Introduction
Body growth is controlled by environmental factors and genetic condition, which interact to form a complex process across all organs (Argente, 2016). A growth delay occurs when a child is growing at the poor or abnormal rate for their age, and can have a wide variety of causes, including a family history of short stature, constitutional growth delay, growth hormone deficiency, hypothyroidism and poor nutrition (Argente, 2016; Rosenfeld, 2003). Up to now, several therapies are used to improve height growth, such as human growth hormone (hGH) replacement, insulin like growth factor-1 (IGF-1) treatment, and bone lengthening surgery (Argente, 2016; Lee et al., 2020a). Among them, hGH replacement therapy has been prescribed to primate for height growth for more than fifty years (Souza & Collett-Solberg, 2011). Recently, the growing interesting in the predicted final height and socio-economic development often have hGH replacement was carried out children in a normal range who does not correspond to short stature (Argente, 2016; Jeong et al., 2021). The effect of hGH therapy is dose dependent, with lower dosages being less efficacious, whereas too high doses may accelerate growth velocity and stimulate a rapid progression through puberty, possibly limiting final height gain (Kamp et al., 2002; Wit et al., 2002, 2005). In addition, there have been reported that rhGH replacement therapy in children and adolescents caused several side effects including pain at injection site, fever, arthralgia, edema, insulin resistance, and progression of scoliosis (Souza & Collett-Solberg, 2011). Furthermore, since hGH stimulates cell multiplication, development of neoplasms is a concern (Souza & Collett-Solberg, 2011). Noteworthy, there are concerns that hGH-treatment may cause precocious puberty, such as prepubertal gynecomastia (Huh et al., 2021). In this regard, there is a growing need to develop new and effective alternative replacements that have low side effects and are safe for the treatment of growth failure.
Oyster (Crassostrea gigas) is a marine bivalve mollusk widely distributed in coastal areas, and have been long widely used in industrial resources in many countries, including Korea, Japan, and China (Xiang et al., 2021; Zhang et al., 2021). Oyster possesses a high nutrient value, because of its abundant microelements, polypeptide, amino acids and other nutrient factors, which can promote immune modulation and prevent inflammatory disease (Xiang et al., 2021). Moreover, several studies demonstrated that oyster components enhanced bone tissue regeneration and prevent osteoclast differentiation due to their high calcium content (Coringa et al., 2018; Lee et al., 2017). In addition, accumulated evidence has demonstrated that fermented oyster (FO) produced by Lactobacillus brevis BJ20 is contributed to bone health (Ihn et al., 2019; Jeong et al., 2019; Lee et al., 2020a, 2020b; Molagoda et al., 2019). There have been reported that FO promotes osteoblast differentiation, leading to bone formation, and prevents ovariectomy-induced osteoclastogenesis (Ihn et al., 2019; Molagoda et al., 2019). Furthermore, Jeong et al. (2019) suggested that FO protect against receptor activator of nuclear factor-κB ligand-induced osteoclast differentiation. Actually, recent studies established that FO increases body length and enhanced the expression of bone morphogenic proteins in the proximal tibial growth plate (Lee et al., 2020a, 2020b). More recently, there have been demonstrated that supplementation of FO in children with short stature for 24 weeks increase the height, and the effect might be mediated via effects on the bone morphogenic proteins levels (Jeong et al., 2021). However, to date, although there have been established that FO attribute to bone health including promoting osteoblast differentiation, suppression osteoclast differentiation, and increasing heigh, whether administration of FO play as an endocrine disruptor has not been studies. Therefore, the aim of the present study was to investigate the effect of FO on the endocrine system in vitro and in vivo, and to establish the safety for development of dietary supplement for bone health.
Materials and Methods
FO extract was obtained from Marine Bioprocess (Busan, Korea). In brief, oyster was fermented with L. brevis BJ20, and used FO in the study was the same batch as used that in a previous report (Lee et al., 2020b). 17α-ethynyl estradiol, 17β-estradiol, testosterone and testosterone propionate (TP) were obtained from Sigma-Aldrich Chemical (St. Louis, MO, USA). Normal saline was obtained from JW Pharmaceutical, Seoul, Korea). FO and chemicals were dissolved in normal saline.
To evaluate the binding affinity of ligands for estrogen receptor (ER)-α and ER-β of FO (50 μg/mL to 600 μg/mL) were measure using PolarScreenTM ER-α Competitor Assay kit (catalog No. A15882; Thermo Fisher Scientific, Waltham, MA, USA) and PolarScreenTM ER-β Competitor Assay kit (catalog No. A15890; Thermo Fisher Scientific), in accordance with the manufacturer’s instructions. The binding affinity of ligands for androgen receptor (AR) was measured using PolarScreenTM AR Competitor Assay kit (catalog No. A15880; Thermo Fisher Scientific). 17β-estradiol (10−1 to 104 nM) and testosterone were used as positive control for the competitive ER binding assay and the comparative AR binding assay, respectively. Fluorescence polarization was detected using fluorescence microplate reader (Beckman Coulter, Brea, CA, USA) at the Core Facility Center for Tissue Regeneration (Dong-eui University, Busan, Korea). Approximate IC50 values were determined by GraphPad Prism Software (GraphPad Software, La Jolla, CA, USA) from competitive binding curves.
All animal studies were performed in accordance with Institutional Animal Care and Use Committee of Gachon University. The protocol used this study was reviewed and approved by the Center of Animal Care and Use ethical board of Gachon University (CACU; Approval No.: LCDI-2021-0076). Immature male Sprague-Dawley (SD) rats (18-days old, n = 24), immature female SD rats (18-days old, n = 24), mature male SD rats (9-weeks old, n = 6) and mature female SD rats (9-weeks old, n = 6) were obtained from Orient Bio (Sungnam, Korea) and housed in a semi-pathogen-free facility with a temperature of temperature, 23 ± 1°C, relative humidity of 50 ± 5% with 12 h light/12 h dark cycles and ad libitum access to food and water. All animals acclimatized for 1 week.
Hershberger bioassay in immature rats was performed in accordance with the Organisation for Economic Co-operation and Development (OECD) guidelines with modifications (OECD, 2009a; US Environmental Protection Agency, 2021). In brief, immature male SD rats (30 ± 5 g) were randomly divided into four groups based on body weight (BW). The rats were orally administered with i) Saline (5 mL/kg; vehicle control, n = 6), ii) FO100 (100 mg of extract FO/5 mL/kg, n = 6), iii) FO200 (200 mg of extract FO/5 mL/kg, n = 6), and subcutaneously injected with iv) TP (4.0 mg of TP/5 mL/kg; positive control, n = 6) daily for 10 days. All sample were obtained 24 hr after the last day of test substance administration, and the blood was collected into serum separating tube (Becton Dickinson, Franklin Lakes, NJ, USA), centrifuged at 800×g for 20 min and then transferred the supernatants. The organs were dissected free of fat under anatomy microscope (Olympus Optical, Tokyo, Japan), and weighed.
Uterotrophic bioassay in immature rats was performed in accordance with the OECD guidelines (OECD, 2009b). Immature female SD rats (32 ± 5 g) were divided into four groups based on BW. The rats were orally administered daily for 3days with i) Saline (5 mL/kg; vehicle control, n = 6), ii) FO100 (100 mg of extract FO/5 mL/kg, n = 6), iii) FO200 (200 mg of extract FO/5 mL/kg, n = 6), and iv) ethynyl estradiol (EE; 1.0 μg of 17α-EE/5 mL/kg; positive control, n = 6). All animals were necropsied 24 hr after the final administration of test substance, and the serum was separated using serum separating tube at 800×g for 20 min. The organs were dissected free of fat under anatomy microscope, and weighed.
The serum concentrations of luteinizing hormone (LH), follicle stimulating hormone (FSH), testosterone, and 17β- estradiol were measured using LH enzyme-linked immunosorbent assay (ELISA) kit (catalog No. ER1123; FineTest, Hubei, China), FSH ELISA kit (catalog No. ER0960; FineTest), testosterone ELISA kit (catalog No. RTC001R; BioVendor Laboratory Medicine, Brno, Czech), and 17β- Estradiol ELISA kit (catalog No. ADI-900-174; Enzo Life Sciences, Farmingdale, NY, USA). All procedures were performed the manufacturer’s instructions. In immature SD rats, the hormone levels following by administration of FO or positive controls were compared with that from mature SD rats.
Nonparametric tests were used in this study. The Kruskal-Wallis test was used to determine the significance of differences between the four groups. If a significant difference was confirmed by Kruskal-Wallis, multiple comparisons were performed using Mann-Whitney-U test as a post-hoc test. All experiments were performed in triplicate for each animal, and the results are presented as the mean ± standard deviation. Statistical analysis was conducted using SPSS version 22 (IBM, Armonk, NY, USA).
Results
In vitro fluorescence polarization assays were used to determine the relative ER and AR binding affinities of FO. Fig. 1A showed that 17β-estradiol, a positive control, was competitive bind to ER-α and ER-β. The IC50 competitive values for 17β-estradiol on ER-α and ER-β binding are approximately 4.61 nM (1.253 mg/mL) and 5.22 nM (1.421 ng/mL), respectively. However, FO in the concentration range from 50 μg/mL to 600 μg/mL was not combined with ER-α and ER-β (Fig. 1B). Meanwhile, testosterone was also competitive bind to AR, and the IC50 value was approximately 22.14 nM (6.385 ng/mL), while FO had no effect on competitive AR assay (Figs. 1C and D). These results indicated that FO does not interact with ER and AR, suggesting consequentially FO does not play as ligand for ER and AR.
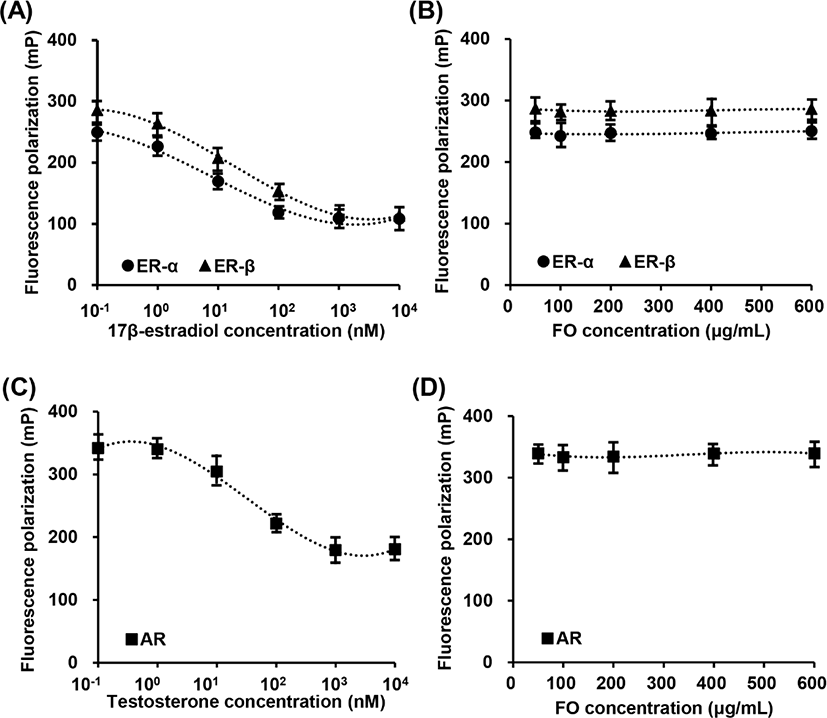
Fig. 2A showed that no significant differences were observed in BW among the groups in immature male SD rats. Furthermore, the relative weights of liver, kidney, adrenal glands and levator ani muscle were no statistical significance between the groups (Table 1 and Fig. 2H). As shown in Figs. 2B–G, the relative weight of androgen-dependent organs, including seimal vesicle, prostate, Cowper’s glands, cavernous body of penis, glans penis and adrenal glands, was significantly increased following by of 4 mg/kg of TP injection for 10 days. However, 100 mg/kg and 200 mg/kg of FO-treated groups, the weights of main androgen-dependent organs were not significantly difference compared with saline group (Figs. 2B–G).
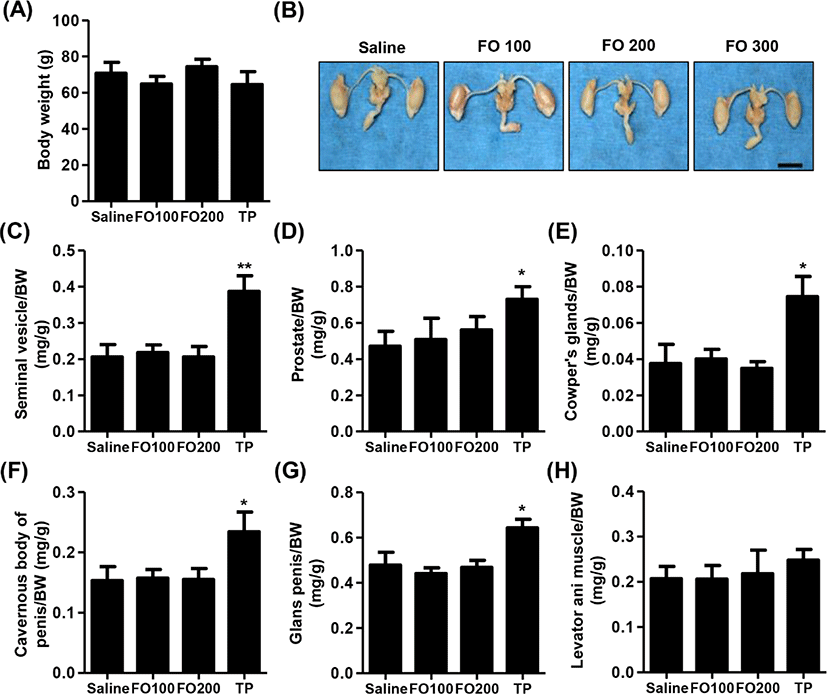
The change of BW after oral administration of saline, 100 mg/kg and 200 mg/kg of FO, and 1 μg/kg of EE for 3 days in immature female SD rats were observed no statistical significance among the groups, although that was slightly increased in EE group (Fig. 3A). In addition, the relative weights of liver, kidney, and adrenal glands were no statistical significance among the groups (Table 1). Nevertheless, the relative weight of estrogen-dependent organs including ovaries, vagina, uterus and oviduct was markedly enhanced by EE administration (Figs. 3B–E). On the other hand, no significant differences were observed in the relative weights of estrogen-dependent organs between the saline group and FO-administered groups.
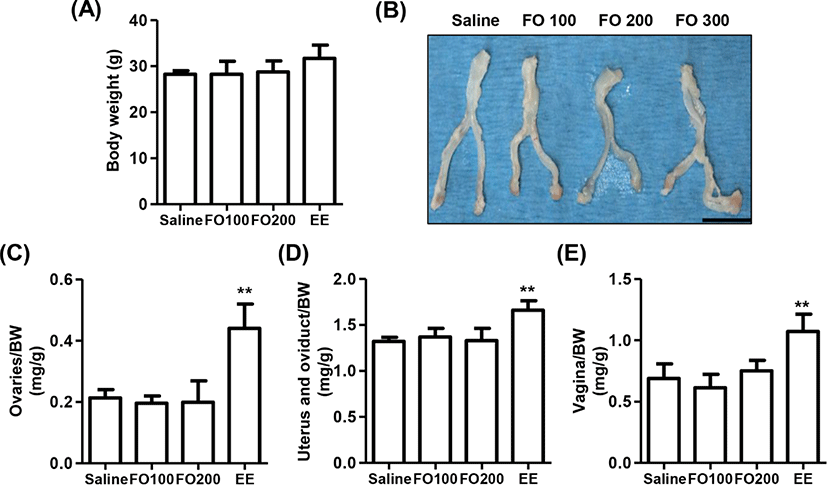
Figs. 4A, B, D and E showed that total serum LH and FSH levels in saline-administered immature rats were significantly higher than those in mature rats. Furthermore, no significant differences were observed in total serum LH and FSH levels between the saline group and FO groups in immature rats. However, the levels of serum LH and FSH were markedly decreased by TP injection in immature male rats, which levels similar to that of saline-treated mature male rats. Meanwhile, the levels of serum testosterone in immature male rats were greatly lower than those in mature male rats (Fig. 4C). The testosterone levels were no significantly difference between saline and FO administration groups, whereas the levels significantly enhanced in TP-treated immature male rats (Fig. 4C). Similar to immature male rats, the serum LH and FSH levels were also significantly reduced by orally administered EE in immature female rats. Additionally, the serum levels of 17β-estradiol in immature female rats were substantially lower than that in mature female rats (Fig. 4F). These levels were significantly increased by oral administration of EE in immature female rats, but not altered by oral administration of FO.
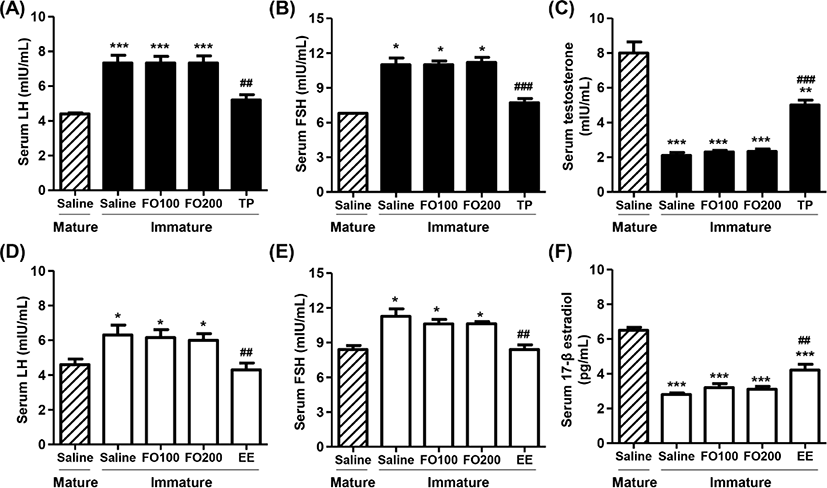
Discussion
In the present study, we investigated the effect of FO on the endocrine system in vitro and in vivo. An endocrine-disrupting compound was defined by the U.S. Environmental Protection Agency (EPA) as “an exogenous agent that interferes with synthesis, secretion, transport, metabolism, binding action, or elimination of natural blood-borne hormones that are present in the body and are responsible for homeostasis, reproduction, and developmental process.” (Diamanti-Kandarakis et al., 2009). Endocrine disruptors act through nuclear hormone receptors, ERs, Ars, progesterone receptors, thyroid receptors, and retinoid receptors, among others (Calafat & Needham, 2007; Diamanti-Kandarakis et al., 2009; Dickerson & Gore, 2007). In this concern, several methods of examination to evaluate the effect of endocrine system disrupt including sexual maturation were established in accordance of OECD guideline (OECD, 2009a, 2009b; Yamasaki et al., 2003).
Hershberger bioassay that established as OECD test guideline (TG) 441 is a short-term in vivo screening assay in male rats for chemicals that interact with the AR (OECD, 2018). It is based on changes in weight of the accessory tissues of the male reproductive tract in response to androgens and anti-androgens in animal models where endogenous androgens are minimal as a result of castration or because the animals are immature (OECD, 2018). According to OECD TG441, the rat model utilizes the weights of five androgen-dependent sex accessory tissues, including ventral prostate, seminal vesicles, levator ani plus bulbocavernosus muscles, Cowper’s glands and glans penis. Furthermore, these include the thyroid hormones on the thyroid effects may also be obtained, and LH, FSH and testosterone (OECD, 2009a, 2018). In the present study, we found that oral administration of 100mg/kg and 200 mg/kg of FO for 10 days doesn’t have effect on the kidney, liver, and androgen-dependent sex accessory organs, including seimal vesicle, prostate, Cowper’s glands, cavernous body of penis, glans penis and adrenal glands in immature male rats. Furthermore, FO has no effect on the serum levels of LH, FSH, and testosterone. Additionally, our result showed that FO does not interact with AR indicating FO does not act as a ligand for AR. To validate the hershberger bioassay and AR competitive binding assay, we used TP and testosterone as a positive control, respectively. Testosterone competitively interacted with AR in vitro assay, and significantly increased the relative weight of androgen-dependent sex accessory tissues and markedly suppressed the serum LH and FSH levels in immature male rats. These results demonstrated that in vitro and in vivo exposure of FO have non-androgenic effects.
The uterotrophic bioassay (TG 440) was validated by the OECD as a short-term screening test to evaluate the ability of a substance to elicit estrogenic activity (Kanno et al., 2003; OECD, 2009b; Owens & Koëter, 2003). This is considered the standard method for identification of potential ER agonists that way interfere with ER signaling (Kleinstreuer et al., 2016). The end point measured is an increase in uterine weight caused by ER-mediated water imbibition and cellular proliferation in the uterine tissue (Kleinstreuer et al., 2016; OECD, 2009b). In this validated method, there has been recommended that immature female rats or ovariectomized (OVX) adult female rats can be used, because immature and OVX animals do not produce endogenous estrogens, the uterus becomes sensitive to external estrogenic substances (Billon-Galés et al., 2011; Kleinstreuer et al., 2016). Estrogen is a steroid hormone, and its synthesis and secretion directly inhibit the secretion of the FSH and LH by the pituitary gland (Zhao et al., 2019). In the present study, we investigated the effect of FO on estrogenic activity in vitro and in vivo. Our results showed that oral administration of FO for 3 days doesn’t have effect on the relative weight of estrogen-dependent sex accessory organs, including ovaries, vagina, uterus and oviduct in immature female rats. In addition, FO has no effect on the serum levels of LH, FSH, and 17β-estradiol, and does not interact with ER-α and ER-β. Furthermore, we have verified the 17α-EE significantly increased the relative weight of estrogen-dependent sex accessory organs in immature female rats, and 17β-estradiol strongly interacted with ER-α and ER-β. Based on these results, we considered that FO have no effect on estrogen activity.
In conclusion, our finding showed that FO does not interact with ER and AR, suggesting consequentially FO does not play as ligand for ER and AR. Furthermore, oral administration of FO did not act as an endocrine disruptor including have androgenic activity, estrogenic activity, and abnormal levels of sex hormone, indicating FO may ensure the safety on endocrine system for development of dietary supplement for bone health.