Introduction
In recent years, ready-to-cook products like fish burgers are produced commercially in different communities of the world. Fish products are a rich source of protein and polyunsaturated fatty acids (PUFAs) which play a key role in human health (Raeisi et al., 2019). Generally, due to the high percentage of unsaturated fatty acids, free amino acids, and water activity, they are more sensitive to oxidation which leads to deteriorating food quality, undesirable changes in organoleptic and nutritional value. Application of natural antioxidants has attracted more attention for inhibiting oxidation and increasing the shelf life of fish products (Avadí & Fréon, 2015). Nevertheless, the natural bioactive compounds are unstable and more sensitive to conditions as temperature, oxygen and light, pH and presence of other nutrients during storage and food processing (Hasani et al., 2018). The encapsulation of bioactive compounds is a useful strategy to overcome these limitations by improving the physical and chemical stability of the phenolic compound-controlled release and protecting these compounds from reaction with food components (Fang & Bhandari, 2010). Previous study (Gortzi et al., 2006) has also reported that encapsulation of bioactive compounds such as phenolic extracts can increase the antioxidant and antimicrobial activity of compounds and retain the stability of antioxidants and antimicrobials during long storage. Liposomal encapsulation systems (spherical bilayer vesicle) are considered as an effective and nontoxic method to provide higher encapsulation efficiency, superior bioavailability, and large-scale production with low cost for encapsulation of bioactive and unstable compounds (Shim et al., 2013).
Some studies showed nano biopolymers like chitosan can notably improve physicochemical stabilization due to covalently or non-covalently bounding (Tan et al., 2017). Phycobiliproteins are a group of colored proteins present commonly not only in cyanobacteria (blue-green algae) but also in red algae, cryptomonads, etc. They are extensively commercially used in foods, cosmetics, biotechnology, pharmacology and medicine. Phycobiliproteins have high potential pharmaceutical and biological properties. Phycobiliproteins are a group of colored proteins with biological activity that are commonly introduced in red algae and cyanobacteria (blue-green algae) etc. Phycobiliproteins are generally noticed as safe (GRAS) food used in food products (Fernandez-Rojas et al., 2014). The utilization of phycobiliproteins as natural functional food additives due to high potential pharmaceutical and biological properties have global demand in the aspect of the toxicity and carcinogenesis of the synthetic food colorants. Therefore, the objective of the present study was to extract phycobiliproteins from Gracilaria gracilis and incorporate nanoliposomes contain phycobiliproteins into common carp burgers. The physicochemical, antioxidant properties and the effect of nanoencapsulated phycobiliproteins on the quality of burgers, and the potential efficacy of the liposomal system in the development of its antimicrobial and antioxidant activity during refrigerated storage (4°C) were evaluated.
Materials and Methods
The red algae Gracilaria gracilis was purchased from the Iranian National Algae Culture Collection (Tehran, Iran). The sunflower oil (Oila oil company, Tehran, Iran) was procured at a local market. Soy lecithin and glycerol were purchased from Merck Company (Darmstadt, Germany). The coating materials were the chitosan with low molecular weight (75%–85% degree of deacetylation) (Sigma-Aldrich, St. Louis, MO, USA). All other chemicals and reagents were of analytical grade. Distilled and deionized water was used for the preparation of all solutions.
The dried Gracilaria gracilis was milled and then mixed with 0.05 M sodium phosphate buffer (pH 6.8) under continuous stirring. The algae cells were further broken using ultrasonic waves in the ice bath and then stirred continuously for 8 h at 4°C. Cell residue was removed by centrifugation (10,000×g, 15 min) and the supernatant was collected. Phycobiliproteins (PBPs) were separated from the supernatant ammonium sulfate precipitation in two steps. The supernatant was removed after the salting-in treatment of 20% saturation of ammonium sulfate at first. The solution was centrifuged at 10,000×g for 15 min and then the resulting supernatant was set to 45% saturation of ammonium sulfate. After centrifugation, the sedimentation between 20% and 45% saturation of ammonium sulfate was collected and dissolved in 100 mL sodium phosphate buffer (pH 6.8). The sample was lyophilized for future study.
The nano-liposomes containing macroalgae phycobiliprotein were prepared as slight modification according to Rasti et al. (2012) method. First, lecithin and sunflower oil was placed in a heating bath (30°C) to ensure dissolve lecithin in oil completely. Then, the phycobiliprotein was added to the lecithin-oil mixture during constant stirring on a hotplate. The solution was hydrated by adding deionized water and glycerol (final concentration 2%, v/v and preheated to 30°C) and then homogenized for 15 minutes. Uncoated liposomes and coated liposomes with chitosan (0.5%, 1%, and 1.5% w/v) are homogenized under high pressure (chitosan solution was prepared by dissolving chitosan in 1% v/v in acetic acid). To have the smaller particle mean size of the liposomes, the liposomal suspension was subjected to a sonication process in an ice bath (7 min, 1 s on and 1 s off) using a probe (Sonicator, 200 UPS, Dr. Hieschler, Teltow, Germany). Samples where are exposed to nitrogen gas and kept at room temperature for further analysis. Finally, lipid vesicles were obtained by freeze-drying (Fig. 1).
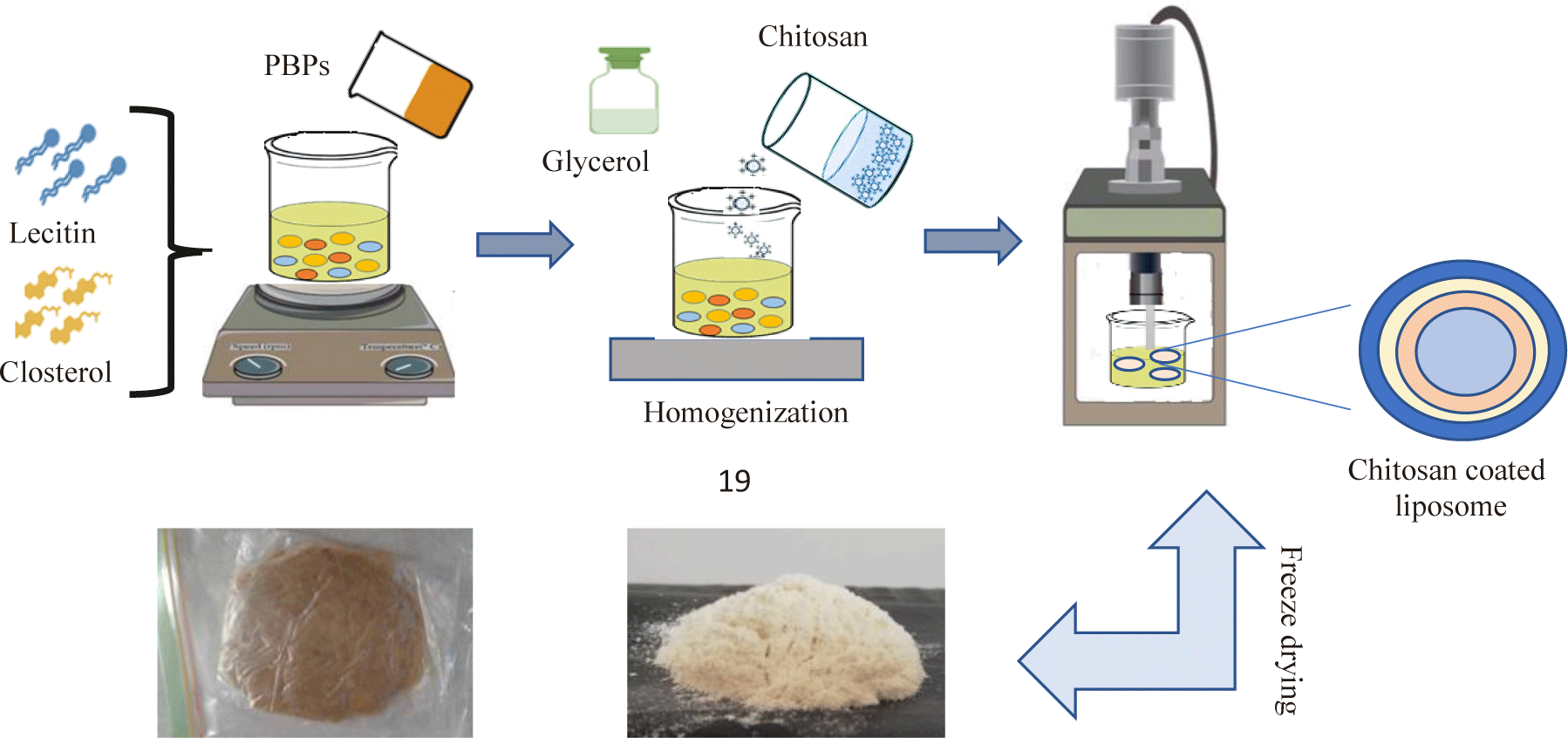
The hydrodynamic particle size and zeta potential of nano-capsules were determined by Malvern Zetasizer 3000HS (Malvern Instruments, Malvern, UK). Zeta potential provides information about the potential difference between the dispersion medium and the stationary layer of the fluid attached to the dispersed particle, which helps to know about the stability of the nano formulations. Measurements were made using aqueous diluted samples (2:1 ratio). Using the principle of photon correlation spectrometry, this instrument also gives the measurement of particle-size distributions in the range.
Encapsulation efficiency (EE) was obtained based on Fathi et al. (2013) method with slight modification. The filtration method using 50 cc filtered falcon tubes (Biologix, Jinan, China) will be used to determine the encapsulation efficiency of nanoliposomes containing phycobiliproteins. For this purpose, 1,000 μL of the nanoliposome is added to the upper part, then the tube is centrifuged at 12,000×g for 10 minutes. After centrifugation, the liquid passed through the filter is separated and the amount of adsorption is read by a spectrometer (6800 UV/VIS model, Jenway, Staffordshire, England) (Fathi et al., 2013). Percentage efficiency (EE) is calculated according to the following equation:
Where WT is the total weight of the phycobiliprotein used in the nanoliposome formulation and WF is the amount of free phycobiliprotein in the filtered phase.
The capacity of the PBPs and encapsulated PBPs in nanoliposome to scavenge the free radical 1,1-diphenyl-2-picrylhydrazyl (DPPH) was determined using the research by Ojeda-Sana et al. (2013) with some modifications. The 2,2-azino-bis-3-ethylbenzothiazoline-6-sulfonic acid (ABTS) radical scavenging activity was determined as described by Leite et al. (2011) to assay the antioxidant capacity of free and nano encapsulated samples. First, the nanoliposomes were stirred with magnetic stirrer (model MS-20D, Wertheim, Germany) for 4 h at 200 rpm to release bioactive compounds from the nanocapsules. Butylated hydroxytoluene (BHT) and ascorbic acid were applied as controls and the results were presented as EC50 values in all tests.
Common carps (Cyprinus carpio) were purchased from a culture pond in Iran. The fresh fish was transported to the lab in ice boxes. Then carps were gutted, beheaded and washed in chilled water. They were filleted and deboned manually. The fillets were minced later by a mincer (Moulinex, Darmstadt, Germany). Preparation of fish burger was performed according to the method of Hasani et al. (2020) (Table 1). Produced fish burgers were divided into 5 experimental groups: sample A, control (0% PBPs); sample B, incorporated with 2.5% PBPs; sample C, incorporated with 5% PBPs; sample D, incorporated with 2.5% PBPs nanoliposomes; and sample E, incorporated with 5% PBPs nanoliposomes. The fish burgers were fried at 180°C for 30 seconds in sunflower oil until the color turned slightly brown. The weight of each fish burger was less than 100 g, and burgers were shaped by the shaper. Then burgers were placed in the refrigerator for 18 days of analysis during storage time.
Ingredient | Value (%) |
---|---|
Fish mince | 80 |
Corn flour | 3 |
Sugar | 1 |
Onion powder | 5 |
Garlic powder | 2 |
Bread crumb | 5.2 |
Pepper powder | 0.2 |
Green chili | 1 |
Salt | 1.6 |
Egg white | 1 |
The proximate composition of fish mince was determined as crude protein by the Kjeldahl method. Total lipids, moisture, and ash contents were determined according to AOAC (2005). The pH value of fish burgers was measured by a pH meter (model Wagtech-cyber scan 510, Eutech, England). The peroxide value (PV) of the samples was determined according to the method described by Pearson (Egan et al., 1981). Thiobarbituric acid value (TBA, mg malonaldehyde/kg) was determined colorimetrically according to the method explained by Pearson (1981). Total volatile base nitrogen (TVB-N, mg N/100 g) content of burgers was determined according to the method of Safari & Yosefian (2007). TVB-N was calculated in 100 g of the fish burger according to the following equation.
The total viable counts (TVC) and psychrophilic total counts (PTC) of fish burgers were determined. Burgers (ten grams) of each treatment was aseptically weighed and homogenized with 90 mL sterile 0.1% peptone water for 1 min at room temperature. Furthermore, the decimal dilutions were prepared and then 0.1 mL of each dilution was pipetted onto the surface of tryptic soy agar. They were incubated for 48 hrs at 37°C for TVC and 10 days at 4°C for PTC according to Egan et al. (1981). Microbial loads were expressed as Log10 CFU/g.
Sensory quality of fried fish burger samples was evaluated by 25 trained panelists. The panelists have scored for overall acceptability using a hedonic scale using scores 10, 9, 8, 7, 6, 5, 4, 3, 2, 1 for excellent, like extremely, like very much, like moderately, like slightly, neither like nor dislike, dislike slightly, dislike moderately, dislike very much, and dislike extremely respectively for each of the sensory properties (Watts et al., 1989).
Results and Discussion
The mean diameter and polydispersity index (PDI) of the chitosan-coated nanoliposomes after the sonication process were 515.5 nm and 0.26, respectively (Fig. 2), which is in agreement with previous studies that found the size range of nanocapsules containing plant extracts (Savaghebi et al., 2019). The PDI of nanoliposomes was determined in the range from 0 to 1 so that values greater than 0.5 exhibits a broad dispersion of particle size (Hasani et al., 2018). The PDI of phycobiliprotein nanoliposomes was 0.26 which displayed the nanoliposomes had a uniform size distribution which is the result of a successful production of nanoliposome coated with chitosan. Zeta potential is one of the important factors in determining the physical stability of nanoparticles (Ojagh & Hasani, 2018). The obtained results showed that the surface charge of liposomes was 21.23 mV. Chitosan has a positive charge in acidic conditions due to the free amine groups. After the coating process, the ionic bonds between these groups and the surface of the liposome change the zeta potential from negative to positive. Liu & Park (2009) observed that coating the surface of liposomes with chitosan leads to surface hydrophilicity properties. The most important property of these lipid nanocarriers is the specific effect on target cells by coating with chitosan.
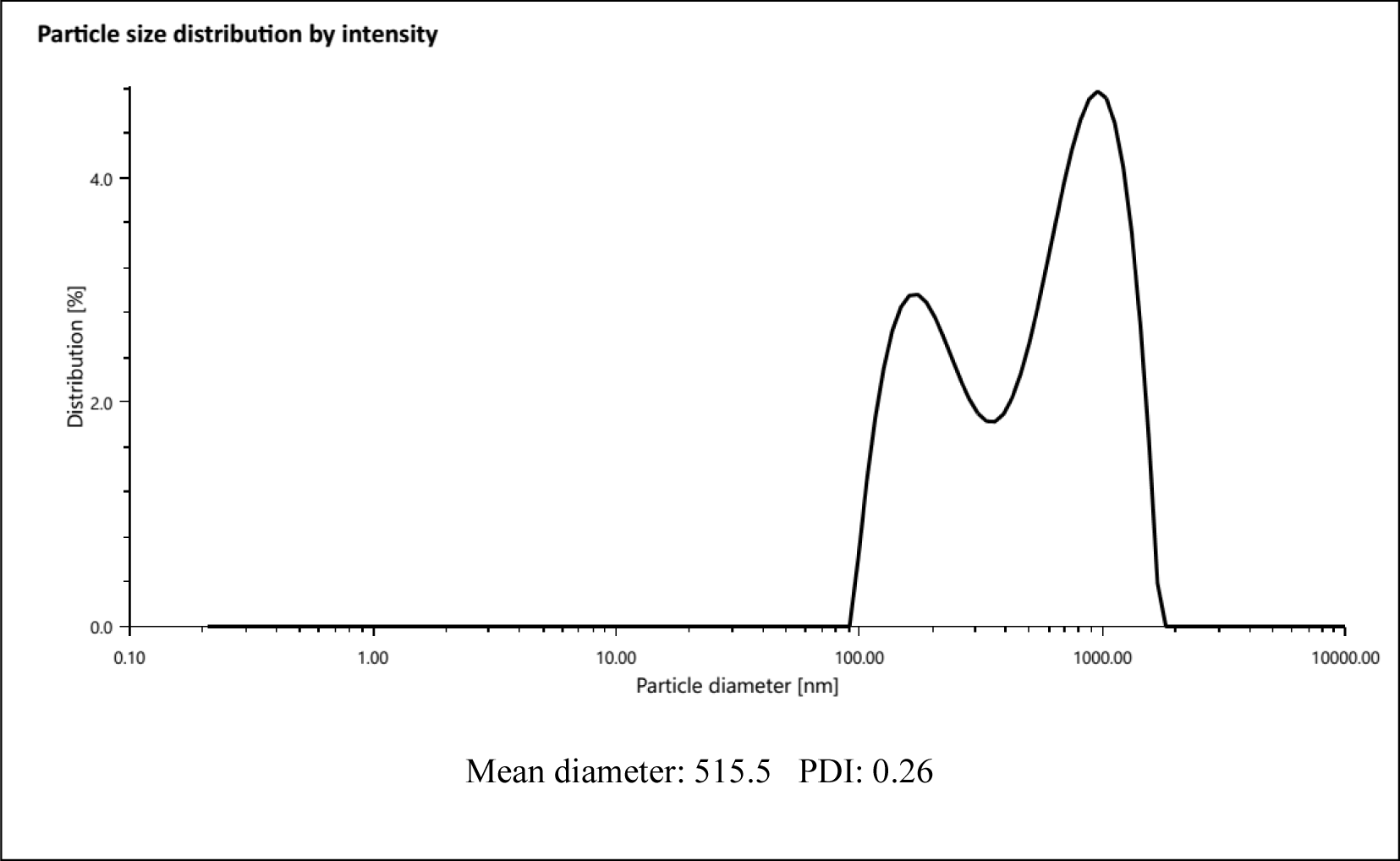
The EE% of the nanoliposomes presented the values 83.98 ± 0.72 which clear that chitosan has a great ability to encapsulate bioactive compounds are found in the phenolic compounds. There are two parts, hydrophilic and hydrophobic in the structure of liposome, so that hydrophilic compounds in the aqueous environment inside the liposome and hydrophobic compounds are enclosed between two phospholipid layers. Therefore, two phospholipid layers act as a reservoir for bioactive compounds. Safari et al. (2020) used the maltodextrin and sodium caseinate as wall materials to nanoencapsulation of phycocyanin with the ratio of 4:1 (coating:core). Based on the results of this study, the encapsulation efficiency of phycocyanin nanocapsules was 48.70, which indicates the high efficiency of this process. The efficiency of the encapsulation process in the study was lower than the present study. The efficiency of the encapsulation technique is affected by the type of coating used, hydrophobic and hydrophilic properties and physicochemical parameters.
The encapsulation efficiency of lipid nanocarriers in the study of Seyed Yagoubi et al. (2017) was reported to be different from 37% to 69% with changes in the amount of fat and surfactant. They found that phycocyanin pigment due to its protein nature has a good emulsifying property that are effective in stabilizing this pigment in the lipid network and increasing the efficiency of the coating.
In this research, the antioxidant properties of PBPs and encapsulated PBPs in nanoliposomes were also investigated. Table 2 shows the values of EC50 in encapsulated and nonencapsulated for DPPH•, ABTS•+ assessment. According to results, the free PBPs showed the lower EC50 (234.5 ± 6.95 ppm) in the DPPH and ABTS test (317.13 ± 7.46 ppm) compared with liposomes without chitosan. Our findings showed that the antioxidant activity of PBPs increased by using chitosan in the liposomal system. Lower antioxidant activity of uncoated-nanoliposomes in comparison with free PBPs demonstrated that bioactive compounds PBPs are located within phospholipid bilayers. Also, Liu et al. (2018) findings presented a similar trend of antioxidant activity in liposomes. The encapsulated PBPs in coated liposomes with 1.5% chitosan had higher antioxidant activity than the other treatment. It can be related to the presence of chitosan in nanoliposomes with hydroxyl groups (OH) in their chemical structures which improve the antioxidant activity by donation of a free electron (hydrogen). It was reported that the chitosan acted as antioxidants by scavenging oxygen radicals such as hydroxyl, superoxide, alkyl as well as extremely stable DPPH radicals tested in vitro (Younes & Rinaudo, 2015).
The yield of mince was 60.30 ± 1.3%, from fish and the initial moisture, protein, fat, and ash content of mince were reported 73.29 ± 0.67%, 15.96 ± 0.23%, 3.13 ± 0.02%, and 2.05 ± 0.05%, respectively. Similar results were reported from common carp (Abdulrahman et al, 2019) and freshwater fish species from the Serrasalmidae family (Rodrigues et al., 2020).
Algae polyphenol or pigments have been shown to successfully prevent lipid oxidation in marine products. Taghavi Takyar et al. (2019) evaluated the effects of ethanolic extracts of Spirulina platensis and Chlorella vulgaris on the decrease of lipid oxidation and increasing the shelf life of rainbow trout fillets at refrigerated storage. Differences in PV of common carp burgers during refrigeration storage are shown in Fig. 3. In this study, the peroxide contents in the treated samples with PBPs and encapsulated PBPs was significantly (p < 0.05) lower than the control during the storage. Biomass or powder of micro and macroalgae have been applied in seafood to improve the quality and extend the shelf-life by decreasing lipid oxidation and microbial growth (Ben Atitallah et al., 2019).
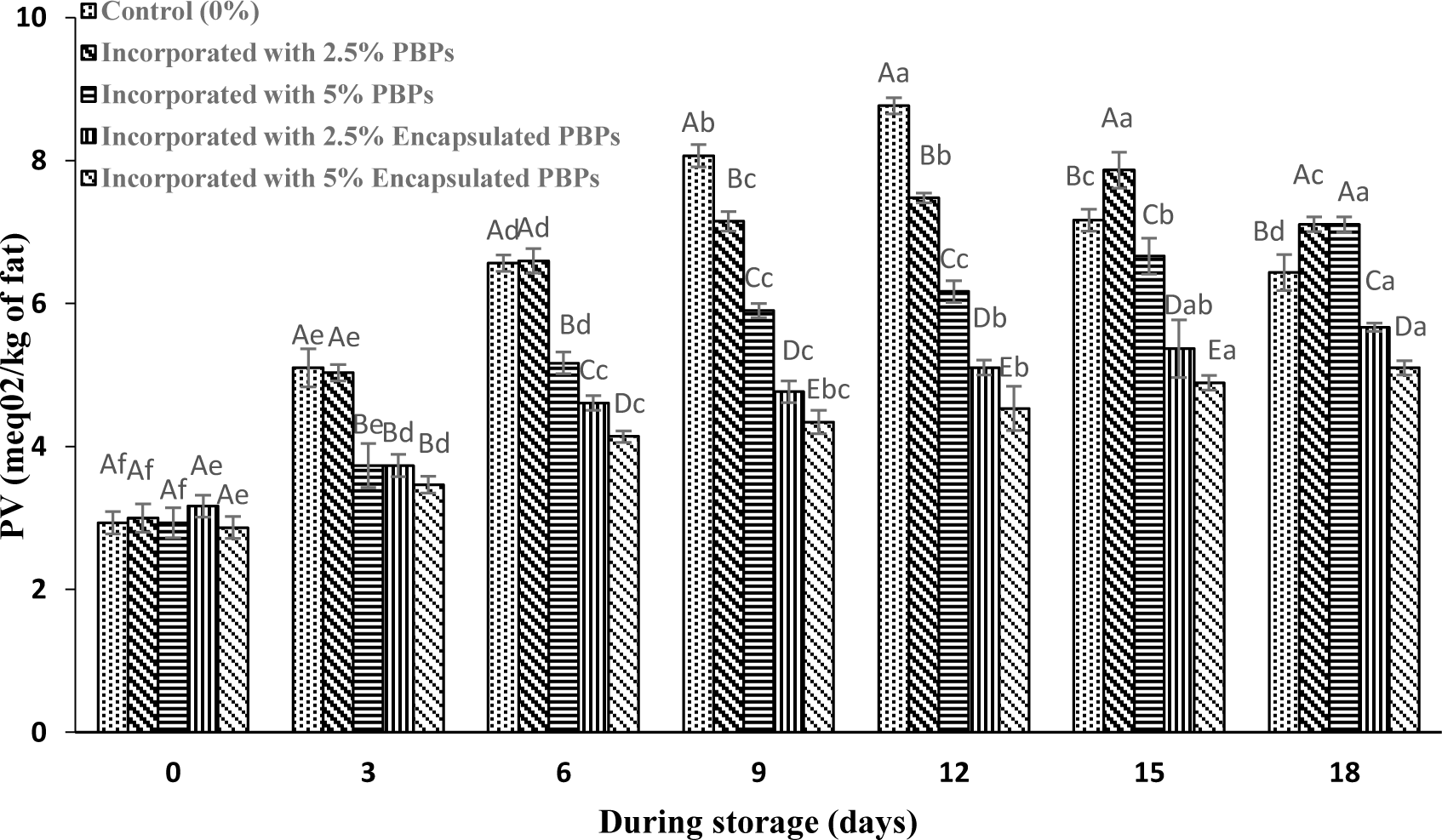
The results have shown that the incorporation of encapsulated PBPs in nanoliposomes had significantly improved the antioxidant potential of samples over 18 days. Chentir et al. (2019) found that utilization of phycocyanin extracted from Arthrospira sp. significantly enhanced the DPPH radical scavenging capacity of gelatin films and restricted the growth of Gram-positive and Gram-negative bacteria.
Variation in TBA values of different treatments during storage is presented in Fig. 4. As can be shown, the initial value of TBA was 0.2 mg MDA/kg, similar to the value reported for fish burgers by Hasani et al. (2020). The TBA values of the common carp burgers increased through the 18 days of storage, especially in the control samples which shows secondary lipid oxidation in the samples. Furthermore, the TBA value of the samples treated with PBPs was significantly lower than the control during the storage, indicating that the PBPs extracted from Gracilaria gracilis could be effective in preventing lipid oxidation. Also, samples incorporated with liposomal encapsulated PBPs showed significantly lower TBA content compared to the control and burgers treated with pure PBPs during the storage period (p ≤ 0.05). This work found that burgers incorporated with 5% PBPs nanocapsules had the lowest PV and TBA (5.10 meqO2/kg fat and 0.53 mg/kg of sample) after 18 days of storage, respectively. This result may be related to the potential of encapsulation in the liposomal system to increase the antioxidant activity of PBPs during utilization on the fish burger by prolonging its availability and presence of chitosan as coating materials due to the hydroxyl groups (OH) in their chemical structures that contribute strongly to the antioxidant activity by donation of a free electron (hydrogen) (Younes & Rinaudo, 2015).
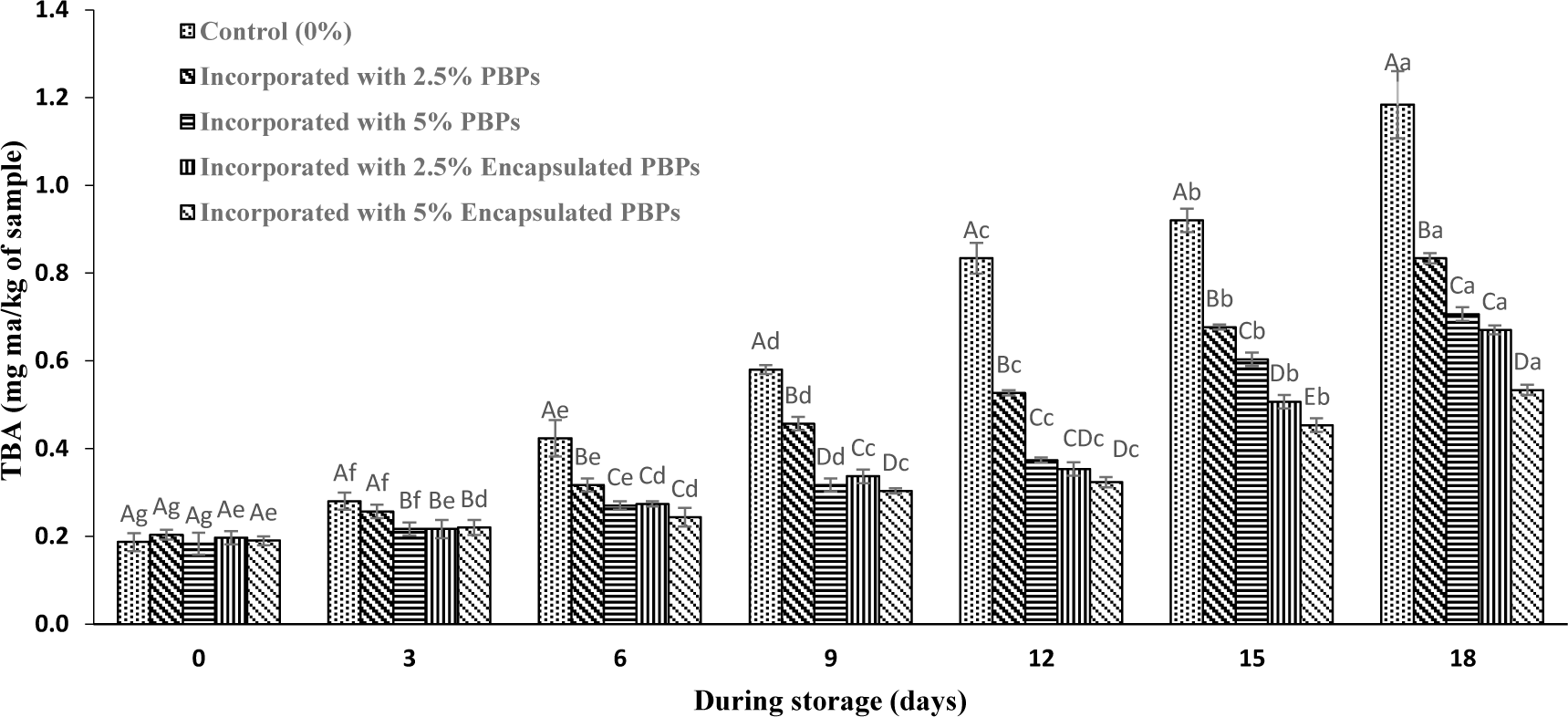
Table 3 shows the effect of PBPs and encapsulated PBPs on the pH value of burgers. The pH of common carp burger increased from 6.77 to 7.97 in control, whereas in samples enriched with 2.5% PBPs, incorporated with 5% PBPs, incorporated with 2.5% and 5%. Encapsulated PBPs in nanoliposome the pH increased from 6.74 to 7.64, 6.67 to 7.62, 6.79 to 7.22, and 6.80 to 7.18, respectively. According to the results, samples incorporated with encapsulated PBPs significantly displayed a limited rate of increase in values than others over storage time. Our results explained that the use of PBPs and PBPs nanoliposome to burger formulation had a significant effect on inhibition of pH changes (p ≤ 0.05) according to the antibacterial and antioxidant effect of PBPs or synergistic effect of chitosan coating in nanoliposomal system on bacteria.
TVB-N is widely studied as an indicator of deterioration of fish products and determined the compounds containing ammonia and primary, secondary, and tertiary amines (Abdollahzadeh et al., 2014). As can be seen in Table 3, the initial TVB-N value of the common carp burgers was 2.92–3.05 mg/100 g in treatments which appeared the good quality of the fresh samples. The TVB-N content increased with prolonging the time of storage for all samples. It was caused by the action of bacteria in the fish muscular tissue that produces ammonia, trimethylamine, and dimethylamine (Raeisi et al., 2019). However, TVB-N values of the samples treated with PBPs were significantly lower than the control during the refrigerated storage (p ≤ 0.05). Our data are in agreement with Hasani et al. (2020) who observed an increase in TVB-N contents from 8.23 to 13.63 mg/100 g in fish burgers stored at 4°C. The lowest TVB-N values in burgers incorporated with 5% PBPs nanoliposome may be related to the antioxidant and antimicrobial activity of algae bioactive compounds and chitosan used as a coating in encapsulation. Gortzi et al. (2006) also displayed that the antimicrobial properties of Origanumdic tamnus extracts after encapsulation in liposome were higher than those of the pure extracts form.
The differences in TVC with the storage time for the control and treated common carp burgers are presented in Table 3. The initial TVC of the produced burgers was low (3.07 Log10 CFU/g), determining the high quality of fish mince used in this experiment and control of hygienic principles of fish processing (ICMSF, 1986). Bacterial load depends on several factors such as manipulation during fillet preparation and processing, contamination of used equipment, and the health of people involved in the work.
Total viable count of the control and burgers incorporated with pure PBPs (2.5%, 5%) and PBPs nanoliposome (2.5%, 5%) increased slowly and reached 8.37, 7.73, 6.80, 5.97, and 5.42 Log10 CFU/g for, respectively, at the end of storage time. According to the results, all burgers treated with different percent of pure PBPs and PBPs nanoliposome significantly inhibited the growth of mesophilic bacteria in burgers in comparison with the control samples during the refrigerated storage. The lower TVC and TPC observed in samples treated with PBPs derived from Gracilaria gracilis may be related to the antibacterial activity of the pure PBPs and liposomal system coated by chitosan as an antimicrobial bioactive compound toward the growth of bacteria in treated burgers. Our results demonstrated that burgers incorporated with 5% nanoliposome had the lowest PTC and TVC values at the end of storage. The results showed that the nano-encapsulated PBPs using chitosan as coating had significant differences with the free PBPs in terms of the bacterial values to the presence of chitosan in the formulation. Many studies have found that chitosan has an important antimicrobial activity. The improvement of the antimicrobial properties of bioactive natural extract, when encapsulated into liposomal delivery systems, was also reported by previous studies (Donsì et al., 2011; Gortzi et al., 2006).
The results of the overall acceptability scores are given in Table 4. The sensory evaluation demonstrated that scores had a significant decrease in all samples during the storage at refrigerated temperature, and the results also prove that the sensory scores decreased significantly with increasing chemical spoilage (p ≤ 0.05). The acceptability scores of burgers incorporated with 5% encapsulated PBPs were higher than other samples. The sensory scores of burgers containing pure PBPs and nanoliposome compared to the control sample showed that the addition of encapsulated and non-encapsulated PBPs could maintain quality of treated burgers without negative effects on odor, color and texture of samples. The results of the sensory evaluation have also shown that samples treated with nanoliposomes were moderately higher in sensory properties than samples treated with non-encapsulated PBPs which matched with chemical properties related to antioxidant and antibacterial properties of the algae phycobiliproteins.
Conclusion
The effect of pure and encapsulated PBPs in nanoliposome in the quality of common carp burgers and the improvement of its antioxidant and antimicrobial properties during refrigerated storage of the burgers were studied. According to chemical, microbial and sensory evaluation burgers treated with 5% nanoliposome containing PBPs with chitosan coating showed lower oxidative spoilage and microbial deterioration during 18 days storage without any significant loss of overall acceptability in comparison with the control and treatment with pure PBPs. In general, the results of the present study proved that the use of PBPs nanoliposome due to the antioxidant, antimicrobial, structural and chemical properties can be a suitable strategy to produce a functional food.