Background
Vibrio parahaemolyticus is a naturally occurring bacterium in estuarine and marine environments and the leading cause of seafood-borne illness in many countries including Korea (Oh et al. 2011; Haendiges et al. 2014; Elmahdi et al. 2016). V. parahaemolyticus is one of the most common causes of seafood-associated bacterial gastroenteritis in Korea, either directly or indirectly, by consuming infected seafood (Kang et al. 2016; Yang et al. 2017).
Fortunately, the majority of environmental V. parahaemolyticus strains are not pathogenic (Han et al. 2007; Elmahdi et al. 2016). In addition, very few V. parahaemolyticus isolates from aquaculture sources in Korea express the virulence genes tdh (thermostable direct hemolysin) and trh (tdh-related hemolysin) (Oh et al. 2011; Yu et al. 2014; Park et al. 2016; Yang et al. 2017). Nevertheless, according to the food poisoning statistics from 2003 to 2016 (KMFDS (Korea Ministry of Food and Drug Safety) 2017), this strain caused infections in 4256 patients, including 2876 patients during 2003–2007, 986 during 2008–2012, and 394 during 2013–2016.
Since the discovery of penicillin in the 1920s, hundreds of antibiotic agents have been developed and applied for clinical use or animal treatment (Aarestrup and Wegener 1999). Vibrio species are usually susceptible to most veterinary and human antimicrobials (Oliver 2006). However, several studies have reported that antibiotic resistance has emerged and evolved in many bacterial genera including Vibrio spp. during the past few decades due to excessive use of antibiotics in human, agriculture, and aquaculture systems (Mazel and Davies 1999; Cabello 2006). As a result of the misuse of antibiotics to control infections during aquaculture production, V. parahaemolyticus exhibits multiple antibiotic resistance, which has increased concerns about public health and the economic threat of this bacterium (Lesmana et al. 2001; Ottaviani et al. 2013; Al-Othrubi et al. 2014; Shaw et al. 2014; Kim et al. 2016a, b; Kang et al. 2017). Elmahdi et al. (2016) reported that both environmental and clinical isolates of V. parahaemolyticus show similar antibiotic resistance profiles. Antibiotic resistance within a wide range of infectious agents is a growing public health threat of broad concern to multiple countries and sectors (WHO (World Health Organization) 2014).
In Korea, the occurrence, presences of virulence genes, and multiple antibiotic resistance of V. parahaemolyticus in seafood and aquatic environments are particularly concerning in fish and shellfish farming and human health.
Food-borne outbreaks caused by V. parahaemolyticus in Korea
V. parahaemolyticus is one of the most common causes of seafood-borne illnesses in Korea. According to the food poisoning statistics from 2003 to 2016 (KMFDS (Korea Ministry of Food and Drug Safety) 2017), 225 cases and 4256 patients were reported in outbreaks caused by V. parahaemolyticus, accounting for 5.8% of total outbreaks and 4.1% of total patients, respectively, based on all food-borne illnesses occurring in Korea. This outbreak was ranked fourth in Korea.
This strain caused illness in 4256 patients from 2003 to 2016, including 2876 (300–732) patients during 2003–2007, 986 (106–329) during 2008–2012, and 394 (25–251) during 2013–2016 (see Fig. 1a). According to these data, the outbreaks associated with V. parahaemolyticus have been decreasing recently. In particular, the patient numbers caused by V. parahaemolyticus were remarkably low during 2013–2015 due to the decreases in the outbreak cases. It is assumed in the results that the Korean government further strengthens the publicity activities to reduce the seafood-borne outbreaks, especially during summer season. The rates of seafood-borne infections associated with V. parahaemolyticus increase during summer, especially between August and September, leading to 1355 patient infections (31.8%) and 1612 patient infections (37.9%), respectively (see Fig. 1b). In addition, according to the originating facility, the patient infection rate caused by V. parahaemolyticus was highest at restaurants (average 60.3%) with a range of 37.2–100.0% during 2003–2016 in Korea (Fig. 1a).
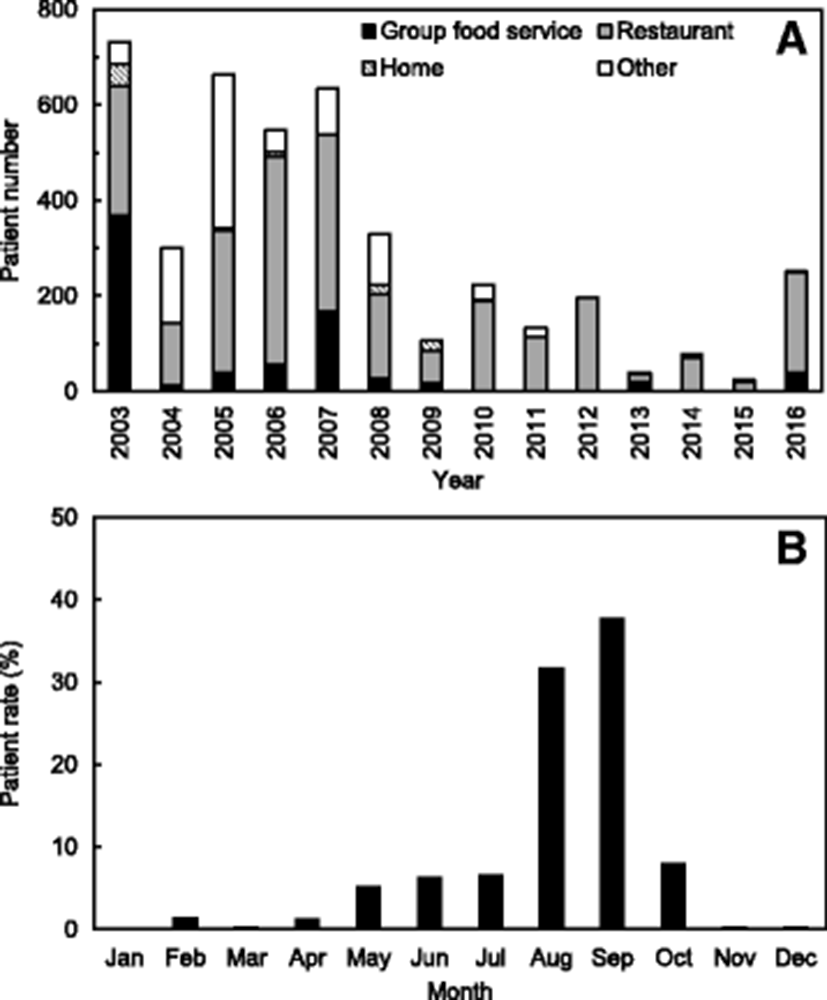
Distribution of V. parahaemolyticus isolates on the Korean coast
Table 1 and Fig. 2 summarize the distribution of V. parahaemolyticus isolated in seawater samples collected from the Korean coast, as reported in many studies. Presumptive strains of V. parahaemolyticus in the samples were isolated using the plates of thiosulfate citrate bile salt and then confirmed using the VITEK, API 20E, or polymerase chain reaction (PCR) systems. In some reported results, V. parahaemolyticus was enumerated by the most probable number method. Han et al. (2012) investigated the abundance of V. parahaemolyticus in 281 seawater samples collected from the west coast of Korea (Kyunggi province and Incheon city). According to their results, the level of V. parahaemolyticus was high in summer, especially in August (92.9%) followed by July (85.0%) and September (76.5%). Park et al. (2016) compared the distribution of V. parahaemolyticus in seawater samples (n = 429) collected from the same shellfish farms at each site along the south coast of Korea during 2013–2015. They reported that the detection rate of V. parahaemolyticus was also highest in August of each year; however, the strain was barely detectable from November to April of the following year. In addition, Yang et al. (2017) reported that V. parahaemolyticus was detectable in 9.7 and 32.9% of the Vibrio sp. isolates obtained from seawater and zooplankton samples in the Geoje coastal area of Korea during September 2016. V. parahaemolyticus was detected more frequently in mesozooplankton samples than in seawater samples.
Samples | Detection rate (%) (positive/total number) | References | |||||||||
---|---|---|---|---|---|---|---|---|---|---|---|
Site (year) | Type (number) | Positive number | Dec~Apr | May | Jun | Jul | Aug | Sep | Oct | Nov | |
Seawater | |||||||||||
Coast in Kyunggi and Incheon | Seawater (281) | 174 | 42.3a | 37.5a | 58.0a | 85.0a | 92.9a | 76.5a | 61.5a | – | Han et al. 2012 |
Shellfish farms in south coast (2013) | Seawater (152) | 9 | 0.0 (0/59) | 0.0 (0/13) | 0.0 (0/13) | 7.7 (1/13) | 35.7 (5/14) | 15.4 (2/13) | 0.0 (0/13) | 7.1 (1/14) | Park et al. 2016 |
Shellfish farms in south coast (2014) | Seawater (143) | 10 | 0.0 (0/61) | 8.3 (1/12) | 8.3 (1/12) | 0.0 (0/12) | 40.0 (4/10) | 33.3 (4/12) | 0.0 (0/12) | 0.0 (0/12) | Park et al. 2016 |
Shellfish farms in south coast (2015) | Seawater (134) | 21 | 2.1 (1/48) | 16.7 (2/12) | 8.3 (1/12) | 16.7 (2/12) | 66.7 (8/12) | 33.3 (4/12) | 23.1 (3/13) | 0.0 (0/13) | Park et al. 2016 |
Fish and shellfish | |||||||||||
Seafood markets in Busan and Gyeong Nam (2004) | Sea foods (213) | 65 | 0a | 6.3a | 9.1a | 50.0a | 66.5a | 64.5a | 41.5a | 18.0a | Kim et al. 2005 |
Seafood markets in Kyunggi and Incheon | Fish and shellfish (685) | 286 | 37.5a | 46.5a | 35.0a | 47.5a | 61.5a | 45.5a | 30.5a | – | Han et al. 2012 |
Seafood markets in Seoul (2005) | Oyster (72) | 48 | 0.0 (0/16) | 87.5 (7/8) | 100 (8/8) | 100 (8/8) | 100 (8/8) | 100 (8/8) | 100 (8/8) | 12.5 (1/8) | Lee et al. 2008 |
Shellfish farms in Geoje and Tongyeong Coast (2011) | Oyster (40) | 29 | – | – | – | 100 (8/8) | 87.5 (7/8) | 87.5 (7/8) | 50.0 (4/8) | 37.5 (3/8) | Yu et al. 2014 |
Gomso Bay (2011) | Shellfish (40) | 39 | – | – | – | 100 (8/8) | 100 (8/8) | 100 (8/8) | 100 (8/8) | 87.5 (7/8) | Yu et al. 2014 |
Shellfish farms in south coast (2013) | Shellfish (169) | 19 | 1.4 (1/73) | 6.3 (1/16) | 23.1 (3/13) | 16.7 (2/12) | 46.2 (6/13) | 30.8 (4/13) | 0.0 (0/16) | 11.8 (2/17) | Park et al. 2016 |
Shellfish farms in south coast (2014) | Shellfish (161) | 14 | 3.8 (3/79) | 0.0 (0/12) | 7.7 (1/13) | 7.7 (1/13) | 25.0 (3/12) | 36.4 (4/11) | 0.0 (0/10) | 12.5 (2/16) | Park et al. 2016 |
Shellfish farms in south coast (2015) | Shellfish (151) | 50 | 4.8 (3/62) | 15.4 (2/13) | 27.3 (3/11) | 72.7 (8/11) | 80.0 (8/10) | 69.2 (9/13) | 56.3 (9/16) | 50.0 (8/16) | Park et al. 2016 |
Shellfish farms in Incheon Coast (2014–2015) | Oyster (96) | 36 | 0.0 (0/40) | 0.0 (0/8) | 50 (4/8) | 100 (8/8) | 100 (8/8) | 100 (8/8) | 100 (8/8) | 0.0 (0/8) | Kim et al. 2016a |
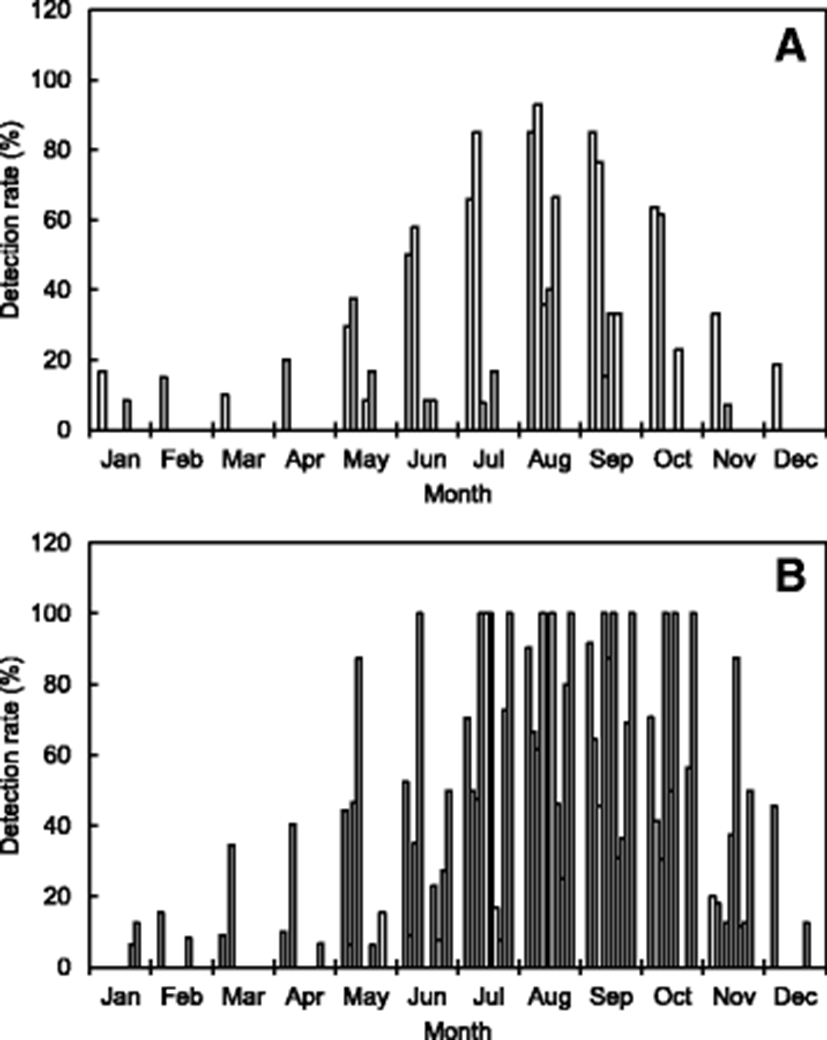
These results indicate that V. parahaemolyticus occurs at high levels during summer to early autumn in seawaters off the Korean coast, especially in July, August, and September. However, the strain was often detected very little during winter to early spring when the water temperature is low in the seawaters off the Korean coast (Table 1 and Fig. 2). Na et al. (2016) investigated the prevalence of pathogenic Vibrio species, including V. parahaemolyticus isolated from 2220 seawater samples during 2013–2015 in Korea and their relationship with marine environmental factors. According to their results, among the environmental factors, the correlation between seawater temperature and V. parahaemolyticus strains was the highest (R2 = 0.90).
Various studies have investigated the distribution of V. parahaemolyticus isolated in samples of shellfish and fish collected from the Korean coast (Table 1 and Fig. 1). Kim et al. (2005) investigated the abundance of V. parahaemolyticus in 213 seafood samples, including sliced raw fish and living marine invertebrates (oysters, ascidians, and sea cucumbers) collected from seafood markets and restaurants in Busan city and Kyeongnam province of Korea. According to their results, V. parahaemolyticus was detected at a range of 41.5–66.5% from July to October, but its incidence was < 20% during the other months. The strain isolation rate (49.2%) in raw marine invertebrates including shellfish was higher (28.9%) than that in sliced raw fish. Another study was conducted in samples (n = 685) of fish and shellfish collected from retail seafood markets in Kyunggi province and Incheon city, Korea. In that study, the monthly detection rate of V. parahaemolyticus ranged from 30.5 to 61.5%; the highest level was found in August (Han et al. 2012).
In addition, most shellfish samples (including oysters) collected from seafood markets and shellfish farms in Korea also contain relatively high levels of V. parahaemolyticus during summer and early autumn (Lee et al. 2008; Yu et al. 2014; Kim et al. 2016a; Park et al. 2016). However, V. parahaemolyticus was often detected at very low rates during winter and early spring in low water temperatures off the Korean coast.
These results show that the seasonal variation and cycle are correlated with water temperature, which is a major factor affecting the abundance of V. parahaemolyticus (Sudha et al. 2014). Some previous studies have reported a positive correlation between water temperature and V. parahaemolyticus counts (DePaola et al. 2003). The outbreaks associated with V. parahaemolyticus in Korea appear to be seasonally dependent because 31.8 and 37.9% of patient infections occurred in August and September during 2003–2016, respectively (Fig. 1b). The Korean results also show seasonal variations in the occurrence of V. parahaemolyticus in the samples of seawater, fish, and shellfish (Table 1 and Fig. 2).
Virulence genes of V. parahaemolyticus isolates in Korea
Virulence of V. parahaemolyticus has been attributed to the presence of the tdh and trh toxic genes using the PCR (Nishibuchi et al. 1985). The tdh and trh genes are widely considered predominant indicators of virulence in V. parahaemolyticus (Su and Liu 2007; Shimohata and Takahashi 2010). Honda and Iida (1993) reported that only 1–2% of environmental V. parahaemolyticus strains normally produce the tdh gene.
In this article, some V. parahaemolyticus isolates were investigated for the presence of virulence genes (tdh and trh) by PCR system. The expected size of the amplified DNA was 251 bp for the tdh gene and 250 bp for the trh gene, respectively. Oh et al. (2009) conducted a study to investigate the presence of the tdh and trh toxic genes in V. parahaemolyticus isolated from brackish water samples collected off the south coast of Korea, such as Geoje city, Tongyeong city, and Goseong Gun (Table 2). According to their results, 23 of 177 isolates were positive for the tdh gene, whereas all strains tested negative for the trh gene. On the other hand, many studies have shown that all V. parahaemolyticus stains isolated from seawater samples collected from the Korean coast were negative for the tdh and trh virulence genes (Lee and Park 2010; Kim et al. 2014, 2016a, b; Park et al. 2016). Yang et al. (2017) reported that all 30 V. parahaemolyticus strains and mesozooplankton samples isolated from seawater were negative for the tdh and trh virulence genes. Therefore, these reports indicate that all V. parahaemolyticus stains isolated from seawater samples collected off the Korean coast are negative for the tdh and trh virulence genes; however, the tdh gene was detected in 13.0% of isolates from brackish water samples collected from sites located in the mouths of streams (Table 2).
Samples | Number of isolates | Virulence genes (%) | Resistant (%)a | References | ||
---|---|---|---|---|---|---|
Site (year) | Type (number) | Antimicrobials used | Three or more of antimicrobials | |||
Water | ||||||
Fish farms in south coast (2004) | Seawater (60) | 145 | – | AM (98.0), OA (30.6), AN (26.5), AMC (20.4), TE (12.2), CIP (6.1), D (4.1), CEP (2.0), CTX and SXT (0.0) | 15.2 (22/145) | Son et al. 2005 |
Coast in Geoje, Tongyeong, and Goseong (2005) | Brackish water (27) | 177 | tdh (13), trh (0) | AM (85.9), RA (26.6), AN (16.4), S (13.6), TMP (13.0), E (3.4), GM (1.1), AMC, TE, CIP, CEP, CTX, SXT, C, and NA (0.0) | 20.3 (36/177) | Oh et al. 2009 |
West coast (2006) | Seawater (22) | 21 | – | AM (100), CF (85.7), AN (81.0), FOX (38.1), GM (9.5), AMC (4.8), CIP, CTX, SXT, and C (0.0) | – | Lee et al. 2009 |
Shellfish farms in Gomso Bay (2008) | Seawater (−) | 28 | tdh (0), trh (0) | – | – | Lee and Park 2010 |
Shellfish farms in Wando Coast (2011–2012) | Seawater (−) | 67 | tdh (0), trh(0) | AM (100), OX (100), VA (64.2), S (56.7), AN (31.3), K (22.4) CF (20.9), E (10.4), CIP (4.5), TE (3.0), SXT, TMP, GM, C, and NA (0.0) | 88.1 (59/67) | Kim et al. 2014 |
Shellfish farms in south coast (2013–2015) | Seawater (429) | 37 | tdh (0), trh (0 | – | – | Park et al. 2016 |
Shellfish farms in Gomso Bay (2014–2015) | Seawater (100) | 79 | tdh (0), trh (0) | OX (100), VA (100), P (100), AM (96.2), E (10.1), S (7.6), FOX (6.3), AN (2.5), CF (2.5), TE, CIP, CEP, CTX, SXT, RA, TMP, GM, C, NA, K, CTT, and CZ (0.0) | 100 (79/79) | Kim et al. 2016b |
Fish | ||||||
Fish farms in south coast (2004) | Fish (66) | 49 | – | AM (97.9), OA (24.1), AN (17.2), TE (4.8), D (4.1), CIP (2.8), AMC (1.4), CTX and SXT (0.7), CEP (0.0) | 38.8 (16/49) | Son et al. 2005 |
Fish farms in south coast (2005–2007) | Fish (180) | 218 | tdh (5.5), trh (0.5) | AM (57.8), RA (11.9), S (8.7), TMP (6.4), TE and C (3.7), AN and NA (2.8), GM (1.8), CEP and SXT (1.4), CTX and E (0.9), CIP (0.5), AMC (0.0) | 10.1 (22/218) | Oh et al. 2011 |
Shellfish | ||||||
Shellfish farms in Geoje and Tongyeong Coast (2011) | Oyster (40) | 129 | tdh (0), trh (0) | AM (79.1), TMP (3.1), S (1.6), CTX, SXT, RA, GM, and NA (0.8), AN, AMC, TE, CIP, CEP, E, and C (0.0) | 1.6 (2/129) | Yu et al. 2014 |
Shellfish farms in Gomso Bay (2011) | Short neck clam (40) | 307 | tdh (0), trh (0) | AM (61.0), TMP (5.2), S (2.9), RA (2.3), GM and NA (1.0), C (0.3), AN, AMC, TE, CIP, CEP, CTX, SXT, and E (0.0) | 3.6 (11/307) | Yu et al. 2014 |
Shellfish farms in south coast (2013–2015) | Shellfish (481) | 84 | tdh (2.4), trh (9.5) | – | – | Park et al. 2016 |
Shellfish farms in Incheon Coast (2014) | Oyster (15) | 71 | tdh (0.0), trh (53.5) | AM (100), S (50.7), CF (52.2), RA (50.7), GM (2.9), E (15.5), CIP (2.8), TE, CEP, CTX, SXT, C, and NA (0.0) | 87.3 (62/71) | Kang et al. 2016 |
Shellfish farms in Incheon Coast (2014–2015) | Oyster (96) | 115 | tdh (0.0), trh (9.6) | AM (94.8), S (60.0), CF (53.9), RA (47.8), E (13.9), GM (7.0), CIP (4.4), CEP(3.5), NA (1.7), TE (0.9), K (0.4), CTX, SXT, C, and CTT (0.0) | 46.1 (53/115) | Kim et al. 2016a |
Shellfish farms in Incheon Coast (2015) | Oyster (16) | 44 | tdh (0.0), trh (9.1) | AM (86.4), S (75.0), CF (56.8), RA (43.2), GM (13.6), E (11.4), CIP (6.8), NA (4.5), TE (2.3), CEP, CTX, SXT, and C (0.0) | 77.3 (25/44) | Kang et al. 2017 |
Other | ||||||
Seafood markets in Busan and Gyeong Nam (2004) | Seafood (213) | 65 | – | AM (96.9), AN (29.2), TE (27.7), CTX (9.2), AMC, CIP, CEP, and SXT (0.0) | – | Kim et al. 2005 |
Coast and seafood markets in Kyunggi and Incheon | Seawater (281) Fish and shellfish (685) | 716 | – | VA(97.3), AM (87.3), CF (48.8), RA (46.1), E (28.7), NA(16.5), TE (13.5), CTX, SXT, GM, C, K, and NN (< 10) | 68.7 (492/716) | Han et al. 2012 |
Geoje Coast (2016) | Zooplank-ton (14) Seawater (14) | 24 6 | tdh (0.0), trh (0.0) tdh (0.0), trh (0.0) | AM (100), PIP (90.0), RA (73.3), CF (73.4), CZ (66.7), TMP (10), AN (6.7), CIP and SXT (3.3), TE, AMC, CEP, CTX, S, GM, C, NA, and CTT (0.0) | 93.3 (28/30) | Yang et al. 2017 |
AM ampicillin, OA oxolinic acid, AN amikacin, AMC amoxicillin/clavulanic acid, TE tetracycline, CIP ciprofloxacin, D doxycycline, CEP cefepime, CTX cefotaxime, SXT sulfamethoxazole-trimethoprim, RA rifampin, S streptomycin, TMP trimethoprim, E erythromycin, GM gentamicin, C chloramphenicol, NA nalidixic acid, CF cephalothin, FOX cefoxitin, OX oxacillin, VA vancomycin, K kanamycin, P penicillin, CTT cefotetan, CZ cefazolin, NN tobramycin, PIP piperacillin
aResistance was classified according to guidelines of the Clinical and Laboratory Standards Institute (CLSI)
In addition, Oh et al. (2011) carried out a study to determine the virulence genes of 215 V. parahaemolyticus isolates from farmed fish in Korea. Of them, 12 (5.5%) and 1 (0.5%) strains were positive for the tdh and trh genes, respectively. Finally, according to various shellfish studies of Korean coastal shellfish farms, some V. parahaemolyticus isolates do not possess the tdh and trh genes (Yu et al. 2014), and a few were positive for only the trh gene with a range of 9.1–53.5% (Kang et al. 2016, 2017; Kim et al. 2016a, b). Another Korean study reported that 2 (2.4%) and 8 (9.5%) of 84 strains isolated from shellfish samples were positive for the tdh and trh genes, respectively (Park et al. 2016). Therefore, some V. parahaemolyticus isolates from cultured shellfish and fish do rarely possess both genes, and a few were positive for only the trh gene (Table 2).
Antibiotic resistance profiles of V. parahaemolyticus isolates in Korea
Pathogenic bacteria with antimicrobial resistance genes are often released with wastewater discharge into the aquatic environment (Baquero et al. 2008), indicating that naturally occurring aquatic bacteria including V. parahaemolyticus are capable of serving as reservoirs for resistance genes. Many studies have investigated antibiotic resistance of V. parahaemolyticus in water samples from the Korean coast (Table 2). The recommended antibiotics to treat V. parahaemolyticus illnesses are tetracycline or ciprofloxacin (CDC (Centers for Disease Control and Prevention) 2013). Some Korean studies that were carried out to characterize the antibiotic susceptibility profile of V. parahaemolyticus isolates in seawater samples indicated that 3.0–12.2% of isolates were resistant to tetracycline and ciprofloxacin (Son et al. 2005; Kim et al. 2014).
A study was carried out in 2004 on the antibiotic resistance of 145 V. parahaemolyticus isolates collected from seawater samples at fish farms on the south coast of Korea (Son et al. 2005). Vibrio spp. were resistant to the majority of antibiotics tested (2.0–98%), except for cefotaxime and sulfamethoxazole-trimethoprim, with the highest resistance found for ampicillin. About 15 of the isolates were resistant to three or more antibiotic agents, including tetracycline or ciprofloxacin, which are usually prescribed for V. parahaemolyticus infections.
Oh et al. (2009) investigated the antibiotic susceptibility of V. parahaemolyticus by analyzing the toxigenic and non-toxigenic strains isolated from brackish water samples on the south coast of Korea, such as in Geoje city, Tongyeong city, and Goseong Gun, to 15 antibiotics. A total of 177 V. parahaemolyticus strains were analyzed including 13% of the isolates with the tdh gene. No significant difference was observed in the distribution of multiple resistances with respect to pathogenic potential, and 20.3% of all strains were resistant to three or more classes of antibiotics. Some strains were resistant to ampicillin (85.9%), rifampin (26.6%), amikacin (16.4%), streptomycin (13.6%), trimethoprim (13.0%), erythromycin (3.4%), and gentamicin (1.1%).
Another study was conducted to investigate the susceptibility patterns to different antibiotic agents of 67 V. parahaemolyticus isolates in seawater samples from shellfish farms on the Wando coast of Korea. All isolates (100%) were resistant to ampicillin and oxolinic acid, and 88.1% of all strains were resistant to three or more classes of antibiotics. However, all isolates were sensitive to sulfamethoxazole-trimethoprim, trimethoprim, gentamicin, chloramphenicol, and nalidixic acid (Kim et al. 2014).
Similarly, Kim et al. (2016a, b) reported that all V. parahaemolyticus strains (79 isolates), isolated in seawater samples from shellfish farms in Gomso Bay, Korea, were resistant to oxolinic acid, vancomycin, and penicillin, and 96.2% of all isolates also demonstrated resistance to ampicillin. Unexpectedly, all isolates were resistant to three or more classes of antibiotics.
These reports indicate that V. parahaemolyticus isolates collected from water samples on the Korean coast show very high resistance to ampicillin, accounting for 85.9–100% (see Table 2). A very high ampicillin resistance rate was observed in V. parahaemolyticus in the USA as early as 1978, even before the recognition of Vibrio vulnificus as a food-borne pathogen (Blake et al. 1979).
Forty-nine isolates of V. parahaemolyticus were obtained from fish at different fish farms on the south coast of Korea and tested for their susceptibility to various antibiotics. The highest incidence of antibiotic resistance was reported against ampicillin (97.9%) followed by oxolinic acid (24.1%) and amikacin (17.2%). Among these isolates, 100 and 38.8% showed resistance to at least one and three of the ten tested antibiotics, respectively (Son et al. 2005). Oh et al. (2011) also investigated the antibiotic resistance of V. parahaemolyticus isolated from fish farms on the south coast of Korea, where 57% of all isolates were resistant to ampicillin, and 65.1% of the isolates were resistant to at least one of the 15 tested antibiotics.
Yu et al. (2014) compared the incidence of antibiotic resistance of V. parahaemolyticus strains isolated from oysters and short-neck clams in each shellfish-growing area of the Korean coast. Of the 129 and 307 isolates from each shellfish species, 79.1 and 61.0% were resistant to ampicillin, respectively, which was the highest of the tested antibiotics. Multiple resistance to at least three antibiotics was relatively low with a range of 1.6–3.6%. Kang et al. (2016, 2017) and Kim et al. (2016a, b) conducted an antibiotic resistance study of V. parahaemolyticus isolates from oysters on shellfish farms located on the Incheon coast of Korea during 2014–2015. Their results indicate that the highest resistance in each study was against ampicillin (86.4–100%) followed by streptomycin (50.7–75.7%), cephalothin (52.2–56.8%), and rifampin (43.2–50.7%). Among these isolates, 46.1–87.3% were resistant to at least three of the tested antibiotics.
Kim et al. (2005) tested the antibiotic resistance of V. parahaemolyticus isolated in 213 seafood samples, including sliced raw fish and living marine invertebrates from different seafood markets and restaurants in Busan city and Kyeongnam province Korea. V. parahaemolyticus isolates were resistant to ampicillin (96.9%), amikacin (29.2%), and tetracycline (27.7%).
Han et al. (2012) determined the antibiotic resistance profile of 716 V. parahaemolyticus strains isolated from samples of seawater, fish, and shellfish on seven coastal areas of Kyunggi province and Incheon city, Korea. According to their results, 97.3 and 87.3% of the isolates showed very high resistance to vancomycin and ampicillin, respectively, followed by cephalothin (48.8%) and rifampin (46.1%). In addition, 68.7% of the isolates presented multiple resistance to at least 3 of the 13 antibiotics tested, including vancomycin and ampicillin. Two exhibited resistance to 11 antibiotics used in this study. However, about 92% of these isolates were highly susceptible to sulfamethoxazole-trimethoprim and chloramphenicol. They also found high sensitivity to gentamicin (82.3%), tobramycin (74.8%), nalidixic acid (71.6%), tetracycline (69.4%), and cefotaxime (63.0%).
Yang et al. (2017) characterized the antimicrobial resistance profile of V. parahaemolyticus isolated from seawater and zooplankton samples in the Geoje coastal area Korea, which is an important area for coastal fisheries, the fishing industry, and tourism. All V. parahaemolyticus isolates were resistant to ampicillin, and 66.7–90.0% exhibited resistance to rifampin, cephalothin, cefazolin, and piperacillin. In addition, 93.3% of the isolates were resistant to three or more antimicrobial agents. This was the first antimicrobial susceptibility data of V. parahaemolyticus recovered from zooplankton samples in Korea.
Several studies have reported that V. parahaemolyticus isolates collected from samples of seawater, fish, shellfish, and zooplankton in Korea have a very high resistance to ampicillin of 57.8–100% (see Table 2). Similarly, a very high ampicillin resistance rate is also observed in V. parahaemolyticus isolated from marine environments and seafood in many countries, including the USA (Elmahdi et al. 2016).
Tetracycline has long been the most commonly used antibiotic in Korean fisheries, particularly to treat heavy infections caused by Vibrio species (Morris and Tenney 1985; Oh et al. 2011). The recommended antibiotics for treating V. parahaemolyticus illnesses are tetracycline and ciprofloxacin (CDC (Centers for Disease Control and Prevention) 2013; Elmahdi et al. 2016). Unfortunately, although a low percentage of tetracycline resistance to V. parahaemolyticus was observed, some V. parahaemolyticus isolates developed resistance to tetracycline (see Table 2).
In addition, many studies have reported high multiple antibiotic resistance in V. parahaemolyticus isolates from samples of water, fish, and shellfish on the Korean coast (Table 2).
Conclusions
V. parahaemolyticus is a naturally occurring bacterium in estuarine and marine environments and a leading cause of seafood-borne illness. Outbreaks associated with V. parahaemolyticus in Korea appear to be seasonally dependent because 69.7% of patient infections occurred in August and September during 2003–2016. In addition, the occurrence of V. parahaemolyticus increased during summer and early autumn in the samples of seawater, fish, and shellfish from the Korean coast, especially in July, August, and September.
All V. parahaemolyticus isolates in the seawater samples from the Korean coast were negative for the tdh and trh virulence genes; however, the tdh gene was detected in 13.0% of isolates from brackish water samples in the stream estuary areas. V. parahaemolyticus isolates from aquaculture production (shellfish and fish) showed various patterns associated with the virulence genes; some did not possess either the tdh or trh gene, whereas a few were positive for only the trh gene or both genes.
Our findings suggest that ampicillin has been excluded as a treatment option for infections caused by V. parahaemolyticus, because the strains from aquaculture sources (e.g., seawater, fish, shellfish, and zooplankton) in Korea have very high resistance to ampicillin, which ranged from 57.8 to100% of the isolates. In addition, multiple resistance to three or more antimicrobial agents were high with a range of 10.1–100% in V. parahaemolyticus isolates from samples of water, fish, and shellfish off the Korean coast, except two shellfish sampling areas (1.6–3.6%). These findings indicate that a high incidence of antimicrobial resistance of V. parahaemolyticus in marine environments is due to exposure to conventionally used antibiotics.
Therefore, monitoring the distribution and antimicrobial susceptibility of V. parahaemolyticus strains in seafood and marine environments, with surveys expanded to a national level, is needed to ensure seafood safety. Moreover, the development of new alternative methods (e.g., probiotics and natural antimicrobials) is urgently needed to reduce antibiotic use in fish and shellfish farming and to avoid threats to public health caused by massive misuse of antibiotics.