Background
Starting from the late 1980’s, soft tunic syndrome (STS) has become one of the most notorious nuisances in Korean ascidian culture industry (Kim et al. 2014). The disease is now known to be caused by a protozoan pathogen Azumiobodo hoyamushi (A. hoyamushi). This parasite resides within the tunic tissue leading to disintegration of the cellulose-based exoskeleton structure of the ascidian (Kumagai et al. 2010, 2011; Kim et al. 2014). It has been reported that the parasite is opportunistic in plaguing the cultured ascidian, but the pathogen has also been reported to be present in other similar marine animals.
While the disease is severely affecting ascidian industry to the extent that even its sustainability is threatened, still there is no effective measure to control the disease. One way that can be considered to resolve this predicament is to utilize various currently available biocidal agents. In our previous report (Park et al. 2014), it was demonstrated that several biocidal drugs are effective in killing the causative protozoa both in vivo and in vitro. Judging simply from the potency and toxicity of drugs tested, several drugs including formalin, hydrogen peroxide (H2O2) and bronopol, in single treatments, were found promising deserving detailed researches.
In the present study, we examined the co-treatment effects of drugs which are singly efficacious in killing the parasites. When two agents are simultaneously applied to kill pathogens, one agent can influence the efficacy of the other (Klaasen 2001). What is ideal for treatment is a synergistic treatment activity with accompanying minimal toxicity to the host. To be synergistic two drugs need to act on different effector systems or exert by different action mechanisms (Mutschuler and Derendorf 1995). In contrast, however, if the two drugs scavenge each other, the net effect can be lower than their arithmetic sum.
In view of the weakness that most non-specific disinfectants have narrow margin of safety (Maris 1995), we aimed to examine combinational effects of anti-parasitic agents with a hope to identify synergistic activities. Effects were evaluated both in pure cultured parasites A. hoyamushi in vitro, and also in artificially infected ascidians in vivo to delineate the applicability of developed methods in the farm.
Methods
Chloramine-T hydrate (chloramine), bronopol, povodone-iodine (poly-vinylpyrrrolidine-iodine complex, PVV-I) were purchased from Sigma Co. (St. Louis, MO, USA). Formaldyhyde solution (37 %) and hydrogen peroxide (35 %) were obtained from Junsei Co. (Tokyo, Japan). All other main chemicals not specified were also obtained from Sigma.
The pathogenic parasite A. hoyamushi was isolated from tunics of diseased ascidians as described previously (Park et al. 2014). Isolated pathogens were incubated at 15 °C in minimum essential medium (MEM) supplemented with fetal bovine serum, glutamine, penicillin, sea salt, streptomycin, and HEPES buffer. Prepared medium was filtered through 0.45 μm filter papers.
Pure culture of A hoyamushi was grown in MEM for 3–4 days to the cell density of about 1 × 108/ml. After adjustment to 5 × 105 cells/ml, the culture was added to 12-well plates in 1-ml volume and incubated with test drug solutions at different concentrations and exposure periods. To estimate parasite-killing effects of drugs, 200 cells were randomly counted with a reverse phase microscopy at 400-fold magnification.
Apparently healthy ascidians weighing about 70 g were transported from a culture farm located in Tongyong City, Kyungnam Province, Korea. They were kept in the laboratory at 15 °C and 30.5 psu for 1 week to acclimatize. Cultured parasites of 1.0 × 107 cells were added into the 100-l ascidian-containing aquaria and maintained until mild symptoms (Kimura scale, Kitamura et al. 2010) developed in about 20 % of organisms (took about 3 days). Thirty individuals each were randomly allocated to 20-L aquaria in the absence and presence of test drugs. Exposures were performed only once on day 1 at necessary drug concentrations and exposure periods. Mortality of the ascidians was monitored for 14 days thereafter. During the exposure, number of live parasites in the tunics was estimated with some ascidians. This experiment was performed in two separate sets of experiments due to difficulties of obtaining non-infected colonies: the first was with 50 and 100 ppm of combined drugs and the second with 20 ppm. The whole procedure of in vivo assessments was conducted according to the Ethics Codes of the Animal Experiment Approval Committee, Kunsan National University, Korea.
Both healthy and diseased ascidians were transported from culture farms of different locations in Tongyong City. Healthy individuals (30 individuals, about 80 g body weight) were maintained with six severe grade ascidians (about 80 g). The healthy ascidians were kept hanging with the ropes similarly to the situation used in the farm. The ropes were about 50 cm long and were perpendicularly maintained at 15 °C. When about 20 % of animals exhibited mild STS signs, ascidians were immersed into the drug bath for 1 h on day 1 before starting monitoring mortality. At the termination of mortality observation, the number of live parasites in the tunic was estimated.
The method was basically identical to that described previously (Park et al., 2014). In short, ten individuals were randomly selected from each group and the whole tunic tissue was isolated. The tunics were immersed in 20-ml filter-sterilized sea water and incubated for 24 h at 15 °C. Total live cell counting was made using a hemacytometer and light microscope.
Results
Based on previous anti-parasitic effects (Park et al. 2014) of various drugs, formalin, H2O2 and bronoplol, chloramines-T (referred to chloramines) and povidone-iodine (PVP-I) were selected for combinational potency measurements. Figure 1 illustrates in vitro parasite-killing effects of test drugs when combined with formalin and treated for 60 min. In the single 5 ppm exposure with formalin, hydrogen peroxide (H2O2), bronopol, chloramine and PVP-I, all drugs exerted parasite-killing effects of 8–31 %. However, combinations of formalin + H2O2 or formalin + bronopol exhibited strong synergistic effects. Synergistic effects were not observed in formalin + chloramine or formalin + povidone-iodine combinations.
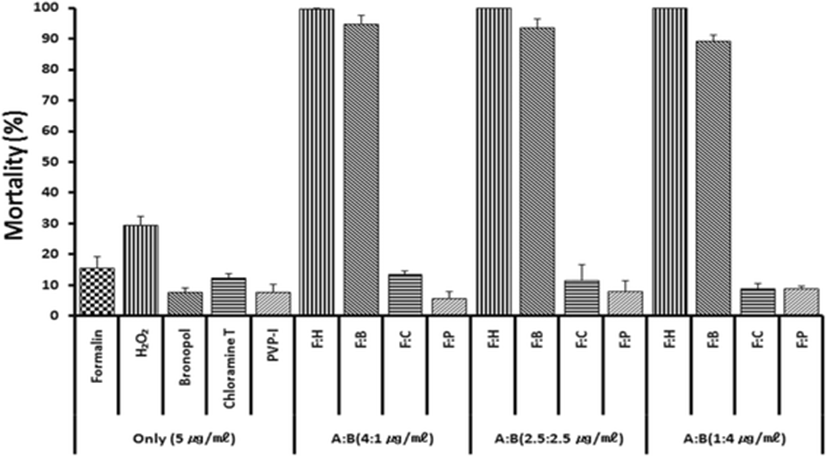
Exposure period of 60 min is likely to be an obstacle in on-site field applications, and thus it must be better if possible to shorten it. In order to evaluate shorter exposures, parasites were exposed in vitro for 20–60 min with 4:1 ppm standard combinations of formalin-H2O2 or formalin-bronopol. As results in Fig. 2a indicate, 20–40 min exposure could only exert a slightly compromized activity indicating that 60 min is the requirement with 4 ppm.
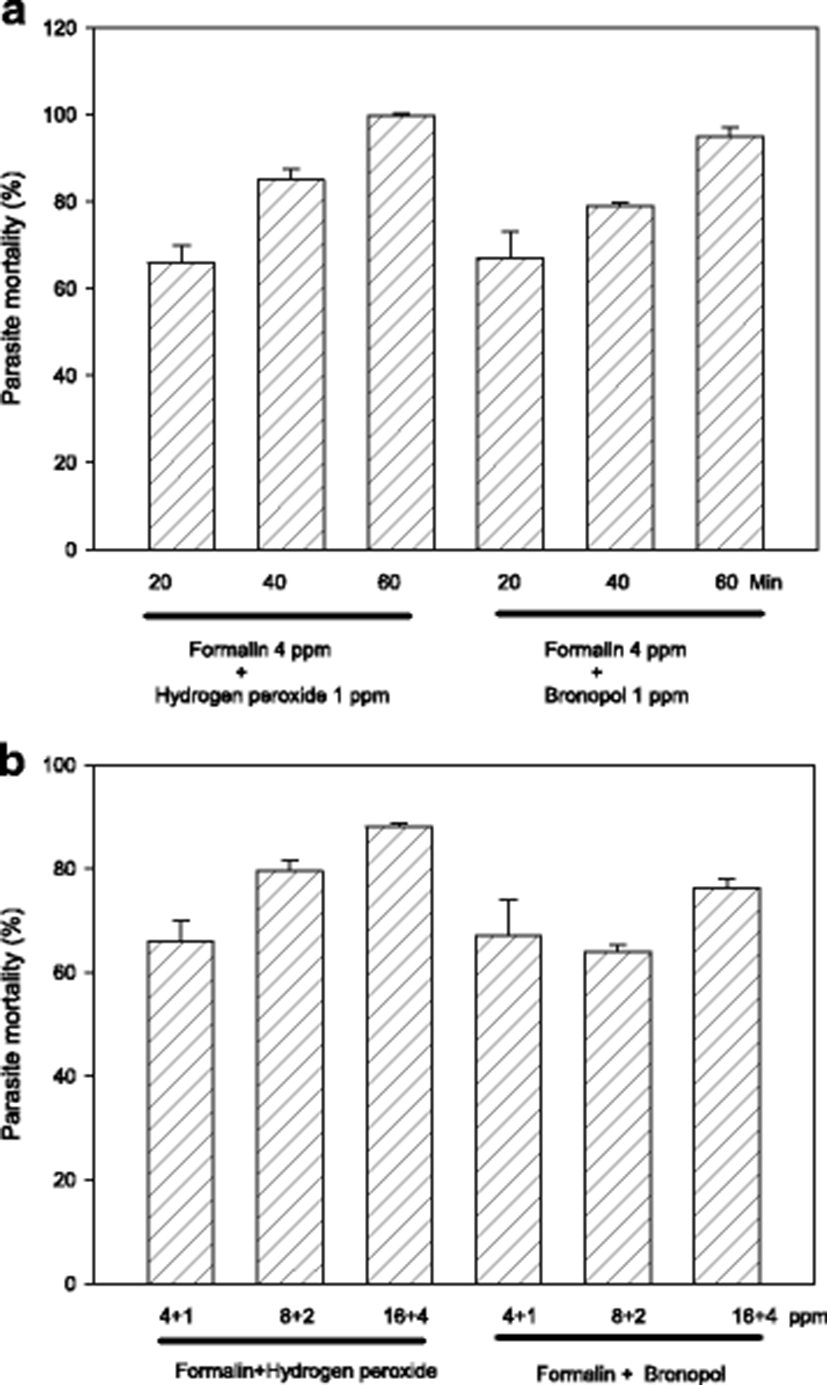
Another option to compensate the submaximal efficacy of shorter drug exposure can be increasing drug concentrations. When formalin-H2O2 (or bronopol) concentration was escalated from 5 ppm (total) to 20 ppm, generally there was a concentration-dependent increase in parasiticidal activity (Fig. 2b). In adition, exposure with 10 ppm was more effective than 5 ppm with formalin-H2O2 but not with formalin-bronopol.
After infection with pure cultured parasites, ascidians were bathed for 1 h in formalin-H2O2 combinations at 20, 50 and 100 ppm (total). Figure 3 illustrates drug effects on mortality after an artificial infection with parasites to non-infected, healthy ascidians. As the tests were performed in two separate sets, the control mortalities were not identical being that of control in 20 ppm was slightly higher than in 50 and 100 ppm.
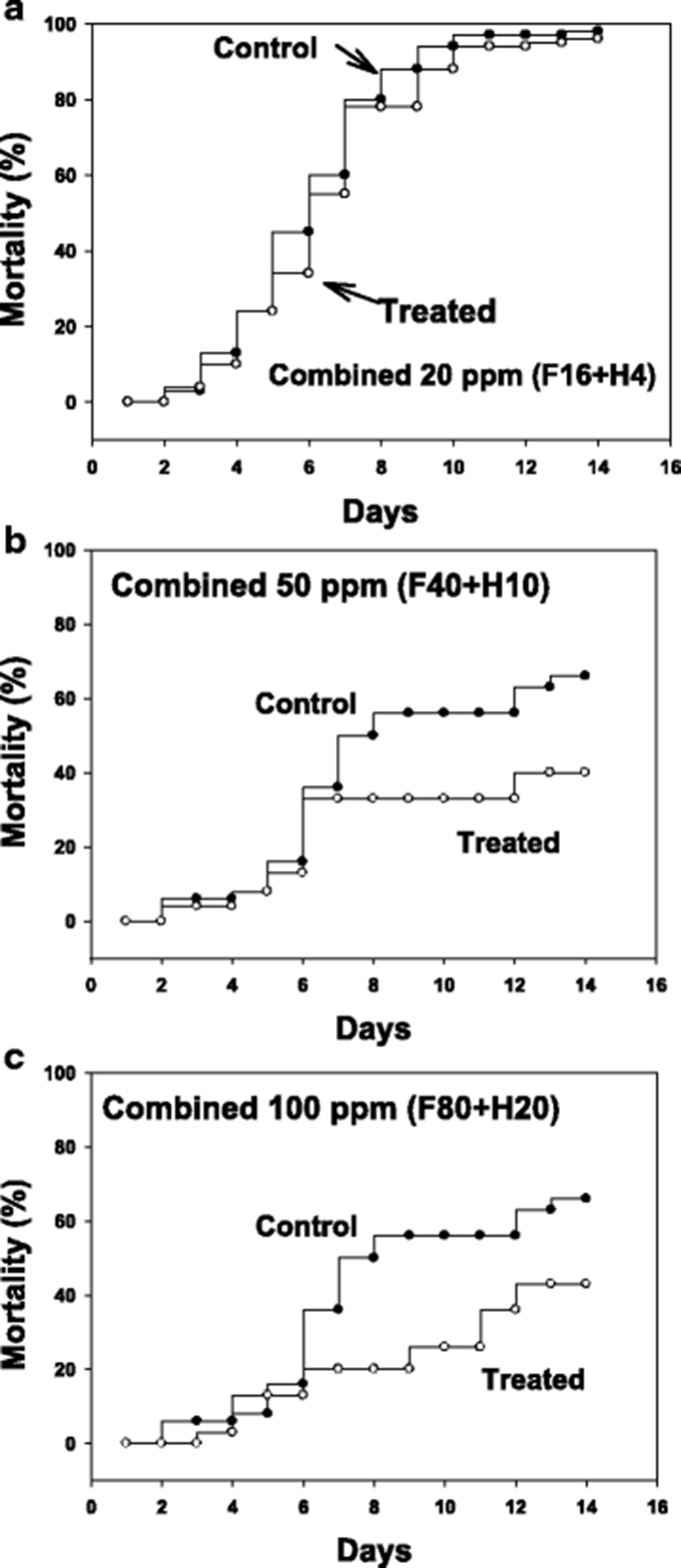
The starting time point for drug testing was when there was a clear appearance of STS signs in about 20 % of animals. Clear reduction in ascidian mortality was obtained with 50 (Fig. 3b) and 100 ppm (Fig. 3c), but not with 20 ppm (Fig. 3a). During the mortality observation period of 14 days, number of live parasites in the tunic tissue was enumerated. It was observed that there were about an average of 20,000 parasites/ascidian on day 5 from the start of monitoring, and the number increased significantly on day 10 to about 80,000 without treatment (see Fig. 4, Control columns). These live parasites were almost completely removed when ascidians were bathed with 50 ppm of formalin-H2O2 once for 1 h on day 1 (Fig. 4, Treated columns).
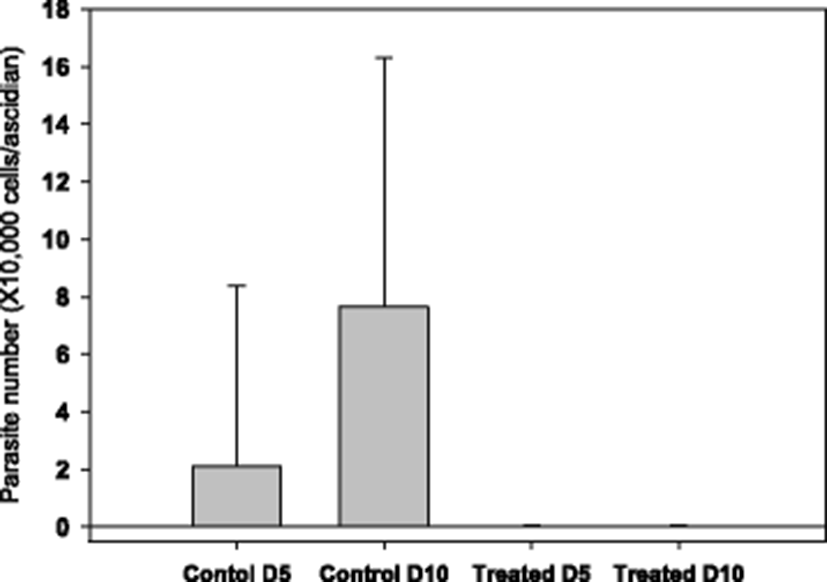
Similar in vivo anti-parasitic effects were identified in another STS model in which infection was achieved by co-incubation with diseased individuals. In the two independent trials with 55–60 ascidians in each treatment group, formalin-H2O2-treated groups (50 ppm total) demonstrated apparently better survivals (Fig. 5). While the mortality was above 80 % without treatment by day 14, mortalities of about 30 % (Trial #1) and 5 % (Trial #2) were respectively observed. In this horizontal transmission model, too, significant reduction in live parasites was confirmed (right-sided two panels in Fig. 5).
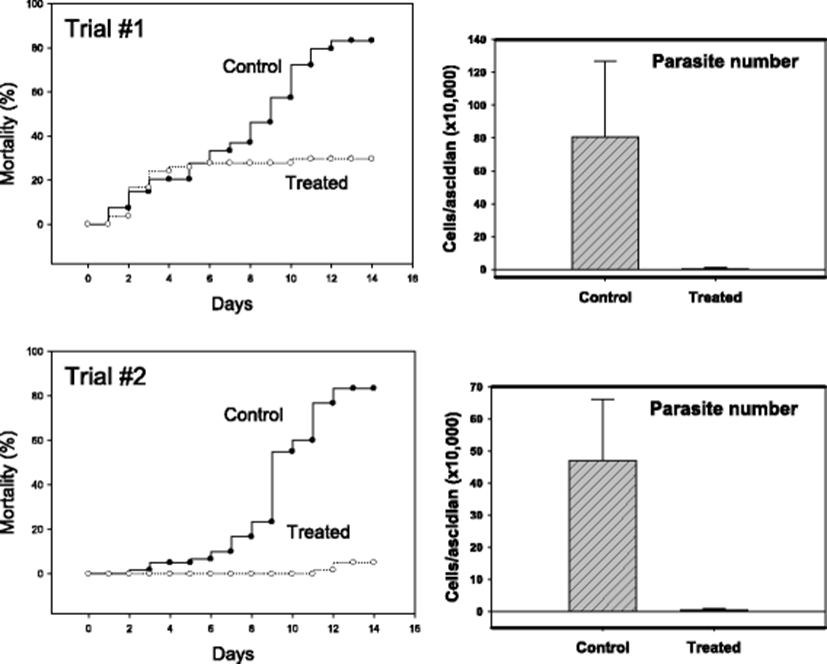
Discussion
It is urgently required to invent methods to control the spread of STS disease, the one most devastating cause currently impacting ascidian (squirt) industry. Different methods have been explored in the pursuit of resolving the predicament, and some examples are farm rotation (Kim et al. 2014), genetic selection of more tolerant individuals (Cho et al. 2008) and present trials to move farms to the far open sea areas. Treatment of the culture animals with biocidal drugs can be considered as one option for this purpose.
In this study, it was assessed whether dual biocide treatment can be a better choice to increase efficacy of the drugs. The choice of test drugs were based on two criteria: (1) effectiveness of drugs in single treatment from previous studies (Park et al. 2014; Lee et al. 2013); (2) availability and legal uses for aquacultural purposes. Dual treatment has not been often adopted in using non-specific biocides in contrast to the frequent uses of antibiotics to achieve synergistic advantage in aquaculture (Smith 2012). Only limited reports are available which suggest synergism in biocidal drug use use. Synergistic anti-parasitic effects were demonstrated when formalin and malachite green were simultaneously treated against the protozoal pathogen Tetrtahymena pyriformis (Gilbert et al. 1979). Combination of sulfonamides with neomycin also exerted potentiated effects against the protozoal parasite Labyrinthuloides haliotidis in abalone (Bower 1989). In addition, synergistic activities were identified in the combinations of formalin + bronopol or formalin + MBT (a cactus extract) against the salmon egg-infecting fungus (Jee and Lee 2009).
Formalin is one of the most widely used non-specific biocides worldwide to treat aquatic pathogens including protozoa (Treves-Brown 2000). This disinfectant is approved for olive flounder and eggs by bath treatments in Korea. Bronopol (bromo-2-nitropropane-1,3-diol) is effective against protozoan parasites, such such as Icthyophthirius multifiliis (Shinn et al. 2012a, b). This drug has not, however, been introduced to Korean aquaculture, yet. Due to its quick decomposition into water and gaseous oxygen after application, H2O2 is very safe to culture animals and human consumers (Treves-Brown 2000). This drug is used in general to sanitize culture water. H2O2 has shown promising results in treating different protozoan parasites (Harms 1996; Russo et al. 2007). In this study, synergistic actions were identified with formalin-H2O2 or formalin-bronopol combinations. No clear synergism was observed with formalin-chloramine and formalin-PVP-I combinations.
Synergistic activities are closely related to action mechanisms of the two drugs. To be synergistic, one drug should enhance the binding of another drug to target sites, or enhance transport to target areas. However, it is almost impossible to expect the occurrence of synergism in a straightforward way because numerous factors are involved until eventual death of parasites occurs (Bell 2005). Experimental demonstration is always needed.
Formalin (formaldehyde) denatures vital biomolecules working as a bridge irreversiblly by liking two amine groups between adjacent molecules (Mason and O’Leary 1991). While bronopol causes membrane damage in organisms through the inhibition of membrane bound enzymes (Stretton and Manson 1973). On the other hand, H2O2 releases reactive oxygen species to denature proteins and lipids in the organisms (Maris 1995). The dissimilarity in mechanisms involved in the combinations of formalin-H2O2 and formalin-bronopol seemed to satisfy necessary conditions for synergism. From unknown reasons, yet, synergism was not observed with chloramine and PVP-I, both of which commonly produce reactive halogen radicals as their action mechanism (McDonnell and Russell 1999).
From the current finding, it was found important to keep the bathing time of 60 min. This can be a barrier in field use because of a long operation time. Methods to facilitate the onset of drug actions seem to be needed: elevation of bath water temperature can be one plausible option, since this change will accelerate the absorption rate of drugs. This method is, at least however, applicable in disinfecting brood stock treatments in inland seed production facilities.
Conclusions
Conclusively, the suggested measure based on this study does not constitute an ultimate tool to control the STS in the field. It is also needed to refine methods to minimize adverse impacts on the ascidians being treated and on the marine environments. Despite all such limitations and accompanying legal restrictions, the results obtained for this study will be useful as one guide to develop field applicable methods against the currently helpless STS situations.