Introduction
Thailand is one of the world’s largest producers and exporters of canned tuna products, with a total production of 445,241 tons worth 56,187 million baht in 2021 (Department of Fisheries, 2022). Skipjack tuna (Katsuwonus pelamis) is the primary commercial tuna species for canned tuna production, accounting for more than 70% of total imported tuna (Department of Fisheries, 2022). Large quantities of by-products (50%–55% of total weight) are generated from tuna processing and include head, skin, bone, viscera, washing water, blood and roe (Klaypradit et al., 2021), so maximal utilization of these materials will reduce waste and also promote economic sustainability.
Tuna roe accounts for 1–3 percent of the fish total weight (Klomklao et al., 2014). Being nutritious and high in amino acids, it could be used as an ingredient in human food products (Yoon et al., 2019). For example, it provides functional protein properties such as emulsifying agent, foaming ability (Park et al., 2016), antioxidant (Intarasirisawat et al., 2014) and proteinase inhibitor (Klomklao et al., 2016). Additionally, free glutamic acid, the most prevalent amino acid in fish roe, is also reported as the main attributor of imparting an “umami taste” (Tavakoli et al., 2021). This finding is consistent with Kocatepe et al. (2019) who demonstrated that fish roe from whiting (Merlangius merlangus euxinus Nordmann 1840) contained high levels of glutamic acid (max 2,232.0 mg/kg), aspartic acid (max 2,393.5 mg/kg), and lysine (max 1,894.8 mg/kg), which are associated with umami taste. The use of umami compounds naturally present in fish roe as taste enhancers can be a potential ingredient to be applied in the food industry. Normally, there are two different types of roe found in skipjack tuna, unofficially called “red roe” and “yellow roe” according to the external color. However, to our knowledge there is no published report of how these two forms differ in terms of nutritional composition, chemical and physical properties, developmental state and equivalent umami concentrations (EUC).
Most functional ingredients are preferred in a dry powder form to facilitate their use and to ensure long and stable storage. Changes in chemical and physical properties of powders have been found to result from sample preparation protocols and drying methods. Lee et al. (2016) reported that protein concentrate from yellowfin tuna roes prepared by two drying methods (freeze-drying [FD] and drying in an incubator at 70 ± 1°C for 15 h) contained high levels of protein, with aspartic acid, glutamic acid and leucine as major amino acids. Park et al. (2016) also showed that tuna roe protein concentrates prepared by FD had high protein functionality, with protein solubility, foaming capacity and oil-in-water emulsifying activity higher than in protein concentrates prepared by incubator (70 ± 1°C for 15 h).
In the present study, therefore, we analyze differences in histology of red and yellow tuna roes. We also examine the influence of three drying methods (hot-air drying [HD], vacuum-drying [VD] and FD) on chemical and physical properties of the dried product that may affect its nutritional value and also analyze of EUC as potential food taste enhancer.
Materials and Methods
Methanol and water (high-performance liquid chromatography [HPLC] grade) were purchased from B&J Honeywell (Muskegon, MI, USA); sodium lauryl sulphate (SDS) and ammonium from Kemaus (Cherrybrook, Australia); 2-mercaptoethanol from Acros (Geel, Belgium); N,N'-methylene-bis-acrylamide, N,N,N',N'-tetramethylethylenediamide from Bio-Rad Lab (Hercules, CA, USA); amino acid standard, amino acid supplement, o-phthalaldehyde and 3-mercaptopropionic acid from Agilent (Santa Clara, CA, USA). All other chemicals and reagents were of analytical reagent grade and commercially available.
Immature (yellow) and mature (red) skipjack tuna (K. pelamis) roes (Fig. 1) were received from a tuna cannery in Samutsakorn province, Thailand. The roes were transported to a laboratory at the Department of Fishery Products, Faculty of Fisheries, Kasetsart University within 2 h. The roes were cleaned of any remaining residues using running tap water. For the histological study, roes were cut into small pieces (5 × 5 cm) using a sharp knife, and later ground using a blender before drying. The obtained ground roes were packed in polyethylene bags and stored at −20°C until use.
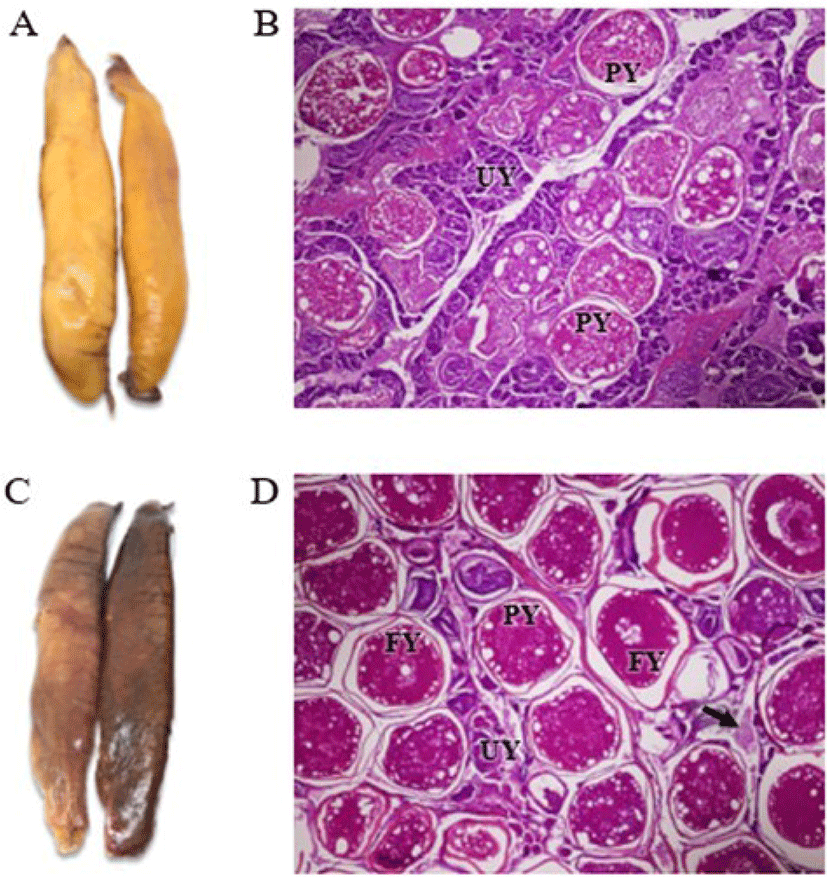
Histological study of fresh roes followed the method of Bell & Lightner (1988). Pieces of roe were fixed with 10% buffer formalin with a sample/fixative ratio of 1:20 at 37°C for 24–72 h. The fixed samples were cut into the size of 0.5 cm cubes and put into an embedding cassette, then dehydrated using an automatic tissue processer (TP1020, Leica, Buffalo Grove, IL, USA). Paraffin embedding was applied to harden the tissues. Micro-tome (RM2235, Leica) was used to slice tissues to a thickness of 5 μm, then floated on warm water. The samples were further dried using a warmer plate at 50°C for 2 h, and then stained with hematoxylin and eosin (H&E staining). The histological structure of the prepared roes was observed using light microscope (Olympus, BX51TF, Tokyo, Japan).
The frozen ground roes were thawed using running tap water and dried using three different methods: 1) HD using hot air dryer (UN110, Memmert, Germany) at 70 ± 1°C for 30 h; 2) VD using vacuum dryer (VO101-thermoblech, Memmert) at 40 ± 1°C, 72 mbar for 21 h; and 3) FD using freeze dryer (Coolsafe 95-15, Labogene, Lillerød, Denmark), where ground roes were pre-frozen at −20 ± 1°C and then freeze-dried under vacuum at –40 ± 1°C for 18 h. The dried roes were ground into fine powder and kept in glass bottles at ambient temperature for further analysis.
The dried roe samples were analyzed for proximate composition including moisture, protein, fat and ash contents following the Latimer (2016).
The water activity (aw) value of all dried roes was determined using water activity meter (4TEV, Aqualab, Pullman, WA, USA).
The color of dried samples was determined by colorimeter (Ultrascan VIS, HunterLab, Reston, VA, USA). The CIELAB system was used to quantify the color of the samples: L* is the brightness value between 0–100; positive a* values indicate redness and negative a* values indicate greenness; positive b* values indicate yellowness and negative b* values indicate blueness.
Oils of ground fresh roe and dried roe samples were extracted according to the method of Bligh & Dyer (1959) before further analysis for fatty acid composition. Samples (50 g) were homogenized with 100 mL of a mixture of chloroform:methanol (1:1, v/v) at 10,000 rpm using homogenizer (T25D, IKA, Staufen, Germany) for 1 min. The homogenates were then combined with 50 mL of chloroform. The mixture was filtered through Whatman No. 4 filter paper (Whatman International, Maid-stone, UK). The filtrate was transferred into a separating funnel. The bottom phase (chloroform phase) was drained off into an Erlenmeyer flask and sodium sulphate (1–2 g) was added. Oils in the chloroform phase were decanted into a round-bottom flask and then evaporated at 40°C using rotary evaporator (R-124S, Buchi, Sankt Gallen, Switzerland). The oils were transferred into an aluminum bottle and kept under nitrogen at −20°C until use.
The obtained oils were prepared as fatty acid methyl esters (FAME) using methyl-tricosanoate (internal standard) according to method Ce 1b-89 of AOCS (1992). Oil samples (25 mg) were mixed with 1.5 mL of 0.5 M methanolic NaOH then boiled at 100°C for 5 min using water bath (WNB22, Memmert). Borontrifluoride (2 mL) was added into the oil then boiled at 100°C for 5 min. The oil was added with 1 mL of isooctane and 5 mL of saturated sodium chloride. The obtained FAME (1.0 μL) were injected into GC (7890A, Agilent) equipped with HP-88 capillary column (100 m × 0.25 mm, 0.20 μm). The initial temperature of the oven was 140°C and increased to 240°C at a rate of 4°C/min, injection at 260°C and flame ionization detector at 280°C using flow rate 1.0 mL/min. The fatty acid profile was calculated with peak of polyunsaturated fatty acid (PUFA) No 3 from menhaden oil standard and reported as percentage of total of fatty acid.
Quantities of 0.5 g of ground fresh roe and dried roe samples were hydrolyzed with 5 mL of 6 M HCl (for amino acid profile study) or with 4.2 M NaOH (for tryptophan study) using heating bath (B-300, Buchi) at 110°C for 24 h. The hydrolyzed roes were diluted with 50 mL of water (HPLC grade), filtered through a 0.22-μm nylon membrane filter, and then 1 μL of filtrate was used for injection. Each amino acid was derivatized with o-phthaldialdehyde (OPA) and determined by HPLC (1200 infinity series, Agilent) equipped with poroshell-120 column (HPH-C18, 4.6 × 100 mm, 2.7 μm) at 40°C and detected for excitation at 230 nm and emission at 450 nm using fluorescence detection.
A reversed-phase HPLC method was developed for separation of amino acids. Mobile phase A consisted of 10 mM di-sodium hydrogen phosphate (Na2HPO4), 10 mM sodium tetra-borate (Na2B4O7 · 10H2O) and 0.5 mM sodium azide (NaN3), where pH was adjusted to 8.2 with concentrated HCl. Mobile phase B consisted of acetonitrile, methanol and water in ratio (45:45:10 [v/v/v]). Gradient conditions were as follows: 0–0.35 min 2% B, 13.4 min 57% B, 13.5–15.7 min 100% B and 15.8-18.0 min 2% B, and the flow rate was 1 mL/min. The amino acid quantities were expressed as g/100 g of protein.
Free amino acid (FAA) study of dried roe samples followed the method of Boone & Claybrook (1977). Quantities of 50 mg of dried roe samples were mixed with 0.5 mL of 10% trichloroacetic acid then homogenized and centrifuged at 10,000×g for 15 min at 4°C. Supernatants were filtered through a 0.22-μm nylon membrane filter, and then 1 μL of filtrate was used for injection. FAA was derivatized with OPA and determined by HPLC (1200 infinity series) equipped with poroshell-120 column (HPH-C18, 4.6 × 100 mm, 2.7 μm). The conditions used according to the study of amino acid profile.
Sample preparation for 5'-nucleotides analysis following modified method from Hwang et al. (2000). Dried roe powder (0.01 g) was homogenized with 5 mL of 7.5% perchloric acid (PCA) using homogenizer (T25D) at 4,000 rpm for 2 min and centrifuged using a centrifuge (UNIVERSAL 32 R, Hettich, Tuttlingen, Germany) at 10,000×g, 4°C for 5 min. The supernatants were filtered through Whatman No. 1 filter paper (Whatman International) then mixed with neutralizing PCA buffer pH 7.6 as a ratio 1:1 (v/v) and filtered through 0.22-μm syringe filter and then 5 μL of filtrate was used for injection. Each 5'-nucleotide was determined by HPLC (1200 infinity series) equipped with supelco column (SUPELCOSIL LC18-1, 25 cm × 4.6 mm, 5 μm) and detected at 254 nm.
A reversed-phase HPLC method was developed for separation of amino acids. Mobile phase A consisted of 0.1 M potassium dihydrogenphosphate (KH2PO4) and 4 Mm tetrabutylammonium hydrogen sulfate, where pH was adjusted to 6.5. Mobile phase B consisted of mobile phase A: methanol as ratio 70:30 (v/v), the flow rate was 1 mL/min and column temperature was ambient 24°C–26°C. The 5'-nucleotide quantities were calculated by comparing the area peak of the sample with the standard.
EUC is the concentration of monosodium glutamate (MSG) (g/100 g sample) equivalent to the umami taste by relation of the umami amino acids (Glu and Asp) and umami 5'-nucleotides (5'-inosine monophosphate [5'-IMP] and 5'-guanosine monophosphate [5'-GMP]), which is calculated according to Equation (1) of Yamaguchi et al. (1971).
where Y is the EUC of the sample (g MSG/100 g); ai is the concentration (g/100 g) of each umami amino acid (Glu or Asp); aj is the concentration (g/100 g) of each umami 5'-nucleotide (IMP, GMP); bi is the relative umami concentration (RUC) for each umami amino acid in MSG (Glu, 1; Asp, 0.077); bj is the RUC for each umami 5'-nucleotide (IMP, 1; GMP, 2.3); and 1,218 is the synergistic constant based on the concentration of g/100 g used.
The protein patterns of ground fresh roe and dried roe samples were determined by sodium dodecyl sulfate polyacrylamide gel electrophoresis (SDS-PAGE). It was operated following the method of Laemmli (1970). Dried roe (50 mg) was dissolved in 1 mL of mixture of 8 M urea solution containing 2% SDS and 2% mercaptoethanol. Sample solution was mixed with SDS-PAGE sample buffer (0.5 M Tris-HCl [pH 6.8]) at ratio of 4:2 (v/ v), then boiled at 100°C for 3 min. The sample (20 μg protein) was loaded onto 4% stacking gel and 12% separating gel and electrophoresed at a constant voltage (90 V) using mini-protean cell (Bio-Rad Lab). After separation, electrophoresed gel was stained in 0.01% coomassie brilliant blue R-250 in methanol solution containing acetic acid and water at ratio of 4:1:5 (v/v) for 3 h and destained with 40% methanol and 10% acetic acid (v/v) for 12 h. The molecular weights of protein bands were determined using precision plus protein™ standards (10–240 K, BioRad Lab).
The experiments were conducted at least three times and values are shown as mean and SD. We used one-way analysis of variance and Duncan’s multiple range test (p < 0.05) to identify significant differences among treatments, using the statistical software SPSS (SPSS ver. 23, IBM, Armonk, NY, USA).
Results and Discussion
Fig. 1A and 1C show the external appearance of immature and mature skipjack tuna roe sacs, with the most apparent difference between them being color (yellow vs. orange-red). The difference might be attributed to the presence of blood vessels in the membrane, as indicated by Chodrijah et al. (2020), who reported that the orange-pink skipjack tuna ovary has conspicuous superficial blood vessels. Typically, oocytes can be found in many stages within the ovary. Histological study revealed that yellow roe (Fig. 1B) contained mostly small oocytes stained purple-blue by hematoxylin, whereas red roe (Fig. 1D) contained mostly large oocytes stained pinkish-red by eosin. These results supported Itano (2000), who found that immature roe of yellowfin tuna contained closely packed oocytes stained with hematoxylin, and that mature roe contained fully yolked oocytes (FY), indicating that the fish was ready for spawning. Moreover, unyolked oocytes (UY) and partially yolked oocytes (PY) were the most common types detected in yellow roe (Fig. 1B). FY and PY were the most common types found in red roe (Fig. 1D) and post-ovulatory follicles (arrow) were also observed. Itano (2000) similarly found that immature roe commonly contained PY, whereas mature roe mainly contained FY as well as some UY or PY. Therefore, our histological examination of yellow and red roes of skipjack tuna verified that these are immature roe and mature roe, respectively.
Tuna roes are dried to increase their shelf life by reducing the water content and aw, and also to eliminate the need for low temperature during transport and storage. However, the physical-chemical and nutritional properties of dried foods might be affected by the drying methods. Tuna roe powders were obtained by three different drying methods, namely HD, VD and FD. Analytical results (Table 1) indicate that all tuna roe powders had low moisture content (5.10%–6.59%) and aw (0.38–0.62), and met national standards for powdered products (Thai Industrial Standards Institute, 2014) to ensure that chemical reactions are reduced and bacterial growth is inhibited. It was found that protein was the main component of dried mature and immature roes, with ranges of 69.32%–70.15% and 69.31%–70.55%, respectively. However, the protein content did not differ (p > 0.05) between mature and immature roes when using the same drying method. Interestingly, lipid content of immature roe was higher (p < 0.05) than that of mature roe. This difference might be explained according to the study of Bik et al. (2020) who indicated that lipid is necessary as an energy source for the development and organogenesis of embryos; Bledsoe et al. (2003) also reported that mature roe has an increased ratio of moisture to lipid contents. By contrast, the carbohydrate in immature roe was lower than in mature roe. Ash content was in the range of 4.13%–5.27% and 4.16%–5.14% for immature and mature roes, respectively. These results resemble those of Lee et al. (2016), who reported that dried yellowfin tuna contained moisture, protein, fat and ash contents of 4.3%–5.6%, 72.3%–77.3%, 10.6%–11.3%, and 4.3%–5.7%, respectively. Similarly, Yoon et al. (2018) reported that dried (at 70 ± 1°C for 15 h using an incubator) skipjack tuna roe had moisture, protein, fat and ash contents of 5.6%–6.5%, 73.0%–76.0%, 12.7%–15.6%, and 5.2%–5.4%, respectively. Al-Sayed Mahmoud et al. (2008) explained that differences in proximate composition of fish roes depends on many factors, including species, maturity stage, diet, season, and processing conditions.
Color is an important aspect of dried products, as it indicates quality and consumer acceptance (Selani et al., 2016). The appearance of the powders is shown in Fig. 2, which corresponds to the color parameters in Table 1. The method used to dry the roes was found to significantly (p < 0.05) affect the color parameters (L*, a*, and b*). HD and VD caused higher redness (a*) and yellowness (b*) but lower lightness (L*) for both mature and immature roes compared to FD. The color values found in this study are in accordance with Sathivel et al. (2009), who prepared catfish roe protein powder using a spray-dryer, and reported L*, a*, and b* values of 71.7, 3.9 and 28.8, respectively. FD with low temperature seems to prevent color changes of dried roes.
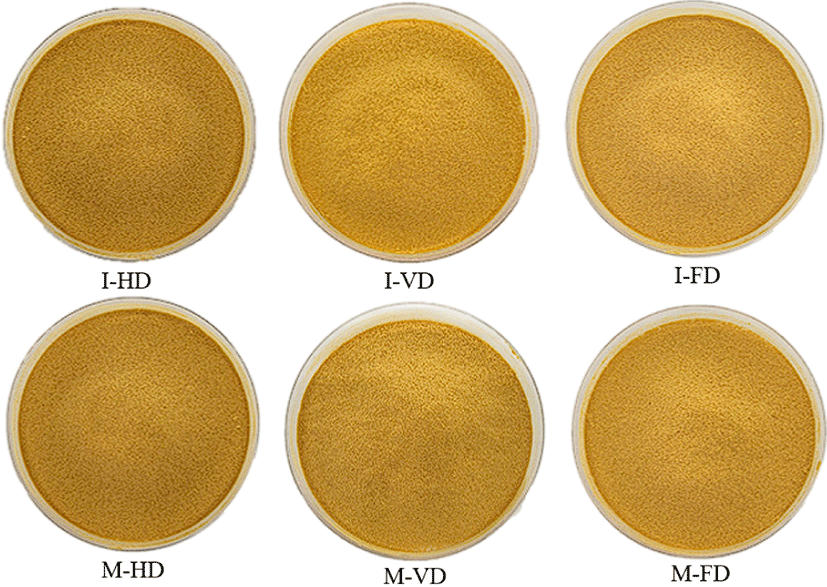
The fatty acid composition of fresh and dried tuna roes is shown in Table 2. The raw material of both mature and immature roes had high PUFA (42.21%–43.50% of total fatty acid), followed by saturated fatty acid (SFA) (35.90%–38.51%) and monounsaturated fatty acid (MUFA) (19.28%–20.60%). In both raw and dried roes, palmitic acid (C16:0) and oleic acid (C18:1) were the major SFA and MUFA, respectively. Huynh et al. (2007) explained that palmitic acid acts as an energy source for fish metabolism during maturation and especially during roe formation in female fish, while oleic acid plays a role in metabolic processes as absorbed energy during the development of the reproductive organs and also is important for ovarian development and fish growth. Moreover, raw and dried tuna roes were high in n-3 PUFA (34.76%–43.50%). Interestingly, docosahexaenoic acid (DHA; C22.6n3), an omega-3 fatty acid, was found as the most abundant PUFA. Tocher (2010) indicated that n-3 PUFA was generally present in the roe of marine species. Rao et al. (2010) also reported that DHA is important in healthy development of retina and brain tissues of fish larvae. Our results were in agreement with Ahmmed et al. (2021a, 2021b) who reported on fatty acids in roe of salmon and mackerel, and found higher DHA (34.47% and 27.5% of total fatty acid, respectively) than eicosapentaenoic acid (EPA; C20.5n3) (7.99% and 11.3%). Intarasirisawat et al. (2011) also found high DHA content in the roes of skipjack, tongol and bonito tunas (23.46%, 26.19%, and 20.53% of total fatty acid, respectively), and lower content of EPA (3.80%, 4.62%, and 4.62% of total fatty acid). Therefore, both dried mature and immature skipjack-tuna roes could be used as a functional ingredient acting as a good source of DHA, which plays an important role in supporting growth and development of the brain, learning ability, restoration of eyes from abnormalities (Horrocks & Yeo, 1999; Klaypradit & Huang, 2008).
I, raw immature roe (yellow); M, raw mature roe (red); I-HD, immature roe dried by hot air oven; I-VD, immature roe dried by vacuum oven; I-FD, immature roe dried by freeze dryer; M-HD, mature roe dried by hot air oven; M-VD, mature roe dried by vacuum oven; M-FD, mature roe dried by freeze dryer; SFA, sum saturated fatty acids; MUFA, sum monounsaturated fatty acid; EPA, eicosapentaenoic acid; DHA, docosahexaenoic acid; PUFA, sum polyunsaturated fatty acid.
The amino acid profiles of raw and dried tuna roes are presented in Table 3. Differences in amino acid content of raw material might be due to the development stage of tuna roe, as previously described for the histological study. Glutamic acid was found as a major amino acid in both fresh immature and mature skip-jack tuna roe (12.81 and 14.08 g/100 g protein), respectively. Proline, cysteine, valine, and isoleucine contents decreased (p < 0.05) while other amino acids were increased with the maturity stage of roe. The differences of amino acid between immature and mature roes might be explained according to the study of Bekhit et al. (2009), who indicated that differences in amino acids of salmon roe depends mainly on the stage maturity and V’Uorela et al. (1979) also reported that an increase or decrease of amino acids with various stage maturity do occur without consistent trends. These results are supported by the study of Sivaloganathan et al. (1998), who determined that FAAs played an important role as an energy substrate for developing roes and larvae and during embryonic stages, but were less important after hatching.
The dried tuna roes contained the following major amino acids: glutamic acid (13.58–14.61 g/100 g of protein), leucine (8.06–8.42), aspartic acid (7.81–8.39), alanine (6.63–6.89), and lysine (6.06–6.89). Yoon et al. (2018) also studied skipjack tuna roe (dried at 70 ± 1°C for 15 h using an incubator) and found comparable levels of glutamic acid (12.7–12.8 g/100 g of protein), aspartic acid (8.8–9.1), alanine (6.7–7.0), leucine (8.5–8.6) and lysine (8.1–8.4). Lee et al. (2016) prepared dried yellowfin tuna roe using freeze dryer and incubator, and reported finding major amino acids as glutamic acid and aspartic acid (13.1–13.2, 8.7–9.2 g/100 g of protein, respectively). However, a different suite of dominant amino acids was reported by Ziaeian et al. (2008) for longtail tuna roe included histidine, leucine and ly-sine (10.80, 10.30, and 11.70 g/100 g of protein), respectively. The difference in amino acid content among tuna roes might be due to differences in habitat, feeding and season (Intarasirisawat et al., 2011). The dried mature roe and immature roe are good sources of protein containing essential amino acids. Dried tuna roes with their high levels of glutamic and aspartic acid are expected to be applicable as a food enhancer. Umami compounds can play an important role in food processing, where they are used for enhancing food flavor (Harada-Padermo et al., 2020; Poojary et al., 2017). The umami taste is mainly attributed to the presence of glutamic and aspartic acid (Dermiki et al., 2013).
FAAs are important contributors to the taste of seafood. Specially, glutamic and aspartic acids were MSG-like components, which play an important role in the umami or palatable taste (Kawai et al., 2012; Yamaguchi et al., 1971). Moreover, the 5'-nucleotides, including 5'-GMP and 5'-IMP had influence on taste enhancing in seafood by synergistic effect with glutamic acid on enhancing umami taste sensation (Bachmanov, 2010; Poojary et al., 2017; Yin et al., 2019). As for study of the synergistic effect of MSG-like FAAs and 5'-nucleotides might increase the umami taste was expressed as EUC. In this study, the effect of different drying methods on umami amino acids, 5'-nucleotide and EUC of immature and mature roes are presented in Table 4. Umami amino acids (L-Glutamic and L-Aspartic) of dried immature and mature roes were higher in HD than was observed in VD and FD method (p < 0.05). This might be related to the use high temperatures resulting in proteolysis promoted during drying process. The results were similar to those of Harada-Padermo et al. (2020) who reported that the MSG-like amino acids, L-Glutamic and L-Aspartic, were found in greater amount in the hot-air dried shiitake stipes (2.68 and 0.39 mg/g of dry sample, respectively). Furthermore, Zhao et al. (2020) also demonstrated that total umami amino acids (L-Glutamic and L-Aspartic) of first grade of wild mushroom (Suillus granulatus) in HD samples were the highest compared with VD and FD samples. This result indicates that drying the immature and mature tuna roes by the conventional method (HD) allows greater extraction of umami compounds. For the different types of skipjack tuna roe, the mature roe dried by hot air oven exhibited umami amino acids higher than the immature roe. This may have been due to the content of FAAs was related to the amount of FAA generation from protein degradation through the Maillard reaction (Eissa et al., 2009).
I-HD, immature roe dried by hot air oven; I-VD, immature roe dried by vacuum oven; I-FD, immature roe dried by freeze dryer; M-HD, mature roe dried by hot air oven; M-VD, mature roe dried by vacuum oven; M-FD, mature roe dried by freeze dryer, 5’-GMP, 5’-Guanosine monophosphate; 5’-IMP, 5’-Inosine monophosphate; EUC, equivalent umami concentration, MSG, monosodium glutamate.
With respect to the umami 5'-nucleotide, it was found that 5'-GMP and 5'-IMP contents demonstrated in the range of 2.91–3.01 and 1.73–1.80 g/100 g sample of immature roe powders and 3.34–3.46 and 2.09–2.21 g/100 g sample of mature roe powder (Table 4). The content of 5'-GMP and 5'-IMP found in this study was higher than those study of Zhao et al. (2020) who indicated the content of 5'-GMP and 5'-IMP in the range of 0.05–1.39 and 0.02–1.30 mg/g of dry weight, respectively. This suggested that nucleotides are not easy to be destroyed and relatively stable in the conventional drying process (Wu et al., 2015). Among the skipjack tuna roes, all mature roe powders obtained from different drying methods had higher amount of umami nucleotides than the immature roe powders.
The synergistic effect of MSG-like components with umami amino acid and umami nucleotides greatly increased the umami taste and this relation was evaluated by the EUC values (Table 4). The content of EUC in dried mature roe was higher than that observed in the dried immature roes. The EUC value of mature roe powder (73.09 g MSG/100 g of sample) obtained from HD was significantly (p < 0.05) higher than other samples. This result was in concordance with Harada-Padermo et al. (2020) who reported that the EUC of hot-air dried shiitake stipes was 81.55 g MSG/100 g of dry sample. Other than that, Hwang et al. (2020) showed that sous-vide cooking beef at 70< for 12 h gave the highest EUC at 53.29 g MSG/100 g compared to other conditions. This study revealed that dried immature and mature roes by the three drying methods contained umami compounds.
The SDS-PAGE patterns of raw and dried tuna roes are shown in Fig. 3. Raw and dried tuna roes presented main band around 97 kDa, which could indicate vitelline-like protein. Yoon et al. (2018); Intarasirisawat et al. (2011) reported that the protein with a molecular weight (MW) of 97 kDa might be a vitel-line-like protein in yellowfin, skipjack, tongol, and bonito tuna roes. Proteins in the range of 31–57 kDa were also observed in raw roes and dried roes that might be subunits of actin, troponin-T. However, the bands of mature roes were more pronounced than immature roes and the bands of dried roes were much darker than those found in raw roes. This observation was supported by Lee et al. (2016) who indicated that protein bands in MW ranges of 50–37 kDa and 15 kDa could be actin, troponin-T and myosin light chain in protein concentrates prepared from yellowfin tuna roe using either incubator (70°C, 15 h) or freeze dryer. Furthermore, proteins bands near 32.5 and 29 kDa have been found in different tuna roes those might represent ovomucoid or phosvitin (Al-Holy & Rasco, 2006; Losso et al., 1993). Proteins with low MW in the range of 15–18 kDa were found for all dried tuna roes but the bands were quite light, which assumed small amount of such protein presenting in the samples. Therefore, different electrophoretic patterns of dried tuna roes compared to raw roes indicating the difference in protein composition affected from drying process.
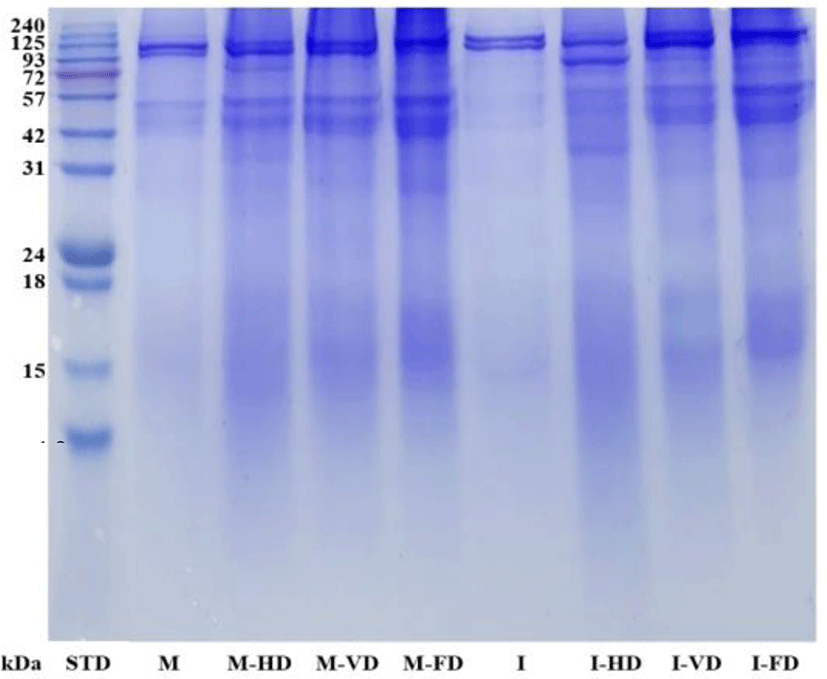
Conclusion
Yellow and red roes of Skipjack tuna were classified as immature and mature roes, respectively. The dried powders prepared from mature and immature roes using three different drying methods provided a good source of protein with abundant glutamic acid, leucine and aspartic acid, resulting in high EUC values. The protein band at molecular weight of 97 kDa (vitelline) indicated the major protein of these roes. All roe powders were also rich in DHA. Therefore, tuna roe powder shows good potential as a functional ingredient, source of protein with high DHA, to promote health and also as taste enhancer. Further study on other functional properties of the roe powders is recommended.