October 2019. Data on the same farm from 2017 were also obtained. The S. asotus fish farm in this study had a floor area of 3,305 m2 and a capacity of approximately 1,000 tons with less than one daily ground water rotation. The feeding started in May 5 of 2017, June 2 of 2018 and May 11 of 2019 when catfish were input, and ended in October 11 of 2017, October 30 of 2019 and October 19, and only commercial pellet feed was provided. The amount of feed supplied varied depending on the culture period. For the first month after feeding, it was changed to fish feed fry (feed size, < 1 mm), No. 1 (1.0–1.1 mm), No. 2 (2.0–2.2 mm), and No. 3 (3.0–3.2 mm), weekly. After that, it was divided into No. 4 (4.0–4.3 mm) and No. 5 (5.0–5.3 mm) for approximately 45 days, and feed No. 6 (6.0–6.3 mm) was supplied until the feed supply was discontinued. The feed supply was provided twice daily (07:00 and 17:00) as a basic schedule, considering the weather conditions caused by rain or high water temperature. Feeding frequency was also determined based on the feeding rate of the catfish, which was sometimes reduced to once a day or omitted. Size-based screening was not conducted during the culture period.
The following parameters were monitored: the stocking periods, planting quantity, stocking quantity, stocking size, feed supply, disease management, stock density, and selling periods. A rearing diary was maintained by the fish farm manager after the daily feeding and cleaning of the farm for additional analysis.
The monthly growth performance of S. asotus was analyzed for 16 months by visiting the farm once a month between the 20th and 30th of each month during the monitoring period. During this period, 15–30 S. asotus individuals were randomly captured from the fish farm using a 7 × 7 mm throwing net. The captured S. asotus were anesthetized using an anesthetic (MS-222, Sigma-Aldrich, St. Louis, MO, USA) at a 100–200 ppm a concentration. The body weight was measured to the nearest 0.1 g using a digital scale (SW-1S, CAS, Seoul, Korea). Growth performance was analyzed by calculating the weight gain rate (WGR), specific growth rate (SGR), and feed coefficient rate (FCR) using a rearing diary from 2017 and measured values from 2018 to 2019.
To investigate the fish farm’s water quality, water temperature, pH, and dissolved oxygen (DO) were measured monthly at approximately 14:00 using a multi-point water quality meter (YSI-58, Yellow Spring, OH, USA) at the study site. Ammonia, nitrite nitrogen (NO2-N), and nitrate nitrogen (NO3-N) levels were measured using a simple water-quality kit (Merck KGaA, Darmstadt, Germany). Water quality was not measured when the farm was emptied of S. asotus for sale or maintenance purposes.
Results and Discussion
This monitoring study was conducted based on rearing diary data of catfish farm at the same farm site, including fry entry, feeding, culture methods, and selling markets. To enhance the manager’s scientific analysis of culture data, we conducted monthly field surveys on growth and water quality changes. Therefore, the results of this study are highly significant as a field-based research report based on three years of data on catfish farm, which is very rare in Korea. Three stockings and sales were recorded during the monitoring period. Field surveys and inspection of the rearing diaries were also conducted. The statuses of the farm are shown in Table 1.
According to the 2017 rearing diary, the culture period lasted 305 days from May 5, 2017 to March 6, 2018. Feeding was continued until October 11 (159 days). Nine sales totaling 27,000 kg occurred between January 2 and March 6, 2018. The 2018 culture period was monitored directly on the fish farm, and extended for 276 days from June 2, 2018 to March 5, 2019. Feeding occurred until October 30, 2018, with a culture period of 150 days. After wintering, 20,160 kg of fish were shipped between March 5 and 8, 2019. The 2019 culture period was extended for 166 days, from May 11 to October 19, 2019, and 30,000 kg of produce were shipped. The 3-year culture data confirmed that approximately 150–160 days were required to rear an S. asotus fish farm for shipping.
The establishment of aquaculture technology for all aspects of S. asotus production has enabled the production of fry, intermediate, and adult catfish. S. asotus fry production was performed in April using male and female adults that were reared indoors for approximately one month (NIFS, 2021). Typically, S. asotus fry are purchased or produced in April for sale, stocked in early May, and reared for approximately 5–6 months until the end of October. S. asotus aquaculture showed similar results during the monitoring (Lee & Kim, 2006). Moreover, this culture period was shorter compared with that of other inland aquaculture species such as eel (approximately 10 months–1.6 years), rainbow trout (approximately 1–2 years), muddy loach (approximately 1.2–1.6 years), bullhead catfish (approximately 2–3 years), and leather carp (approximately 1.6–2 years) (unpublished data). However, owing to the trend in the S. asotus industry, most sales are made at the end of October; hence, many S. asotus batches are retained until the following year and sold after the price trends are favorable (NIFS, 2021).
During our examinations in 2023, we confirmed that the farms were managed using the same methods. The facilities and culture methods have remained similar for the past four years. The stock density was adjusted annually, taking into account price and production. It can be inferred that catfish farming has an unstable industrial environment, which is applicable to the entire domestic aquaculture industry, including leather carp, muddy loach, and bullhead catfish (Lee & Kim, 2006). The Korean government established the 4th basic plan for the Promotion of Inland Fisheries from 2017 to 2021 and the 5th plan from 2022 to 2026 (MOF, 2022). However, more field-based policies need to be transformed to ensure eco-friendliness by considering aquaculture system diversity (Kim, 2008; Lee, 2009).
The most important factors for increasing profits in the aquaculture industry are operating costs and production and selling prices (Paek & Park, 2016). The domestic catfish farming industry had a stable fry supply but was severely negatively affected by the malachite green crisis in 2005. Catfish is an easy-to-breed species with a well-established consumer market, and production has increased overall. However, its production and price are unstable (KOSIS, 2022; Fig. 1). To overcome this issue and develop a sustainable catfish aquaculture industry, advanced aquaculture technologies such as bioflocs technology (BFT), recirculating aquaculture systems (RAS), smart aquaculture, and eco-friendly aquaculture technologies with aquaponics and Integrated Multi-Trophic Aquaculture (IMTA) need to be applied (Hwang et al., 2021; Kim et al., 2019a, 2022; Lee et al., 2019). In addition, it has been reported that catfish grow well and support the possibility of BFT and aquaponic systems (Kim et al., 2019b). Catfish’s growth and survival rates were more than 10% and 5% higher, respectively, in the BFT experimental group than in the flow-through system group (Park et al., 2021).
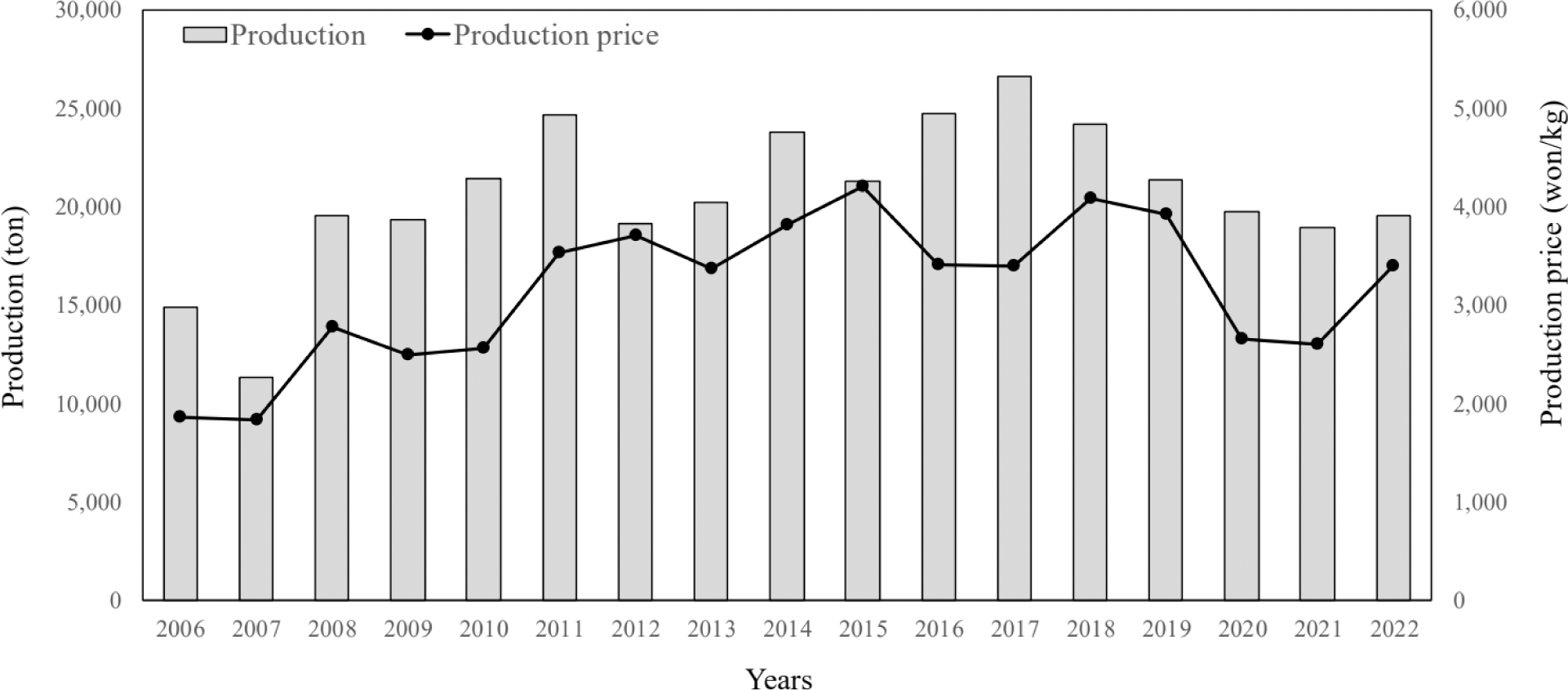
Competitiveness in the aquaculture industry can be improved by increasing the value of catfish and controlling the timing of the selling period. However, an economic analysis could not be conducted in this study because the catfish farm manager did not consent to the analysis for personal reasons. Monitoring and analysis are required to accumulate basic data from different sites for policy establishment and aquaculture technology development.
The growth performance results of the monitoring survey from 2017 to 2019 are presented in Table 1 and Figs. 2 and 3.
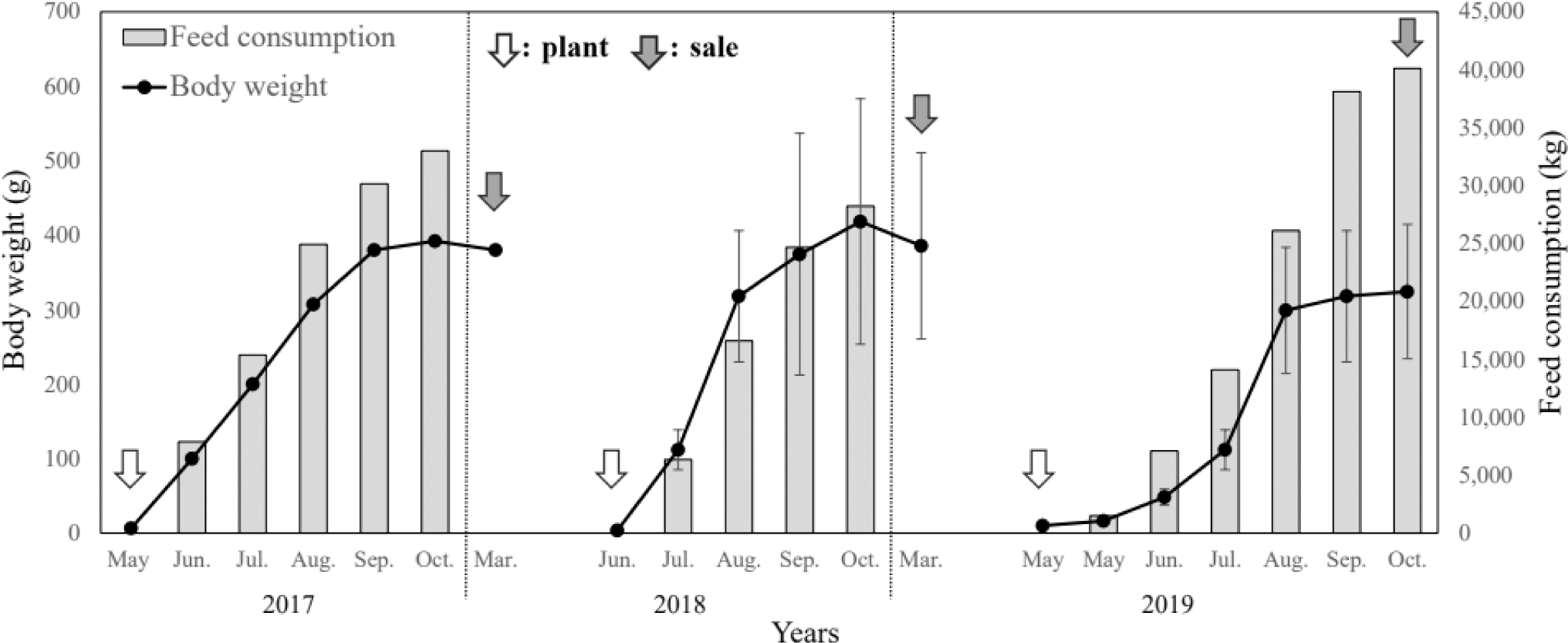
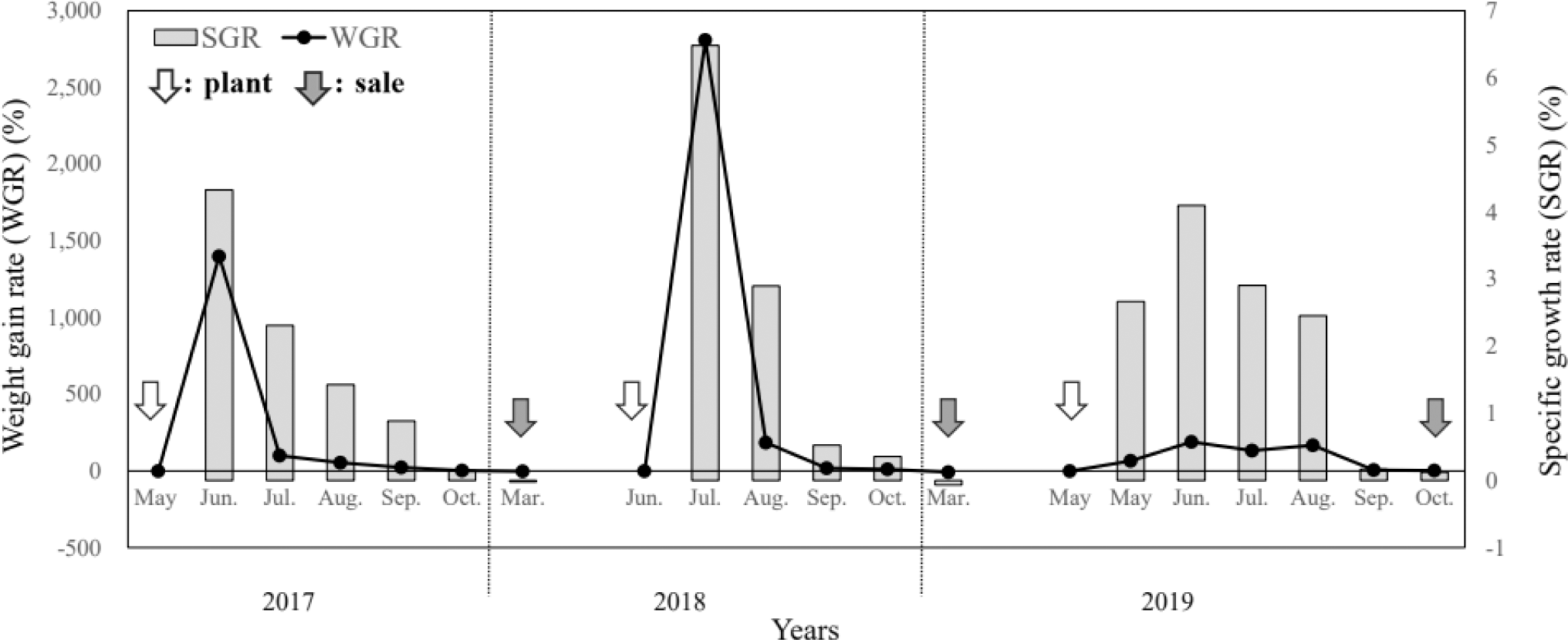
In May 2017, S. asotus (body weight, 6.67 g) was stocked and grew to 100 g in June, showing the highest growth rate with a monthly WGR of 1,399.2% and SGR of 4.3%; by October the weight was 392.5 g. During the 2017 culture period, the WGR was 4,664.7%, the SGR was 1.27% for the culture period and 2.43% for the feeding period, the FCR was 1.25, and the stocking density increased from 0.17 kg/m2 at entry to 8.17 kg/m2 at sale.
In 2018, S. asotus was stocked at an initial weight of 3.85 g in June and 112.0 ± 26.8 g in July; the monthly WGR was 2,809.1% and the SGR was 6.5%, which was estimated to be the fastest growth during the study period. The survey in October showed that the weight increased to 418.3 ± 164.8 g but the growth rate showed. Feeding was stopped during the winter months from November 2018 to March 2019, and the S. asotus weighed 385.7 ± 125.1 g in March 2019 (WGR of –7.8% and SGR of –0.1% compared with the weight before feeding was stopped), indicating a decrease in body weight. In 2018, WGR was 6,452.0%, SGR was 1.52% for the culture period and 2.79% for the feeding period, FCR was 1.42, and stocking density increased from 0.09 kg/m2 at entry to 6.10 kg/m2 at sale.
In 2019, S. asotus were stocked in May with an initial weight of 10.0 g. The highest growth rate during the study period was observed in June with a monthly WGR of 190.4% and SGR of 4.1%. During the 2019 culture period, the WGR was 3,270.0%, the SGR was 1.11% for the culture period and 2.12% for the feeding period, the FCR was 1.52, and stocking density increased from 0.26 kg/m2 at entry to 9.08 kg/m2 at sale.
Starvation experiments have shown that the catfish have showed reduced body weight and growth, decreased immunity, and anorexia; similar results have been reported in flatfish (Lee et al., 2008; Park et al., 2006). Low water temperatures cause reduced growth in fish owing to decreased digestive enzyme activity and metabolic rates (Fauconneau et al., 1983). In the present study, body weight loss was caused by low temperatures during winter. Outdoor monitoring results from muddy loach farms showed a weight loss of approximately 56% during winter stocking (November–March) (Choi et al., 2020). As this is expected to reduce in total catfish production in the future, the nutritional status and physiological changes in catfish caused by starvation at low water temperatures need to be studied.
As S. asotus on the farm was stocked at different stocking densities at the surveyed aquaculture sites from 2017 to 2019, the monitoring experiment could not be replicated; hence, distinguishing statistical differences between the results from years at a significant level was impossible. However, the fastest growth occurred from June to August, and this trend was observed during all the three years (2017–2019). These results also suggest that feed intake, WGR, SGR, and FCR varied and that total production was a function of stocking density. In particular, a high stocking density could reduce access to feed, reducing intake opportunities, resulting in a sustained decline in growth (Rowland et al., 2006). Consequently, further research should determine the appropriate densities for various aquaculture fish species and rearing environments.
Optimal stocking density varies among species (Lim et al., 2021). Kim et al. (2005) reported that small flatfish exhibited growth and high survival rates without large flatfish. In addition, selecting the optimal stocking density is considered particularly important. For example, the indoor culture of eels requires a regular screening process every 2–3 months to ensure efficient growth (NIFS, 2009). The monitoring results from 2017 to 2019 showed that the FCRs were 1.25, 1.42, and 1.38, and the stocking densities were 0.17 kg/m2, 0.09 kg/m2, and 0.26 kg/m2 in 2017, 2018, and 2019, respectively. This indicates that the feeding efficiency of catfish at a medium stocking density was highest in 2017. This difference may be attributed to differences in stocking density, as the catfish were reared by the same manager on the same fish farm in a similar water quality environment. Fish were fed commercial feed ranging in size from 1 to 6 depending on the rearing period, and the growth rate and feed efficiency increased with increasing feed size in flatfish experiments (Heo, 2011; Kim et al., 2019b). Therefore, judiciously increasing the feed size increases growth and feed efficiency.
Water quality parameters of the S. asotus farm under outdoor conditions are listed in Table 2. The results were similar for 2018 and 2019. Water temperature is the most important factor in aquaculture because it affects fish growth (Iwata et al., 1994). However, the water temperature is highly variable in outdoor aquaculture because of seasonal effects. Several studies have reported weight loss and increased mortality owing to high temperatures (Choi et al., 2011; Kim & Lee, 2017; McCarthy et al., 1998). In this study, high temperatures above 30°C were observed in July and August 2018 and 2019 during the survey period; however, no mass mortality was observed.
During the monitoring period, the fish farm was prepared for mortality due to a rapid DO decrease and deterioration in water quality due to fasting, reduced feeding, and increased groundwater circulation. In general, outdoor aquaculture relies on feeding and water reflux to maintain the rearing environment; hence, the risk of growth reduction and death is always present owing to sudden environmental changes. In particular, changes in water quality must be managed because ammonia, nitrite nitrogen (NO2-N) are acutely toxic to fish. They increase the methemoglobin levels in fish blood, leading to respiratory distress or toxicosis (Kroupová et al., 2018; Lewis & Morris, 1986). Disease-related infections caused by introducing diseases are among the primary causes of mortality in aquaculture. Damage caused by natural disasters, such as typhoons, also requires special attention as outdoor aquaculture farms are constantly exposed to these elements (Sohn et al., 2015).
Although there were no water quality data in 2017, we directly surveyed catfish farms in 2018 and 2019 to monitor changes in water quality, and the results are discussed in the paper. There are limitations to measuring changes in the water quality of fish farms monthly. Recently, we used to the Hobo data-logger device, which records real-time water quality and it can perform excellently in measuring water temperature, DO, and salinity. However, the performance of devices for measuring substances such as ammonia, nitrite nitrogen (NO2-N), and nitrate nitrogen (NO3-N) is unsatisfactory. These water quality measurement devices are necessary because they provide essential and continued useful data for long-term monitoring research (Guragai et al., 2018).