Introduction
Protein assumes greater importance in aquacultural feeds mainly due to the fact that the level and quality of protein greatly influences feed cost. Therefore, protein content should be carefully adjusted in feeds, bearing in mind that the dietary protein in excess to that required for growth is only catabolized (Cowey 1979) and that protein inadequacy leads to poor growth and feed inefficiency. Consequently, to improve the utilization of protein for tissue synthesis rather than for energy purposes, an adequate knowledge of protein requirements and of the effect of environmental factors on protein utilization is necessary.
Dietary protein requirements are dependent on a variety of factors such as stock size, water temperature, feeding frequency, amount of non-protein dietary energy, and dietary protein quality (Shimeno et al. 1980). As fish is an ectotherm, and water temperature is one of the most important abiotic factors affecting growth and survival of the aquatic animals. All fish species are characterized by an ideal range of temperature in which they show their maximum growth (Oyugi et al. 2011). Any alterations in the optimum water temperature have a marked and direct effect on many of the key physiological processes and behavioral activities (Brett 1979; Jonassen et al. 2000; Sarma et al. 2010) which can also be detected in the form of alterations in hematological parameters (Haider 1973; Steinhagen et al. 1990). Temperature beyond optimum limits of a particular species adversely affects the health of aquatic animal due to metabolic stress and increases oxygen demand and susceptibility to diseases (Wedemeyer et al. 1999). It limits the biochemical reactions, affects their metabolism and distribution, and directly influences the survival and growth at the various stages of their life cycle.
Catfishes are the preferred candidate species for aquaculture owing to their consumer preference and commercial and medicinal value. Among those, Heteropneustes fossilis, commonly known as the stinging catfish or singhi, is considered as one of the most demanded freshwater air breathing fish species in the tropical waters of the Indian subcontinent and Southeast Asian region (Christopher et al. 2010). The range encompasses India, Thailand, Bangladesh, Pakistan, Nepal, Sri Lanka, Myanmar, Indonesia, and Cambodia (Burgess 1989). Its primary habitat includes ponds, ditches, swamps, and marshes. It is hardy, amenable to high stocking densities, and adapts well to hypoxic water bodies (Dehadrai et al. 1985). Due to the presence of accessory respiratory organs, it has got the ability to utilize atmospheric oxygen for respiration and, therefore, can survive for quite a few hours outside the water which makes it an ideal species for aquaculture (Vijayakumar et al. 1998; Haniffa and Sridhar 2002). Heteropneustes fossilis is an important tropical freshwater food fish (Mohamed and Ibrahim 2001). It has very high iron content (226 mg/100 g) and fairly high content of calcium compared to many other freshwater fishes (Saha and Guha 1939). Being a lean fish, it is very suitable for people to whom animal fats are undesirable (Rahman et al. 1982). Due to high nutritive value and low fat content, the stinging catfish is recommended in the diets of the sick and the convalescents (Alok et al. 1993). The fish efficiently utilizes prepared feeds and is able to withstand adverse environmental conditions. In addition to this, it has high nutritional and medicinal value (Pillay 1990; Jhingran 1991; Thakur 1991). This fish is popular particularly because it can be cultivated in swampy areas and derelict water bodies without involving costly reclamation. It is easily stored and transported live to consumers. Thus, this species is ideal for wastewater aquaculture as well (Tharakan and Joy 1996) and is abundantly available in open water system of floodplains, canals, and beels.
The effects of water temperature on growth and protein requirements of fish have been well documented for many species (El-Sayed et al. 1996; Van Ham et al. 2003; Anelli et al. 2004; Chatterjee et al. 2004; Larsson and Berglund 2005; Han et al. 2008; Singh et al. 2008; Singh et al. 2009; Huang et al. 2016; Mishra et al. 2019). Some aspects of nutrition of H. fossilis has been worked out in the past mainly on determining its optimum feeding practices and nutritional requirements (Niamat and Jafri 1984; Akand et al. 1991; Jhingran 1991; Anwar and Jafri 1992; Firdaus 1993; Firdaus et al. 1994; Firdaus and Jafri 1996; Mohamed 2001; Mohamed and Ibrahim 2001; Firdaus et al. 2002; Usmani and Jafri 2002; Usmani et al. 2003; Ahmed 2007; Siddiqui and Khan 2009; Ahmed 2010; Khan and Abidi 2010; Khan and Abidi 2011a, 2011b; Ahmed 2012; Farhat 2011; Farhat 2012; Khan and Abidi 2012; Ahmed 2013a, 2013b; Farhat 2013a, 2013b; Ahmed 2014; Farhat 2014a, 2014b, 2014c; Khan and Abidi 2014; Ahmed 2017; Farhat 2017); however, study on effect of water temperature on the nutritional requirements of H. fossilis under culture condition has not been worked out.
Since biochemical parameters such as serum aspartate amino transferase (AST) and alanine amino transferase (ALT) levels and the hematological parameters commonly measured clinically as biomarkers for health and good indicator of various sources of stress, to ascertain the effect of temperature on dietary protein requirement more precisely, these parameters are also considered and analyzed.
The aim of this study was to determine the influence of water temperature on protein requirement and to optimize the rearing temperature so that this fish could maximize its performance in terms of growth and health in an intensive culture system.
Materials and methods
Seven casein-gelatin-based isocaloric diets (14.99 kJ/g digestible energy) with varying levels of dietary protein (28, 32, 36, 40, 44, 48, 52% protein) were prepared (Table 1). Diets were designated as D28, D32, D36, D40, D44, D48, and D52. Two intact protein sources, casein and gelatin, were used at 4:1 ratio. The dietary protein level was increased by adjusting the fractions of casein and gelatin in the diet. Dextrin was served as the carbohydrate source. A combination of cod liver oil and corn oil (2:5) was used as a source of lipid to provide n-3 and n-6 fatty acids. Vitamin and mineral premixes were prepared as per Halver (2002). Digestible energy (DE) was calculated using conversion factors of 33.5, 20.9, and 12.6 kJ/g for fat, protein, and carbohydrate, respectively (Halver and Hardy 2002). The diet was prepared as per Siddiqui and Khan (2009). The final diet in the form of dough was cut into small cubes, sealed in polythene bags, and kept in refrigerator at − 20 °C till further use.
aCrude protein (80 g/100 g)
bCrude protein (95 g/100 g)
cCod liver oil from SevenSeas Ltd., Hull, UK.
dCorn oil was obtained from Fortune, Adani Wilmar Ltd
eHalver (2002)
fVitamin mixture (1 g vitamin mix +2g œ-cellulose) choline chloride 0.50; inositol 0.20; ascorbyl-2-polyphosphate 0.10; nicotinic acid 0.075; calcium pantothenate 0.05; riboflavin 0.02; menadione 0.004; pyridoxine hydrochloride 0.005; thiamin hydrochloride 0.005; folic acid 0.0015; biotin 0.0005; alpha-tocopheryl acetate 0.04; vitamin B12 0.00001; Loba Chemie, India
gMineral mixture (g/100 g) calcium biphosphate 13.57; calcium lactate 32.69; ferric citrate 2.97; magnesium sulphate 13.20; potassium phosphate (dibasic) 23.98; sodium biphosphate 8.72; sodium chloride 4.35; aluminium chloride.6H2O 0.0154; potassium iodide 0.015; cuprous chloride 0.010; magnus sulphate. H2O 0.80; cobalt chloride. 6H2O 0.1; zinc sulphate. 7H2O 0.40; Loba Chemie, India
hCalculated on the basis of fuel values 23, 20.19, 16.0, and 37.6 kJ/g for casein, gelatin, dextrin, and fat, respectively, as estimated on Gallenkamp ballistic bomb calorimeter
iEstimated on Gallenkamp ballistic bomb calorimeter
Induced-bred fry of the stinging catfish, H. fossilis, were obtained from the Ghazipur Fish Market, New Delhi, India and transported to wet laboratory (Fish Rearing Laboratory, Department of Zoology, Central University of Kashmir, J&K), given a prophylactic dip in KMnO4 solution (1:3000) and stocked in indoor circular aqua-blue colored, plastic lined (Plastic Crafts Corp, Mumbai, India) fish tanks (1.22 m × 0.91 m × 0.91 m; water volume 600 L) for about 2 weeks. They were then acclimated to two different constant temperatures (18 and 26 °C). The desired temperatures were adjusted with the help of thermostatic water heaters (Rusun, Fish Aquarium Home, Laxhami Nagar, New Delhi, India). Prior to the commencement of the feeding trial, fish were acclimated to the respective water temperatures for 7 days to stabilize their internal mediums and allow metabolic compensation (Castille Jr and Lawrence 1981) and to ensure full thermal adaptation. During this period, the fish were fed with a casein-gelatin based H-440 diet (Halver 2002) thrice a day (0700, 1200, 1730 h) until apparent satiation, at each temperature. The apparent satiety was ensured simply by visual observation and the fish were carefully observed during feeding to ensure satiety without overfeeding. The diet was fed as long as the fish actively consumed it at each feeding schedule. Since feed allocation was done till the fish desired to feed and no feed was dispensed once the fish stopped feeding actively, there was no unconsumed feed in the culture tank. A photoperiod of 12 h light/12 h dark was maintained throughout the experimental period.
For conducting the present experiment, H. fossilis fry (2.97 ± 0.65 cm; 5.11 ± 0.34 g) were sorted out from the above acclimated lot and stocked in quadruplet groups (n = 4 tanks per treatment) in 70-L circular polyvinyl troughs (water volume 60 L). The experiment was conducted in a thermostatic experimental setup. Throughout the experimental period (84 days), temperature was regularly measured three times daily with a thermometer at each feeding schedule. Fish were fed experimental diets in the form of semi-moist cakes in the form of cube (1 × 1 × 1 cm) as per the above feeding schedule. Initial and weekly individual weights were recorded on a top-loading balance (Sartorius CPA- 224S 0.1 mg sensitivity, Goettingen, Germany) after anaesthetizing with tricane methane sulphonate (MS-222; 20 mg/L; Finquel®). The feeding trial lasted for 84 days. Fish were fasted on the day of weekly measurements. A KMnO4 bath was administered after every weighing session (5 g/L for 30 min) as a prophylactic measure. Fecal matter, if any, was siphoned off before and after every feeding. The culture troughs were siphoned once every day. The experiments were conducted absolutely as per the guidelines for animal ethics.
Water quality parameters of the troughs were maintained at different temperatures (18 and 26 °C). Water was sampled from each trough to determine water temperature, dissolved oxygen, free carbon dioxide, total alkalinity, TAN, nitrite, and pH based on daily measurements following the standard methods (APHA 1992). The pH was determined by using digital pH meter (pH ep-HI 98107, USA).
At the end of experiment, eight fish were randomly sampled to assess environmental stress (high temperature) trial. The fish were exposed to high temperature (33 °C) and the mortality time was recorded in seconds.
Six subsamples of a pooled sample of 20 fishes were analyzed for initial body composition. At the end of the experiment, all 30 fishes from each replicate of dietary treatments were pooled separately and three subsamples of each replicate from the pooled sample (n = 4) were analyzed for final carcass composition. Proximate composition of casein, gelatin, experimental diet, and initial and final body composition was estimated using standard methods (AOAC 1995) for dry matter (oven drying at 105 ± 1 °C for 22 h), crude protein, (nitrogen estimation using Kjeltec 8400, Hoeganas, Sweden), crude fat (solvent extraction with petroleum ether B.P 40–60 °C for 2–4 h by using Soxlet extraction technique, FOSS Avanti automatic 2050 equipment, Sweden), and ash oven incineration at 650 °C for 2–4 h. To confirm the calculated levels of gross energy of the prepared test diets, each dietary sample was ignited in Gallenkamp ballistic bomb calorimeter (Gallenkamp Ballistic Bomb Calorimeter-CBB 330 010L, Gallenkamp, Loughbrough, UK). The analysis revealed a close agreement with the calculated values of the gross energy density (Table 1).
Evaluation of the hematological parameters involved the determination of the red blood cell count (RBCs × 109), hemoglobin content (Hb; g dL−1), and hematocrit value (Hct%). At the end of the experiment, fish were anaesthetized with MS-222 (20 mg/L; Finquel®) before taking the blood samples. The blood samples were then collected from the caudal vein of individual fish (nine fish from each replicate of the treatment) employing heparinized syringes. To avoid blood coagulation, all mixers, pipettes, and test tubes used were rinsed with anticoagulant (3.8% solution of sodium citrate). Erythrocyte count was determined by an improved Neubauer hematocytometer with Yokoyama’s (1974) solution as the diluting medium. Blood hemoglobin was determined spectroscopically (Genesis, UV) following Wong’s (1928) method and was expressed in grams per deciliter (Hb g/dL). Hematocrit value (Hct%) was measured by spinning the micro-wintrobe tube containing well mixed blood for about 5 min at 12,000g and then measuring the packed cell volume which was expressed in percentage.
On the final day of the feeding trial, five fish from each tank (n = 4x5) were anesthetized (MS-222; 20 mg/L) before subjecting to body measurements. The fish, liver, and viscera of each specimen were weighed by blotting dry on a filter paper, and total length of the fish was taken. The values were recorded to calculate the hepatosomatic index (HSI%), viscerosomatic index (VSI%), and condition factor (CF).
Blood serum was collected after centrifugation at 3000 rpm for 10 min and then stored at − 20 °C in order to analyze aspartate aminotransferase (AST) and alanine aminotransferase (ALT) activities. Biochemical analysis of serum AST and ALT activities were done as per Reitman and Frankel (1957).
Growth performance of the fish fed experimental diets at different temperatures was measured as a function of the weight gain by calculating following parameters:
A completely randomized design with four replicates per treatment was used for assessing the optimum protein requirement of the fish at two different temperatures. All growth data were subjected to two-way ANOVA as per Snedecor and Cochran (1982) to test any differences and/or the interaction between dietary protein and temperature. Differences among treatment means were determined by Duncan’s multiple range test at a P < 0.05 level of significance (Duncan 1955). Relationship between dietary protein level and protein deposition (PD) g/fish was modeled using second-degree polynomial regression analysis (Zeitoun et al. 1976). The protein requirement of fry H. fossilis was determined as the point on the graph where the biological response was found to be equal to 95% of the maximum response. All the statistical analyses were done using Origin (version 6.1; Origin Software, San Clemente, CA).
Results
Data on average thermal growth coefficient (TGC), feed conversion ratio (FCR), and protein deposition (PD g/fish) of the fry Heteropneustes fossilis, after 84 days of feeding are summarized in Table 2. Growth performance and feed intake were significantly affected by both dietary protein levels (P < 0.0007) and rearing temperature (P < 0.0001). However, interactive effects of dietary protein and temperature were not found (P > 0.05). H. fossilis fry fed diets containing different levels of protein exhibited superior response in terms of TGC, FCR, and PD g fish−1 with 44% protein at 26 °C temperature. The groups reared at 18 °C showed a consistent improvement in their performance up to 40% protein in the diet. However, the values recorded for TGC, FCR, and PD g fish−1 for the groups held at 18 °C were inferior compared to those held at 26 °C even though fed with the same level of dietary protein. This indicates that fish held at this temperature failed to express their maximum growth potential.
Ave in wt average initial weight, Ave fn wt average final weight, TGC thermal growth coefficient, FCR feed conversion ratio, PD protein deposition, FI feed intake, different upper case letter indicate significant differences (P < 0.05) between temperatures whereas different with superscripts indicate significant differences between dietary protein levels; NS nonsignificant (P > 0.05)
The dietary protein levels and rearing temperatures had significant influence on carcass protein of H. fossilis fry (Table 3); however, interactive effects of both were not recorded (P > 0.05). Moisture content of H. fossilis showed a positive correlation with the increase in dietary protein at both the temperatures (18 and 26 °C), whereas the carcass fat content showed a negative correlation. Carcass protein tended to increase significantly (P < 0.05) in fish fed 40 and 44% protein (diet D40 and D44) at 18 and 26 °C temperatures (P < 0.05). Moreover, temperature had no significant effect on carcass fat and ash contents.
NS non-significant (P > 0.05)
Different upper case superscripts indicate significant differences (P < 0.05) between temperatures, whereas different with superscripts indicate significant differences between dietary protein levels
As per the results, significant differences (P < 0.05) in terms of ALT and AST activities were recorded among the two experimental groups reared at two different temperatures. The values of ALT and AST were significantly (P < 0.05) higher among the groups reared at 18 °C temperature compared to those reared at 26 °C water temperature. Also, serum enzymes of H. fossilis seem not to be much affected by different protein levels in this study but were more affected by water temperatures (Table 4). However, the interactive effects of dietary protein and temperature were not found.
AST aspartate amino transferases, ALT alanine amino transferases, Hct hematocrit, Hb hemoglobin, RBCs red blood cells count
Different upper case superscripts indicate significant differences (P < 0.05) between temperatures, whereas different with superscripts indicate significant differences between dietary protein levels; NS non-significant (P > 0.05)
Dietary protein and temperature had significant impact on hematological parameters (P < 0.05) with no interactive effects of both. Fish fed diet containing 28% protein achieved the lowest hematological values at both the temperatures. RBCs, Hb g/dL, and Hct% increased with increasing levels of dietary protein up to 44% (diet D44), both at 18 and 26 °C rearing temperatures (Table 4). Thereafter, the above hematological parameters remained almost the same in fish fed 48% (diet D48) dietary protein and the exhibited a decline with further increase in the dietary protein intake at 52% (diet D52). However, at 18 °C, the magnitude of response in above values was comparatively lower than that attained in fish raised at 26 °C for the same dietary protein level.
VSI and HSI decreased with increase in dietary protein levels up to 44% and increased in fish fed dietary protein beyond 44% at 48 (D48) and 52% protein (D52). Hepatosomatic index (HSI) was found to be influenced by the levels of dietary protein and temperatures but no interaction occurred, while viscerosomatic index (VSI) was affected by only diets and not by temperatures (Table 5).
HSI hepatosomatic index, VSI viscerosomatic index, CF condition factor
Different upper case superscripts indicate significant differences (P < 0.05) between temperatures, whereas different with superscripts indicate significant differences between dietary protein levels; NS non-significant (P > 0.05)
Resistance rate to thermal stress increased significantly (P < 0.05) among the groups reared at 26 °C water temperature than those reared at 18 °C (Table 5). The stress resistance response remained best for the groups fed with 44% dietary protein. This parameter was affected by both dietary protein levels and rearing temperatures; however, no interactive effects of both were noted (P > 0.23). The normal values of water quality parameters during the entire length of experiment are provided in Table 6.
Mean ± SD (n = 183). Recorded thrice daily at 07:00, 12:00, and 17:30 h
aUndetectable
Based on the above response parameters, second-degree polynomial regression analyses were performed to study the relationships between dietary protein levels and the protein deposition and were expressed in the form of Y = aX2=+ bX + c. The value of X that corresponds to Y95%max was defined as the requirement. PD g/fish data (Y95%max) to dietary protein levels (X) was subjected to a second-degree polynomial regression analysis. The curve attained its 95% maximum response at 40.8 and 41.8% protein of the diet (Fig. 1) at 18 °C and 26 °C water temperature, respectively.
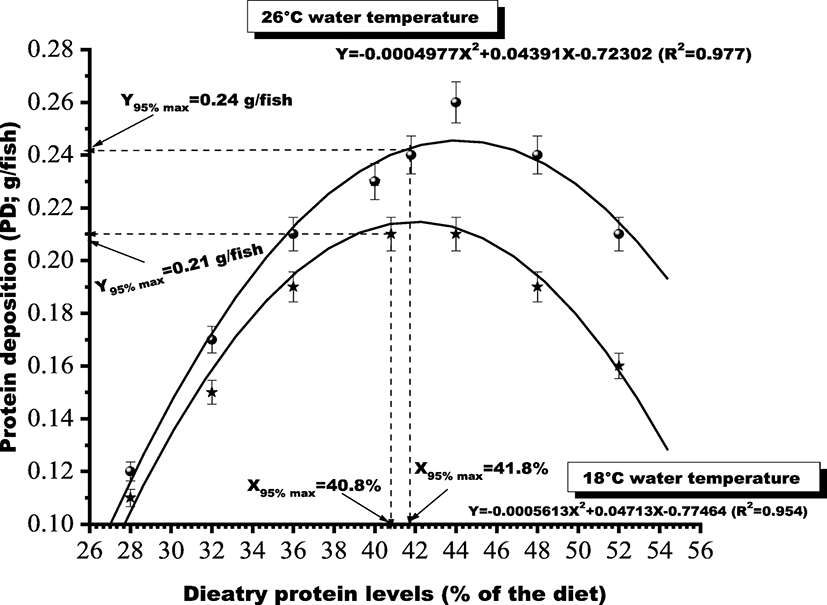
Discussion
The aim of this study is to assess the influence of water temperature on dietary protein requirement, protein deposition, carcass quality, and hematological parameters of fry H. fossilis. Temperature is a pervasive factor affecting food intake, growth, and food conversion of fish (Fry 1947; Brett 1979). Since water temperature has potent influence on metabolic rate and energy expenditure affecting nutrient requirement and growth performance of the poikilothermic vertebrates including fish (Brett 1979; Dutta 1994; Bhikajee and Gobin 1998), its influence on nutrient requirement and growth warrant thorough investigation.
Several studies have reported that the specific water temperature range showed that the faster growth and low temperature causes sluggishness by retarding the digestion speeding of fish (Bailey and Alanara 2006). Some researchers have found that the digestion rate has been increased as the temperature increases (Turker 2009). Environmental temperature is one of the most important ecological factors which also influence the behavior and physiological process of aquatic animals (Xia and Li 2010).
The results showed that growth in terms of thermal growth coefficient, feed conversion, and protein deposition of the fish attained best values with dietary protein levels of 40 and 44% at 18 and 26 °C water temperatures, respectively. The fish attained its maximum growth potential in terms of TWG, FCR, protein deposition, and body protein content at 26 °C water temperature. Carcass protein content exhibited best value for the groups fed 44% dietary protein at 26 °C temperature. Hematological parameters also attained their normal physiological range with 44% protein diet at 26 °C. Inferior values for these parameters were recorded for the groups held at 18 °C water temperature presumably due to the fact that the body metabolism occurs at a slower rate if fish are held at sub-optimum or lower water temperatures. Similar trend has also been reported by Peres and Oliva-Teles (1999) and Ozorio et al. (2006) in various other cultivable finfish species where fish held at 18 °C water temperature could not attain their maximum growth potential even if supplied with the required level of dietary protein. Growth performance and feed intake were significantly affected by both dietary protein levels and rearing temperature. However, interactive effects of dietary protein and temperature were not found. Depressed growth, lower feed intake, and protein deposition were more commonly noted for the groups reared at 18 °C. Even the groups fed dietary protein at 40 to 44% could not attain their maximum growth potential and feed intake at 18 °C as attained by the groups fed same diets at 26 °C. The study clearly indicates that dietary protein requirement of H. fossilis for maximizing the growth, feed conversion, and for attaining best values for hematological parameters ranges somewhere between 40.8 and 41.8% at 26 °C water temperature.
Choice of mathematical models in estimating the dietary level for a limiting nutrient is very important. Some studies show better regression coefficients when a broken-line analysis (Y = a + bX) is used (Baker 1986), whereas some respond better to a second-degree polynomial regression analysis (Tacon and Cowey 1985; El-Dakar et al. 2011). In this study, although data were fitted best for broken-line regression analysis, the p value of the t test for estimated coefficient was not found significantly different from zero for broken-line regression analysis. Therefore, second-degree polynomial regression analysis which exhibited a significant p value of the t test for the estimated coefficient has been employed for quantifying dietary protein requirement of H. fossilis fry. The requirements have been determined at 95% confidence interval. Based on above analyses, 44% dietary protein at 26 °C water temperature appears to be optimum for growth of H. fossilis fry and the curve did not reach a plateau until 44% dietary protein level. The second-degree polynomial fitting of protein deposition values at 95% maximum response exhibited optimum dietary protein requirement of fry H. fossilis between 40.8 and 41.8% (Fig. 1) at 26 °C water temperature. This level fall in the range of the previously reported dietary protein requirements of some other catfish species such as young H. fossilis 40–43% (Siddiqui and Khan 2009), Cyprinus carpio 41.25% (Ahmed and Maqbool 2017), higher than that for walking catfish, Clarias batrachus 36% (Singh et al. 2009), spotted snake-head, Channa punctatus 40% (Zehra and Khan 2012), and marbled spinefoot rabbitfish, Siganus rivulatus 40% (El-Dakar et al. 2011) and is lower than the requirements reported for African catfish, Clarias gariepinus 43% (Farhat 2011), Mystus nemurus 42% (Khan et al. 1993), Malaysian catfish, bagrid catfish, Mystus nemurus 44% (Ng et al. 2001), and striped murrel, Channa striatus 55% (Kumar et al. 2010).
The PD g/fish increased progressively with the increase in dietary protein up to 40% for the groups held at 18 °C and up to 44% for the groups held at 26 °C water temperatures, respectively. The PD/fish value attained by the groups reared at 18 °C was somewhat lower than that attained by the groups at the same level of dietary protein at 26 °C. This may probably be due to the reason that an increase in temperature at 26 °C might have increased the activity of digestive enzymes accelerating digestion of the nutrients, thus resulting in better growth (Shcherbina and Kazlauskene 1971) in the form of deposited protein. Hilge (1985) found that the optimum temperature for best growth of European catfish, Silurus glanis was almost within the range of 18 to 26 °C with best results noted at 27 °C. Brown et al. (1989) reported a 40% increase in growth rate of cod reared at 8.3 °C compared with 4.5 °C. This value was similar to that of Otterle et al. (1994), who reported an increase in growth rate of about 50% with each 4 °C increase in temperature between 6 and 14 °C. Protein deposition in this study was found to decrease for the groups fed dietary protein above 44% in diets D48 (48%) and D52 (52%) irrespective of the water temperatures. Proteins represent a very important source of energy in fishes. Since teleosts have developed the capacity for converting amino acid to glucose (Bever et al. 1981) by gluconeogenesis which is utilized for energy production through TCA cycle intermediates (Kumar 1999), it is reasonable to assume that the decline in protein deposition at higher levels of dietary protein for the groups fed diets D48 (48% protein) and D52 (52% protein) may probably be due to catabolism of excess protein for energy purposes thus reducing its deposition for tissue building or growth.
There are conflicting findings about the effect of dietary protein levels on the efficiency of protein utilization in the literatures. Lee et al. (2001) reported an increase in protein utilization efficiency with the increased intake of dietary protein by the fish, whereas Duan et al. (2001) and Lee et al. (2003) did not find any significant influence of dietary protein on efficiency of protein utilization. However, Kim et al. (2001), Kim and Lee (2009), and Gullu et al. (2008) pointed out a decrease in protein utilization with increasing dietary protein above optimum level which is in agreement with the present results. Davis and Stickney (1978) stated that fish convert protein more efficiently when fed dietary protein level less than optimal level that yields the maximum growth and feed efficiency. Steffens (1981) also reported that raising the dietary protein level improves the growth rate and food conversion but reduces the protein productive value in Salmo gairdneri and Cyprinus carpio. Similar findings were evident in this study where fish fed 48% and more protein manifested reduction in protein deposition.
The protein requirements or protein utilization of fish is also influenced by dietary non-protein energy levels (Dias et al. 1998; Lupatsch et al. 2001; Tibbetts et al. 2005; Wang et al. 2006). Hence, it is possible to reduce the dietary protein level to a certain degree by increasing non-protein energy and directing protein to growth rather than energetic use in a number of fish species (Forster and Hardy 2000). A protein-sparing effect is generally more pronounced at low protein levels rather than high levels (Dias et al. 1998; Tibbetts et al. 2005) mainly because of the preferential use of protein as an energy source by fish at high protein levels (Tibbetts et al. 2005). Cowey (1979) has also suggested that any change in dietary energy content changes the optimal protein requirement of the fish. Although in this study, the diets were formulated to be isocaloric and the digestible energy content of the diets was not significantly different (P > 0.05) among treatments, protein deposition decreased slightly with the increasing protein content and thus growth appears to be affected more by dietary protein levels than by energy levels. As per NRC (1993), the optimum P/DE values for fish range between 17 and 26 mg protein/kJ DE which in the present study, also almost corresponds to diets with 44% protein (27.21–27.88 mg protein/kJ DE) at 26 °C. Therefore, in this study, highest protein deposition with 44% dietary protein at 26 °C may be due to balanced P/DE ratio at this level of dietary protein.
H. fossilis fed intermediate levels of dietary protein (36–44%) exhibited higher feed intake than those fed still higher levels of protein in the diet (48–52%). This may probably be due to the reasons that fish fed nutrient-deficient diets usually increase the feed intake to meet the protein or the energy needs. Since the diets in this study were formulated to be isoenergetic, it is plausible that the fish fed intermediate levels of dietary protein might have consumed more feed in order to meet their protein requirements.
Temperature affects the body composition by altering feed intake (Jobling 1997) and various studies have shown that body protein is significantly affected by temperature (Cui and Wootton 1988; Koskela et al. 1997; Bendiksen et al. 2003; Tidwell et al. 2003). In this study as well, in addition to dietary protein levels, temperature also had significant influence on body protein and moisture contents of fry H. fossilis. The carcass quality of fish in terms of carcass protein content attained its superior value for the groups fed with 44% dietary protein at 26 °C temperature. Fish fed diets containing 28–36% protein tended to deposit more fat than those fed 40, 44, 48, and 52% dietary protein. In diets D28, D32, and D36, the carbohydrate contents increased at the expense of dietary protein which might have participated in de novo lipid synthesis from carbohydrate. Since these diets contain an improper ratio of protein to energy, this might have led to deposition of body fat from dietary carbohydrates.
Water temperature is one of the most important ecological factors that significantly influence some physiological process of fish such as growth, metabolism, and blood values. As has been shown in Table 4, hematocrit and hemoglobin concentrations were significantly (P < 0.05) altered by different water temperatures, However, interactive effects of dietary protein and temperature were not found. The results of this study are in line of the results reported by Koeypudsa and Jongjareanjai (2010) for hybrid catfish. Data related to hematological parameters in this study indicates that to sustain normal physiological processes in the body, H. fossilis should be held at 26 °C water temperature.
To study the effect of water temperature on the protein requirements of fish, Daniels and Robinson (1986) conducted two independent studies in which the red drum, Sciaenops ocellatus were maintained at 22–26 °C water temperature in the first and at 26–33 °C in the second. According to the authors, fish reared at lower temperature required less protein (35%) than those at higher temperature (44%). It is considered that water temperature affects feed intake and feed conversion efficiency (NRC 1993). Therefore, it is reasonable to assume that the suboptimal temperature in the present study might have deviated the feed intake in H. fossilis held at 18 °C and may be one of the reasons for reduced growth performance in groups held at this temperature; even if fed with the same dietary protein level.
Water temperature has substantial effect on fish metabolism. In response to decrease in water temperature, the enzyme activity of tissues increases (Hochachka and Somero 1984). In a stressful and unfavorable environmental condition ALT and AST activities may increase in blood serum. In the present study, serum ALT and AST levels were affected by different water temperatures. Serum ALT and AST amount in different fish fed varying levels of dietary protein at 26 °C are comparatively lower and attained normal physiological range at requirement level (44% dietary protein) than those fed at 18 °C. These results clearly indicated that 26 °C is the favorable water temperature for better growth of H. fossilis fry.
Survival rate in this study were not affected significantly by different levels of dietary protein but was more affected by temperature as the groups held at 18 °C had significant (P < 0.05) mortality at lowest level of dietary protein. On the other hand, mortality at 26 °C water temperature was not recoded even at the lowest level of dietary protein. The results are in line with various other finfish and shellfish studies (Li et al. 2011; Sun et al. 2015; Abdelrahman et al. 2019). Azaza et al. (2008) described that the survival rate of Nile tilapia, Oreochromis niloticus was significantly lower when it was reared at lower and upper level of its optimum water temperature.
Stress resistance of the fish in different life period is affected by levels of salinity, temperature, environment, and nutrition (Jalali et al. 2008; Gholami 2010). The results of present study showed that resistance rate to thermal stress significantly higher (P < 0.05) in fish fed dietary protein at 26 °C water temperature who were able to withstand temperature challenge for longer duration (Table 5) than those fish fed at 18 °C water temperature which were found to be more prone to temperature challenge test and exhibited mortality in comparatively less time.
Based on the 95% maximum response of second-degree polynomial regression analyses of PD g/fish data, it is recommended that fry H. fossilis could perform well if fed with dietary protein levels between 40.8 and 41.8% with a P/DE ratio of 27.21–27.88 mg protein/kJ DE at 26 °C water temperature. This study also corroborates that the performance of the fish and protein requirement was strictly governed by the rearing temperature as fish reared at 18 °C water temperature could not perform well in terms of growth, feed conversion, and protein deposition even if fed with the same level of dietary protein.
Conclusion
The information developed in the present study could be utilized for optimizing the growth potential of this fish by making better utilization of the nutrient at the 26 °C, the required temperature optima. The finding of the present study would further be useful for effective management strategies for the mass culture of this highly preferred fish species.