Introduction
Paralytic shellfish toxins (PSTs) are the principal cause of only one type of shellfish poisoning. There are several additional shellfish related marine toxin syndromes such as amnesic shellfish poisoning, neurotoxin shellfish poisoning, diarrhetic shellfish poisoning, etc.
Paralytic shellfish poisoning is only one type of shellfish poisoning caused by the accumulation of toxins from dinoflagellates.
In humans, poisoning through the consumption of toxin-bearing shellfish induces paralysis (Bricelj & Shumway, 1998; Mortensen, 1985). It is true that certain species of cyanobacteria produce toxins, even saxitoxin, but these typically occur in freshwater and are more of a concern for drinking water. The majority of toxins that accumulate in shellfish are from dinoflagellates of marine origin.
In addition to bivalves, PSTs have also been detected in non-filter-feeding gastropods (Itoi et al., 2012), crustaceans (Negri & Llewellyn, 1998), and zooplanktivorous fish (Sephton et al., 2007; White, 1981). Typically, these species accumulate toxins through the food web (White, 1981). Several studies have shown that puffer fish also accumulate PSTs (Abbott et al., 2009; Kodama et al., 1983; Nakashima et al., 2004; Sato et al., 2000).
TTX is structurally different from PSTs, although both are low molecular weight neurotoxins that block sodium channels. The stimulated nerves are therefore unable to generate action potentials, which then leads to paralysis (Kao, 1986). Because this mechanism of action is common to both PSTs and TTX, it is necessary to determine whether puffer fish poisoning is caused by PSTs or by TTX.
In both Korea and the rest of the world, PST poisoning is considered a major public health problem. In addition to PSTs, TTX has a very high fatal dose and is considered to be critical in food hygiene. Food poisoning from puffer fish has also become a social problem in Korea, resulting in fatalities every year (Kim et al., 2003).
In the current study, we focused on the puffer fish, which is a popular fishery product in Korea. Specifically, we studied Takifugu pardalis and Takifugu niphobles, which are known to be highly toxic. Mouse bioassay (MBA) and liquid chromatography-tandem mass spectrometry (LC-MS/MS) were employed to analyze TTX and PSTs in puffer fish, and the effects of these toxins were examined.
Materials and Methods
For the analysis of PSTs and TTX were used to prepare the standard solutions of PSTs, i.e., C1, C2, dcGTX2, dcGTX3, dcSTX, GTX1, GTX2, GTX3, GTX4, GTX5, NEO, STX (Marine Biosciences of the National Research Council, Halifax, NS, Canada) and tetrodotoxin (Alomone Labs, Jerusalem, Israel). High performance liquid chromatography (HPLC) grade solvents acetonitrile, methanol (Merck, Darmstadt, Germany) and an analytical grade reagent hydrochloric acid (Merck) were used for preparation of the analysis sample solution and mobile phase. In addition, mass spectrometry grade reagents formic acid, ammonium formate (Fluka, Buchs, Germany) were used for the preparation of HPLC mobile phases. Analytical grade reagents trichloroacetic acid (TCA), sodium hydroxide, and acetic acid were purchased from Sigma (St. Louis, MO, USA) and deionized water was passed through a Milli-Q water purification system (Millipore, Bedford, MA, USA).
Takifugu pardalis and Takifugu niphobles samples were collected once per month using a fixed set net along Mireuk-do, Tong-Yeong of Korea from January through March, 2014. Harvested fish were stored chilled, typically for less than 24 h prior to testing and dissection of the muscle, intestines, and liver of individuals. These individual samples were kept frozen at –20°C prior to toxin analysis.
PSTs were extracted from 5-g samples of homogenized tissue following the Association of Official Analytical Chemists (AOAC) method (AOAC, 2005) using 5 mL of 0.1 N HCl. These sample extracts were deproteinated for LC-MS/MS analysis, and 25 µL of 30% (w/v) TCA was added to 500 μL of sample extract in a microcentrifuge tube, then mixed in a vortex mixer and centrifuged at 7,000×g for 5 min. Next, 20 μL of 1.0 M NaOH was added to neutralize the solution, which was mixed and centrifuged again. Finally, the solution was filtered through a 0.2 μm syringe filter (Adventec, Tokyo, Japan) into an autosampler vial in preparation for LC-MS/MS analysis by boiling for 10 min and centrifuging at 7,000×g for 10 min at 4°C. The supernatant was filled up to 10 mL with 0.1% acetic acid, and an aliquot of 2 mL was then passed through a 0.2 μm membrane filter (Adventec) for analysis by LC-MS/MS. For TTX analysis, the sample solutions were prepared by the Korean Food Standard (MFDS, 2021). Frozen samples were thawed, and each organ (2 g) from an individual specimen was homogenized and extracted with 8 mL of 0.1% acetic acid by boiling for 10 min and centrifuging at 7,000×g for 10 min at 4°C. The supernatant was filled up to 10 mL with 0.1% acetic acid, then an aliquot of 2 mL was passed through a 0.2 μm membrane filter for analysis by LC-MS/MS. Another 8 mL sample was used in the MBA.
Following the AOAC Official Method 959.08 (2005), 1 mL of HCl extract was injected intraperitoneally into 17–20 g mice, and the times of death were recorded. The concentrations of PSTs were then calculated and presented as μg/100 g STX eq sample using Sommer’s Table. For TTX analysis, 1 mL of 0.1% acetic acid extract was injected into the abdominal cavity, and the times of death were recorded. The mouse unit (MU) was calculated according to the time of death and the body weight of each mouse.
Analysis of PSTs by LC-MS/MS was performed using selective reaction monitoring (SRM). The HPLC unit consisted of a Surveyor MS Pump Plus and Surveyor AS Plus (Thermo Electron, San Jose, CA, USA). A triple-quadrupole mass spectrometer (TSQ Quantum Discovery Max; Thermo Electron, San Jose, CA, USA) was used for mass detection. For toxin determination, SRM-chromatograms were recorded in positive ionization mode for the transitions listed in Table 1. In autosampler, tray temperature was 7°C. These separations were performed on a TSK Gel-amide-80 column (250 × 0.2 mm, 5 μm; Tosoh Bioscience, Tokyo, Japan) preceded by a guard column cartridge (4.0 × 0.2 mm; Tosoh Bioscience) and oven temperature was 35°C. Eluents A (water) and B (acetonitrile-water, 95:5) each contained 2 mM ammonium formate and 3.6 mM formic acid. The analytic conditions were as described previously (Song et al., 2013). TTX analysis was performed using the same LC-MS/MS system and column as PST analysis, with positive ionization mode and eluents A (0.1% formic acid) and B (methanol) as the linear gradient elution. With the aim of validation of the analysis conditions, the mixed standard solution for PSTs were prepared so that it would become 0.001, 0.01, 0.05, 0.1, 0.5, and 1.0 μg/g. TTX standard also prepared 0.01, 0.05, 0.1, 0.5, and 1.0 μg/g. PSTs and TTX standard solution were diluted with 0.003 N hydrochloric acid and injection volume was 10 μL.
PSTs | TTX | ||||||
---|---|---|---|---|---|---|---|
Spray voltage | 5.0 kV | 3.5 kV | |||||
Capillary temp. | 350°C | 320°C | |||||
Sheath gas | 17 (Arb) | 50 (Arb) | |||||
Aux gas | 19 (Arb) | 10 (Arb) | |||||
Polarity | Positive | Positive | |||||
Detection | Selected reaction monitoring (SRM) | SRM |
The limit of detection (LOD) was set to be > 3 as signal to noise ratio (S/N) in the Xcalibur software. The limit of quantitation (LOQ) was determined at a concentration of LOD × 3. The recovery (%) of the test method was analyzed by treating the standard solution, which included base concentration in the samples of pufferfish muscle, through the same pre-treatment method (n = 5). The final concentration of 1.0 μg/g of the standard solutions. Matrix effect (%) was calculated by repeated measurements by adding the sample extracts so that the each toxin standard became 1.0 μg/g.
Results and Discussions
Takifugu pardalis and Takifugu niphobles samples collected between January and March 2014 from the coastal regions of Tongyeong were analyzed for TTX and PST levels using MBA and LC-MS/MS. The samples consisted of seven T. pardalis and seven T. niphobles, each of which was divided into three parts for the experiment: muscles, intestines, and liver. Gender differentiation was difficult because the gonads were not fully developed, and no related tests could be conducted.
We hypothesized that since TTX-bearing marine organisms possess PSTs and that PST-bearing organisms also possess TTX, both types of toxins were analyzed.
The toxicity of the 0.1 N hydrochloric acid extracts and 0.1% acetic acid extracts prepared from three organs of each specimen were analyzed by MBA. Most of the extracts killed mice with typical signs of TTX and PSTs. However, Ahmed (1991) reported that TTX and PSTs are both neurotoxins and produce similar symptoms in mammals, yet the traditional MBA monitoring method for toxins does not distinguish between TTX and PSTs. Therefore, we detected each toxin by LC-MS/MS for superior accuracy.
For TTX analysis, a TTX single standard solution was used in the SRM mode with the parent ion set as m/z 320 and the fragment ions as m/z 302, 256, and 162. As a result, the two types of puffer fish samples yielded a chromatogram identical to the standard solution at a retention time of 3.94 min (Fig. 1). Additionally, a simultaneous analysis of the standard solution mixtures of 12 PST components (C1, C2, dcGTX2, dcGTX3, dcSTX, GTX1, GTX2, GTX3, GTX4, GTX5, NEO, and STX) showed that all toxins were separated within 8 min (Fig. 2); all these toxins, except dcSTX and STX, were detected in the puffer fish samples.
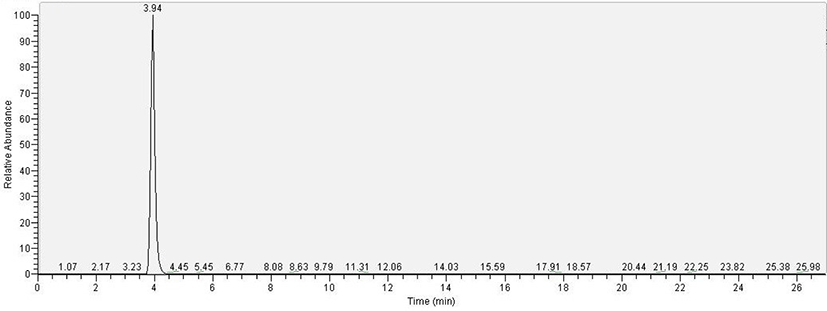
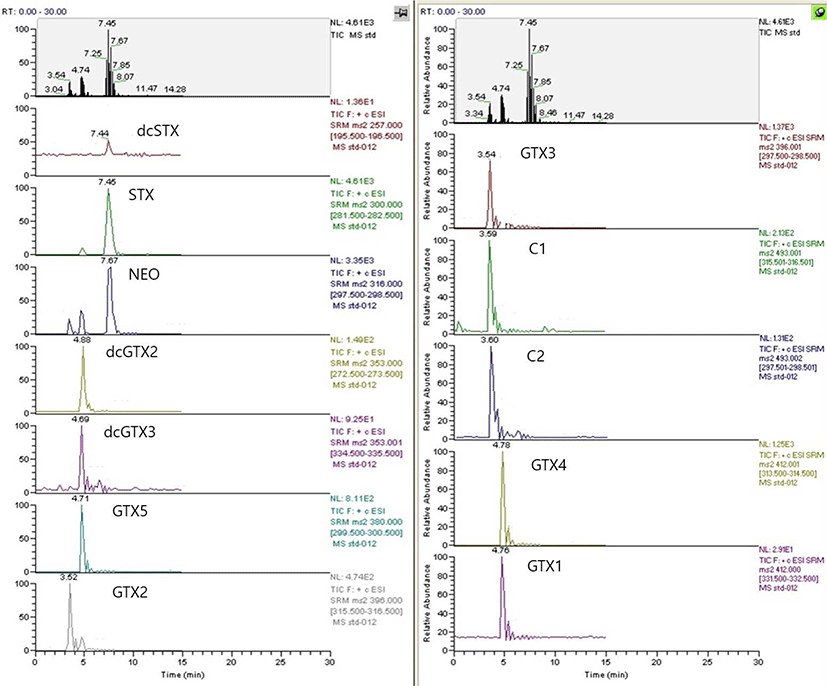
This study used a combination of MS/MS and hydrophilic interaction liquid chromatography (HILIC) in the analysis. HILIC is a very useful method for the separation of polar compounds (Alpert, 1990). This method is advantageous because substances are separated by the interaction of the hydrophilic column and the mobile phase; ionization efficacy is not diminished because the mobile phase does not contain ion pair reagents, and PSTs can be quickly and selectively analyzed simultaneously (Dell’Aversano et al., 2005).
The validation of the analysis conditions, the mixed standard solution for 12 PSTs were prepared so that it would become range of 0.001–1.0 μg/g. The correlation coefficient (r2) of 12 PSTs standard was above 0.99, which showed a good linearity in each concentration range.
LOD for STX, GTX1, GTX3, GTX4, GTX5, dcSTX, and C1 was 0.001 μg/g, besides, NEO, GTX2, dcGTX2, dcGTX3, and C2 was 0.01 μg/g, when S/N was assumed as > 3, and LOQ was 0.003 μg/g and 0.03 μg/g, respectively. And to evaluated recovery (%), the standard solution of 12 PSTs mix were added to pufferfish muscle such that the final concentration of 1 μg/g. Then the spiked samples were treated in the same method of sample pre treatment and analyzed. Determined recovery (%) of samples was in the range of 65.2%–93.7% for 12 PSTs standard. The results showed that C toxins was lower than other toxins. This is thought to be due to interference by salt that was not eliminated by the pre treatment process. Dell’ Aversano et al. (2005) and Song et al. (2013) were also showed that salt contained in crude extracts can partially inhibit C toxins ionization. In addition, the established methods of analysis was almost free from the effect of pufferfish matrixes.
Meanwhile, the correlation coefficient (r2) of TTX is 0.99. LOD and LOQ were 0.01 μg/g and 0.03 μg/g, respectively. It was determined that recovery (%) of tested samples was more than 90%, and there was not matrix effect. Determined TTX pre treatment method was satisfactory for pufferfish.
Table 2 lists the results of the analysis of seven specimens of T. pardalis and T. niphobles, each divided into muscles, intestines, and liver and examined for TTX. In T. pardalis, the TTX levels were within the range of 1.3–1.6 μg/g in the muscles, 18.8–49.8 μg/g in the intestines, and 23.3–96.8 μg/g in the liver. In the T. niphobles, the TTX levels were within the range of 2.0–4.5 μg/g in the muscles, 23.9–71.5 μg/g in the intestines, and 28.1–114.8 μg/g in the liver. Both species showed the lowest detected levels of TTX in the muscles, which is the most commonly consumed portion; the concentrations were the highest in the liver, which is non-edible. This is consistent with the results of previous studies by Jang & Yotsu-Yamashita (2006), who reported a high degree of toxicity in the T. pardalis liver, and Itoi et al. (2012), who showed a high degree of toxicity in the T. niphobles liver. However, previous studies of various types of puffer fish reported differences in toxicity among the same species, depending on the area in which they were caught (Kim et al., 2006; Kim et al., 2007; Kim et al., 2008).
In biological experiments involving mice and conducted from the perspective of food hygiene, concentrations of TTX less than 10 MU/g are not considered harmful; this is in contrast to the TTX detection limits (Kawabata, 1978); 1 MU of TTX is 0.178 μg (Jen et al., 2008). In the current study, when converted to MU, all levels in the muscles were less than 10 MU/g; however, as the TTX levels detected in the intestines and liver were more than 10 times that value, it is thought that these parts are not suitable for consumption.
Table 3 shows the results of the PST detection from the three sample parts using LC-MS/MS. The value of each toxin detected was quantified and converted into saxitoxin equivalents (Table 4; Luckas et al., 2003) and the highest value was noted. In Korea, the maximum permitted PST limit in foods is 80 μg/100 g or 0.8 μg/g (KFDA, 2021).
LC-MS/MS, liquid chromatograph-tandem mass spectrometry; dcSTX, decarbamoylsaxitoxin; ND, not detected; STX, saxitoxin; NEO, neosaxitoxin; dcGTX2, decarbamoylgonyautoxin-2; dcGTX3, decarbamoylgonyautoxin-3; GTX1, gonyautoxin-1; GTX2, gonyautoxin-2; GTX3, gonyautoxin-3; GTX4, gonyautoxin-4; GTX5, gonyautoxin-5; C1, N-sulfocarbamoylgonyautoxin-C1; C2, N-sulfocarbamoylgonyautoxin-C2.
Toxin | ReTx | Toxin | ReTx |
---|---|---|---|
STX | 1.0000 | GTX5 | 0.0644 |
NEO | 0.9243 | dcSTX | 0.5131 |
GTX1 | 0.9940 | dcGTX2 | 0.1538 |
GTX2 | 0.3592 | dcGTX3 | 0.3766 |
GTX3 | 0.6379 | C1 | 0.0060 |
GTX4 | 0.7261 | C2 | 0.0963 |
STX, saxitoxin; GTX5, gonyautoxin-5; NEO, neosaxitoxin; dcSTX, decarbamoylsaxitoxin; GTX1, gonyautoxin-1; dcGTX2, decarbamoylgonyautoxin-2; GTX2, gonyautoxin-2; dcGTX3, decarbamoylgonyautoxin-3; GTX3, gonyautoxin-3; C1, N-sulfocarbamoylgonyautoxin-C1; GTX4, gonyautoxin-4; C2, N-sulfocarbamoylgonyautoxin-C2.
When the toxin levels in each part were compared, both types of puffer fish showed low levels of toxin in the muscles. PSTs were detected mainly in the intestines, and dcGTX3 levels were the most prevalent component for both species examined. However, the relative toxicity of dcGTX3 was 0.3766, which was lower than that of STX (relative toxicity 1). Low levels of toxins were also detected in the liver, with dcGTX3 being the most prevalent component in T. niphobles.
In the TTX analysis, higher concentrations were detected in the liver compared with in the intestines, whereas PSTs were higher in the intestines. Because neither TTX nor PSTs were detected at high levels in the muscles, consumption of this specific part of the puffer fish is considered safe.
The toxicity profile of the detected PSTs is illustrated in Fig. 3. When the PST composition was compared between species, dcGTX3 was the most prevalent component in the panther puffer, and dcSTX, STX, GTX1, GTX4, and C2 were not detected. In T. niphobles, the most prevalent component was dcGTX3, and dcSTX, STX, and NEO were not detected. GTX1, GTX2, and GTX4 levels were higher in T. niphobles than in T. pardalis, and small amounts of C1 and C2 were also detected in T. niphobles. In a previous study of two species of fresh water puffer fish from Bangladesh (Tetrodon cutcutia and Chelonodon patoca), STX, dcSTX, GTX2, GTX3, dcGTX2, and dcGTX3 were detected (Zaman et al., 1997). In another study, dcSTX, neoSTX, and GTX5 were detected in seven species of marine puffers from the Philippines (Sato et al., 2000). Taken together, these results indicate that there is no characteristic PST profile for puffer fish. As mentioned, the composition and content of toxins vary according to habitat, environmental conditions (e.g., freshwater or marine water), and puffer species.
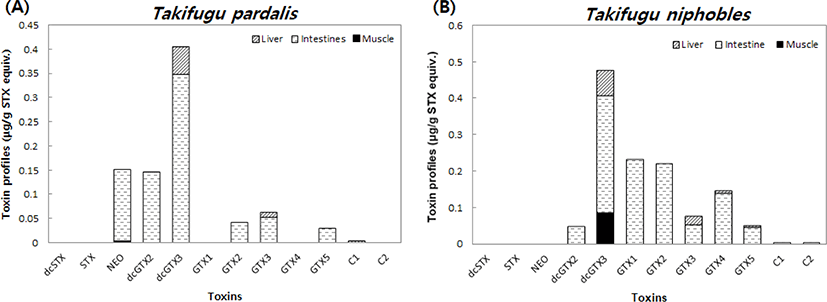
Additionally, when PSTs were calculated as saxitoxin equivalents, the levels were all lower than 0.5 μg/g, which is below the permitted maximum standard of 0.8 μg/g. This suggests that the toxicity of T. pardalis and T. niphobles from the southern coast of Korea is due mainly to TTX and that PSTs do not exert an effect. However, it is low enough in muscle not to be a hazard but the other parts could be hazardous to health and have to be removed from contact with the muscle first.
In summary, the current findings demonstrate that TTX was the major toxin in T. pardalis and T. niphobles inhabiting Korean coastal areas and that PSTs were minor toxins. It indicates that the causes of TTX-induced toxicity in aquatic animals, such as puffer fish, are identical to those of PST-induced toxicity. PSTs are widely known as toxins produced by dinoflagellates, which are a type of phytoplankton. TTX is produced by marine bacteria (Lago et al., 2015). Also, the amount of TTX accumulate in puffer fish through a food chain including bacteria, plankton, invertebrates and fish (Lago et al., 2015). However, TTX has been isolated in significant amounts from the representative PST-producer, Alexandrium tamarense (Kodama et al., 1996; Schantz, 1986). In addition to, Lago et al. (2015) and Sato et al. (2000, 1998) stated that PSTs are also produced by bacteria. In the marine ecosystem, phytoplankton and bacteria produce both PSTs and TTX, which is consistent with the results of the current study.
In future studies, we plan to investigate PST accumulation in other TTX-bearing puffer fish species inhabiting Korean coastal areas. Additionally, we will examine the mechanisms of TTX and PST accumulation by identifying the organisms responsible for puffer toxicity in Korean coastal areas.
Conclusion
This study was hypothesized that since TTX-bearing marine organisms possess PSTs and that PST-bearing organisms also possess TTX, both types of toxins were analyzed. Takifugu pardalis and Takifugu niphobles samples were analyzed for TTX and PST levels using MBA and LC-MS/MS. As a result of the validation of the established method in this study, it was an effective method for the quantification of TTX and PSTs. The results of the present study showed that the lowest detected levels of TTX in the muscles, which is the most commonly consumed portion; the concentrations were the highest in the liver, which in non-edible. The PST levels in each part were compared, that was detected mainly in the intestines, and dcGTX3 levels were the most prevalent component for both species examined. This result signified in the marine ecosystem, phytoplankton and bacteria produce both PSTs and TTX. Additionally, it is low enough in muscle not to be a hazard but the other parts could be hazardous to health and have to be removed from contact with the muscle.