Background
The annual growth rate of the aquaculture industry has experienced a faster expansion during the last decades compared with other animal protein food-producing industries such as pork, poultry, and beef (Galkanda-Arachchige et al. 2019). But further development of the aquaculture industry highly depends on how seriously sustainable development criteria are being considered. The feed is a key factor for the sustainable development of the aquaculture industry as it can significantly influence the production costs (50–70%) and trophic transfer efficiency (Hishamunda et al. 2009). Among all the different protein sources used in the fish diet, fishmeal is an excellent source of essential amino acids with high palatability and digestibility properties. Fishmeal also contains other nutrients such as essential fatty acids, minerals, vitamins, phospholipids, and nucleotides (Tacon and Metian 2015). But, high demand and low supply of fishmeal due to overexploitation of fisheries resources have resulted in increased fishmeal prices. Therefore, many scientists have been looking for alternative protein sources in order to minimize fish feed costs and promote sustainable aquaculture development.
Several plant and animal protein sources have been tested for total or partial replacement of fishmeal in aquafeed. Soybean meal is one of the most commonly used plant proteins in fish feed due to global availability, low price, good nutritional value, and relatively balanced amino acid profile (Gatlin et al. 2007). Animal rendered by-products such as meat and bone meal, poultry by-product meal, and tankage meal have also been used as the fishmeal alternatives due to good digestibility, nutritional value, and low price (Galkanda-Arachchige et al. 2019). Tankage meal is commonly known as the processed product of dead farm animals and is derived from whole carcasses including hoofs and skin (Hertrampf and Piedad-Pascual 2000). The nutritional composition of tankage meal is not much different from meat and bone meal which is widely used as a fishmeal substitute in aquafeed (Rossi Jr and Davis 2014). Poultry by-product meal is also one of the common ingredients widely used in fish and shrimp feed with high nutritional value, good palatability, and digestibility. Poultry by-product meal is derived from different parts of poultry carcasses excluding feather and intestine and including head, feet, neck, and undeveloped egg (Galkanda-Arachchige et al. 2019). Despite all the practical aspects of these animal by-products and plant protein ingredients, high levels of fishmeal replacement by these ingredients in the carnivorous fish diet has resulted in lower growth, feed efficiency, and immunity (Rossi Jr and Davis 2014).
Olive flounder (Paralichthys olivaceus) is a marine carnivorous fish species with a high market value in East Asia. This species is the most produced fish among other aquaculture finfish species in the Republic of Korea with more than 41 thousand tons in 2017 (FAO 2020). However, limited success has been achieved in the formulation of sustainable feed as olive flounder commercial feed contains more than 60% fishmeal. Also, most of the fishmeal replacement research in olive flounder has been conducted in juvenile and at the laboratory conditions (Hamidoghli et al. 2020a). Therefore, this practical on-farm feeding trial aims to replace 10–20% of fishmeal in a control diet (containing 65% fishmeal) by animal and plant proteins (tankage meal, poultry by-product meal, and soy protein concentrate) in the diet of sub-adult olive flounder.
Materials and methods
The formulation of experimental diets was based on nutritional requirements of olive flounder previously described by Hamidoghli et al. (2020a) and are presented in Table 1. The main protein sources in the basal diet were sardine fishmeal (32.47%), anchovy fishmeal (32.47%), and soybean meal (14.90%). Fish oil was used as the main lipid source in the present experiment. Whereas, all diets were isonitrogenous (62% protein) and isolipdic (9% lipid). The three experimental diets consisted of a basal diet with no fishmeal replacement as the control diet (CONT), 10% replacement of fishmeal by tankage meal as an animal protein source (AP10), and 20% replacement of fishmeal by tankage meal, poultry by-product meal, and soy protein concentrate (SPC) as animal and plant protein sources (APP20). The amino acid compositions of the three experimental diets are shown in Table 2. There were no major differences in the amino acid composition of experimental diets and amino acids were matched to the nutritional requirement of olive flounder. Preparation of diets was done at the National Institute of Fisheries Science (NIFS), Pohang, Republic of Korea. All fine-powdered and dried ingredients were mixed based on the feed formulation using an industrial ribbon mixer (RBM series, Sung Chang Machinery Co., LTD, Seoul, Republic of Korea). Thoroughly mixed ingredients were transferred to an extruder machine (Twin extruder, ATX-II, Fesco, Republic of Korea) to make floating extruded pellets with a size of 11–13 mm. During the extrusion process, barrel three, four, and five had a temperature of 85.8, 83.9, and 79.4, respectively. Cutter volume was set at 20 and cutter RPM was 16%. Then, the extruded pellets were dried using an industrial oven drier at 60 °C for 6 h. Fish oil was added to the extruded pellets at the last stage to act as a coating material and help with the floating aspect.
1SPC soy protein concentrate
2Contains (as mg/kg in diets): Ascorbic acid, 300; dl-Calcium pantothenate, 150; Choline bitate, 3000; Inositol, 150; Menadion, 6; Niacin, 150; Pyridoxine HCl, 15; Rivoflavin, 30; Thiamine mononitrate, 15; dl-a-Tocopherol acetate, 201; Retinyl acetate, 6; Biotin, 1.5; Folic acid, 5.4; Cobalamin, 0.06.
3Contains (as mg/kg in diets): NaCl, 437.4; MgSO4˙7H2O, 1379.8; ZnSO4˙7H2O, 226.4; Fe-Citrate, 299; MnSO4, 0.016; FeSO4, 0.0378; CuSO4, 0.00033; Calciumiodate, 0.0006; MgO, 0.00135; NaSeO3, 0.00025.
4Ectra (% of mixture): Vitamin C (0.3%), Vitamin E (0.3%), Choline (0.8%), antifungal agent (0.06%)
Sub-adult olive flounder was purchased from a private farm (Geoje-si, Gyeongsangnam-do, Republic of Korea). The feeding trial was conducted at the Song-hae aquaculture farm located at Namhae, Republic of Korea. Fish were accumulated to the new environment by feeding the CONT diet for 1 month. After this period, 90 olive flounder (327 ± 9.3 g, mean ± SD) were randomly distributed in circular plastic tanks with 5 m diameter and 0.7 m depth. Three tanks (replicates) were considered for each diet in a flow-through system (pumped seawater). Fish were fed up to satiation level (0.5–1.2% of body weight) two times per day at 8:00 and 16:00 for 16 weeks. Total fish weight in each tank was determined every month and the feeding rate was adjusted accordingly. Fish were fed the 11 mm feed for the first 8 weeks and the 13 mm feed for the following 8 weeks. Water temperature was approximately 21.1 ± 4.4 °C and salinity was 33 ± 1 ppt during the feeding trial with small fluctuations.
At the end of the 16 weeks feeding trial, the total number and weight of fish in each tank were measured for the calculation of growth performance parameters including weight gain (WG), feed efficiency (FE), specific growth rate (SGR), protein efficiency ratio (PER), and survival. Three fish from each tank were randomly selected to measure hepatosomatic index (HSI), viscerosomatic index (VSI), and condition factor (CF).
Three fish per tank were randomly selected and blood samples were collected from the caudal vein of each fish. Serum was separated from blood samples by centrifugation at 5000g for 10 min and stored at −70 °C until analysis. Determination of serum biochemical parameters including aspartate aminotransferase (AST), alanine aminotransferase (ALT), glucose (GLU), total protein (TP), and total cholesterol (TCH) was performed with an automatic chemical analyzer Fuji DRICHEM 3500i (Fuji Photo Film Ltd., Tokyo, Japan). Non-specific immune responses including lysozyme and superoxide dismutase (SOD) activity were determined according to the method described by Hamidoghli et al. (2020b). SOD was determined using an assay kit (Enzo ADI-900-157, Enzo Life Sciences, Inc.) with a water-soluble tetrazolium salt that is reduced by O−2 and produces a dye that can be measured spectrophotometrically at 450 nm. Serum lysozyme was determined by the turbidimetric method using Micrococcus lysodeikticus suspension and the amount of produced enzyme was measured through spectrophotometric absorbance.
In addition, three fish from each tank were removed for the analysis of whole-body proximate composition. The proximate composition of flounder whole-body and the experimental diets were performed by standard methods of Association of Official Analytical Chemists, AOAC (2005). Fish whole-body samples were ground thoroughly, pooled, and then freeze-dried for 48 h (Advantage 2.0, VirTis, New York, USA). Moisture content was determined by drying in an oven at 105 °C (OF-02G-4C, WiseVen®, Germany). Crude ash was determined by combustion at 550 °C using a muffle furnace (DAIHAN, WiseTherm®, Germany). Crude protein was analyzed by the Kjeldahl method (2300 Autoanalyzer, Foss Tecator. AB, Hoganas, Sweden) following the strong acid digestion of samples, distillation, and titration for measuring nitrogen content (N × 6.25). Crude lipid content was analyzed by soxhlet extraction method (ether extract) using the soxhlet system 1046 (Tecator AB, Hoganas, Sweden). The diet and whole-body samples were hydrolyzed with 6 N HCL at 110 °C for 24 h. Quantification of amino acids was performed using high-performance liquid chromatography (HPLC) by the ninhydrin method (Sykam Amino Acid Analyzer S433, Sykam; Eresing, Germany).
The intestinal histology was done as previously described by Bae et al. (2020). Three fish from each tank were dissected, 1 cm sections of anterior intestine were removed and fixed in the 10% neutral buffered formalin. Then the samples were dehydrated in graded levels of ethanol and embedded in paraffin. Paraffin blocks of tissue were made and were sectioned (4 μm thick) using a rotary microtome (CUT 4055, MicroTec, Germany). The sliced sections were stained with hematoxylin and eosin (H&E) and observed in a light microscope. Villi length was observed in each sample and mean values were compared statistically.
All the data were analyzed by one-way ANOVA (SAS Institute, Cary, NC, USA) to determine significant differences at P < 0.05 level for various parameters. When differences were significant, the least significant difference (LSD) test was used to define which groups are significantly different.
Results
During the 16 weeks of the feeding trial, all diets seem to have sufficient palatability and well-accepted by olive flounder. Proximate composition and amino acid analysis of diets did not show significant differences and diets were considered nutritionally balanced (Tables 1 and 2). Results of growth performance did not show significant differences for WG, SGR, and CF for fish fed all the experimental diets (P > 0.05). Moreover, feed utilization performance parameters such as FE and PER were not significantly different among all treatment groups (P > 0.05). Fish fed CONT, AP10, and APP20 diets did not show significant differences in organosomatic indices such as VSI and HSI (Table 3). The survival percentage of fish was not affected by dietary treatments.
The whole-body proximate composition of sub-adult olive flounder fed the experimental diets for 16 weeks is presented in Table 4. These results indicate no significant differences in whole-body proximate composition among fish fed the different experimental diets (P > 0.05). Amino acid composition of sub-adult olive flounder whole-body also shows no significant differences in fish fed the different dietary treatments (Table 5).
Hematological parameters of sub-adult olive flounder fed the three experimental diets is shown in Table 6. Fish fed the AP10 and APP20 diets showed no significant difference in AST, ALT, GLU, TP, and TCH compared with those of fish fed the CONT diet (P > 0.05).
The non-specific immune response of sub-adult olive flounder fed the three experimental diets for 16 weeks is presented in Table 7. SOD and lysozyme activities of fish fed the AP10 and APP20 diets were no significantly different from those of fish fed the CONT diet (P > 0.05).
The anterior intestinal morphology of fish fed the three experimental diets is shown in Fig. 1. According to these figures, there was no damaged intestinal structure or villi observed. A comparison of villi length of the anterior intestine of sub-adult olive flounder fed the experimental diets for 16 weeks is shown in Table 8. There were no significant differences in villus length of fish fed CONT, AP10, and APP20 diets (P > 0.05).
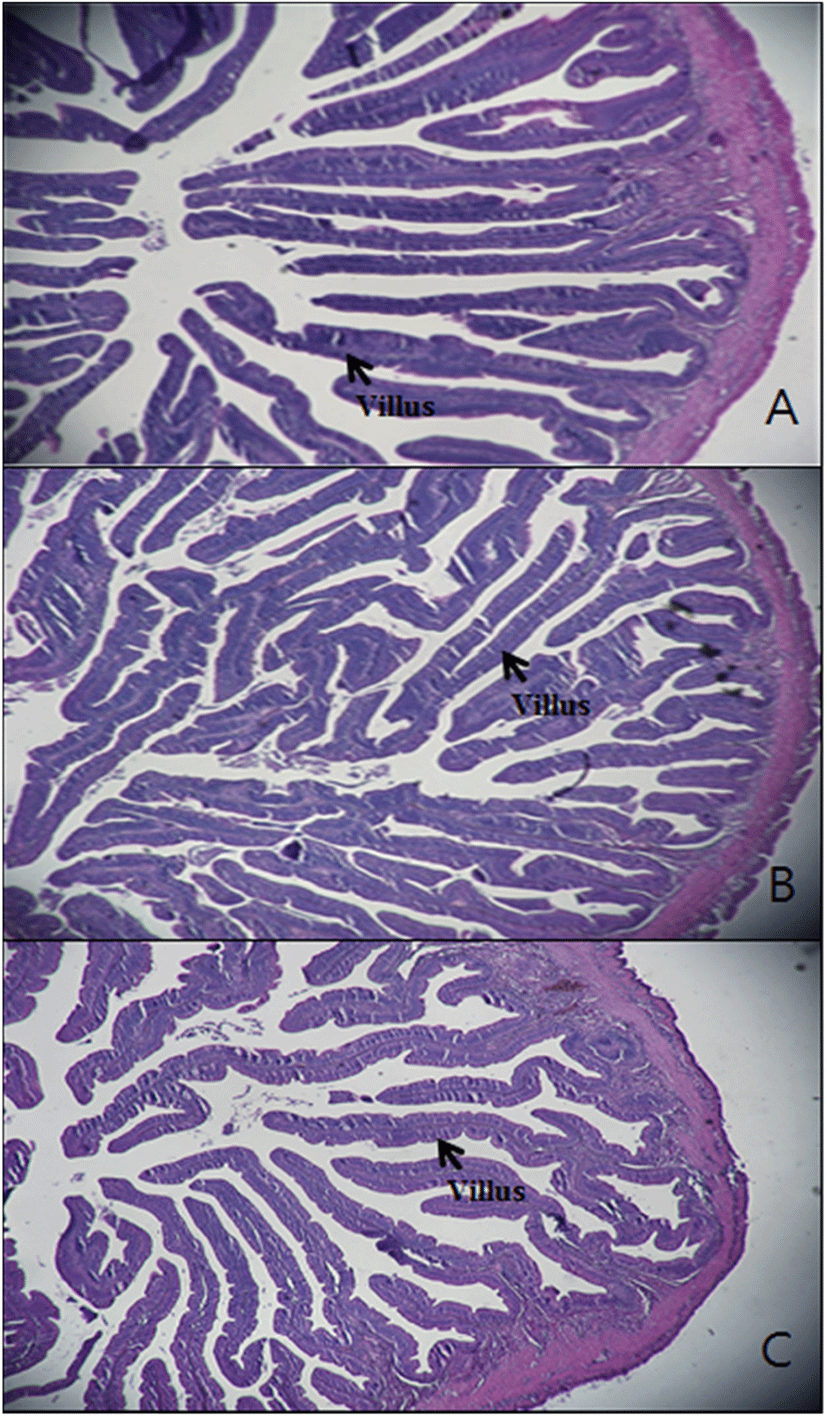
Discussion
The amino acid composition of experimental diets was sufficient to support the requirements of olive flounder. Few studies were found investigating the essential amino acid requirement of olive flounder. Based on these studies, arginine, lysine, and methionine requirements of olive flounder are 2.04–2.10%, 1.5–2.1%, and 1.44–1.46%, respectively (Hamidoghli et al. 2020a). According to Table 2, all these requirements were met by the experimental diets, except for methionine in the control diet (0.1% lower). But no deficiency signs were observed during the experiment and all diets were equally palatable and well-accepted by fish.
1Values are means of triplicate groups of fish
2Weight gain (WG, %) = (final wt. − initial wt.) × 100/initial wt
3Specific growth rate (SGR, %) = (loge final wt. − loge initial wt.) × 100/days
4Condition factor (CF, %) = (wet weight/total length3) × 100
5Feed efficiency (FE, %) = (wet weight gain/dry feed intake) × 100
6Protein efficiency ratio (PER) = (wet weight gain/protein intake)
7Visceralsomatic index (VSI, %) = viscera wt. × 100/body wt.
8Hepatosomatic index (HSI, %) = liver wt. × 100/body wt.
9Survival (SUR, %) = 100 × (final fish number/initial fish number)
Diets | |||
---|---|---|---|
CONT | AP10 | APP20 | |
Moisture | 75.5 ± 1.43ns | 74.8 ± 1.58 | 74.4 ± 1.09 |
Crude protein | 17.8 ± 1.66ns | 18.2 ± 0.50 | 18.9 ± 0.52 |
Crude lipid | 3.51 ± 0.10ns | 3.99 ± 0.19 | 3.66 ± 1.15 |
crude ash | 4.09 ± 0.27ns | 4.06 ± 0.34 | 4.00 ± 0.51 |
1Values are means from triplicate groups of fish
1Values are means from triplicate groups of fish
1Values are means from triplicate groups of fish
2AST (U/L): Aspartate aminotransferase
3ALT (U/L): Alanine aminotransferase
4GLU (mg/dl): Glucose
5TP (g/dl): Total protein
6TCH (mg/dl): Total cholesterol
Diets | |||
---|---|---|---|
CONT | AP10 | APP20 | |
SOD2 | 85.9 ± 9.48ns | 79.5 ± 9.74 | 72.4 ± 6.24 |
Lysozyme3 | 2.63 ± 0.77ns | 2.28 ± 0.33 | 2.92 ± 0.52 |
1Values are means from triplicate groups of fish
2SOD (% inhibition): Superoxide dismutase activity
3Lysozyme (U/ml): Lysozyme activity
Diets | |||
---|---|---|---|
CONT | AP10 | APP20 | |
Villus length (μm) | 895 ± 111ns | 858 ± 98.5 | 758 ± 139 |
1Values are means from triplicate groups of fish
Growth performance parameters such as WG, SGR, CF, FE, PER, VSI, and HSI were not influenced by the dietary treatments in this study. This means that up to 20% of fishmeal can be replaced by the combination of tankage meal, poultry by-product meal, and soy protein concentrate with no deterioration effects on growth performance. Previously, Kikuchi et al. (1997) reported that 20% of fishmeal can be replaced by meat and bone meal in juvenile olive flounder (4 g) diet with no significant effects on FE, PER, and daily feed consumption. These authors also demonstrated that 40% replacement of fishmeal can have negative effects on growth performance parameters. In another study by Lee et al. (2012), 20% of fishmeal was successfully replaced by meat and bone meal in juvenile olive flounder. This is while only 10% replacement was possible with ingredients such as silkworm pupae meal or promate meal without changes in growth parameters. Generally, the most important aspects that need to be considered in fishmeal replacers are nutritional composition (amino acid balance), palatability, and digestibility (Jobling 2016). In the present study, 20% replacement of fishmeal with tankage meal, poultry by-product meal, and soy protein concentrate mixture could have been possible due to proper nutritional composition, palatability, and digestibility of these ingredients. This statement can be supported by diet proximate and amino acid composition along with growth performance (WG and SGR) and feed utilization performance (FE and PER) results.
The whole-body proximate and amino acid composition of olive flounder after 16 weeks of the feeding trial further supported the results obtained for growth performance (Tables 4 and 5). Fish body proximate composition is an important factor used in fish nutrition studies and is highly influenced by the feeding regime, body size, seasonal changes, and habitat (Shearer et al. 1994; Hardy and Barrows, 2002). Lee et al. (2012), demonstrated that 20% replacement of fishmeal with meat and bone meal in olive flounder did not influence whole body and liver moisture, protein, and ash composition but reduced the crude lipid content. The proximate composition of moisture, lipid, and protein in the fish body can directly determine the energy storage capacity, growth, reproduction, and survival (Luo et al. 2013). This is contrary to the results of the present experiment and could be explained by the properties of fishmeal replacers used in both studies.
In the present study, serum biochemical parameters of olive flounder were not significantly affected by the replacement of fishmeal at 10 and 20%. Serum AST, ALT, GLU, TP, and TCH reflect the response of fish species to changes in the environmental and biological conditions (Haider 1973). Similar to our results, Seong et al. (2018) observed no significant differences in serum AST, ALT, GLU, and TP of juvenile olive flounder (8.3 g) when fishmeal was replaced by fermented soybean meal. These parameters are clear indicators of damaged liver and/or stress in fish (Hilmy et al. 1985; Shahsavani et al. 2010; Jiang et al. 2015; Abdelkhalek et al. 2015). Along with these parameters, the nonspecific immune response parameters such as serum lysozyme and super oxidase dismutase activity showed insignificant differences which further supports the results for growth performance, whole-body proximate composition, and hematological indices. Therefore, it could be suggested that alternative protein sources used in the present study did not cause stress or tissue damage in olive flounder.
One of the reasons for growth deterioration in fishmeal replacement studies could be related to intestinal histology changes. Intestinal histology is an important aspect that helps with the understanding of pathological alteration of different nutritional sources (Rašković et al. 2011). In the present study, intestinal histology of olive flounder did not show any signs of physical damage or villi length differences when fishmeal was replaced at 10 or 20%. This is in accordance with the results obtained by Shi et al. (2017), where 100% of fishmeal was replaced by Chlorella meal in the carp diet. In contrast, Kokou et al. (2015) observed significant changes in gastrointestinal tract morphology when 60% of fishmeal was replaced by soybean meal in gilthead sea bream. These authors concluded that antinutritional factors present in some ingredients could have negative effects on gastrointestinal morphology.
Rendered animal by-products such as tankage meal, meat and bone meal, and poultry by-product meal are low-price ingredients with great potential as fishmeal replacers (Rossi Jr and Davis 2014; Galkanda-Arachchige et al. 2019). But it has been shown that a mixture of animal and plant proteins can be more suitable fishmeal replaces in aquaculture (Lee et al. 2002; Ye et al. 2011). There are, of course, other studies reporting higher replacement of fishmeal in olive flounder diet with other animal or plant ingredients. For example, Kim et al. (2014) reported that 30% of fishmeal can be replaced by tuna by-product meal in olive flounder without adverse effects on WG, SGR, feed efficiency ratio, or protein retention. It is worth mentioning that most of the fishmeal replacement studies on olive flounder have been done on juvenile fish at experimental (laboratory) condition, whereas, the present study investigated the replacement of fishmeal in sub-adult olive flounder raised in actual farm condition.
Conclusions
Based on the results of this experiment, 10 and 20 % of dietary fishmeal can be replaced by tankage meal and combination of tankage meal, poultry by-product meal, and soy protein concentrate, respectively, in olive flounder. This conclusion is based on the results obtained from growth performance, whole-body proximate and amino acid composition, serum biochemical parameters, non-specific immune responses, and intestinal histology.