Introduction
Oxidative stress is a common cause of neurodegenerative diseases like Alzheimer’s diseases (AD), which are the leading causes of death worldwide (Singh et al., 2019). Excessive quantities of reactive oxygen species (ROS), a cellular metabolism consequence, play a critical role in neurodegeneration (Zorov et al., 2014). The brain uses a large amount of oxygen to produce energy; owing to the low antioxidant levels and many polyunsaturated fatty acids in the brain tissue, it is vulnerable to ROS-induced oxidative damage (Uttara et al., 2009). Increased oxidative stress in the brain has been reported to be closely associated with cognitive decline in aged mice, where ROS modifies amyloid beta (Aβ) and hyperphosphorylation of tau (p-Tau) protein, two critical biomarkers of AD brains (Liu et al., 2015; Logan et al., 2019). Many studies have reported glycogen synthase kinase-3 beta (GSK3β) activity to be directly related to increased levels of Aβ, p-tau, and synaptic damages in AD patients and in rodent models (DaRocha-Souto et al., 2012) where p-tau, increased by GSK3β, modulates the level of acetylcholinesterase (AChE) (Cortés-Gómez et al., 2021). Presence of large amounts of AChE is a common feature of AD brain and is associated with Aβ and neurofibrillary tangles (NFT). Aβ aggravation and abnormal p-tau levels influence AChE expression and increase neurotoxicity (García-Ayllón et al., 2011). Activity of AChE in blood and plasma can be used to assess AD pathophysiology, since plasma AChE activity is increased in patients with AD (Carvajal & Inestrosa, 2011). In AD, memory and learning are impaired due to the suppression of brain-derived neurotrophic factor (BDNF)/tropomyosin receptor kinase B (TrkB) signaling (Zhang et al., 2016). In addition, some data shows that Aβ reduces BDNF activation via reducing phosphorylated cyclic adenosine monophosphate (cAMP) response element binding protein (CREB) protein, and phosphorylation of GSK3 at serine-129 leads to CREB deactivation which causes dysregulation in BDNF/TrkB signaling (Amidfar et al., 2020; Grimes & Jope, 2001).
Capsosiphon fulvescens (CF) is a green seaweed that has been traditionally used as functional food material to cure gastrointestinal problems and hangovers in Korea (Critchley et al., 1998). CF approximately contains 13% ash, 1% lipids, 30% proteins and 55% polysaccharides along with essential macro and micro minerals, such as Ca, Fe, Mg, Na, K, and P. It exhibits strong 2,2-diphenyl-1-picrylhydrazyl (DPPH) and nitrite scavenging activities (Asanka Sanjeewa et al., 2018). Fang et al. (2012) had reported nine compounds from 95% EtOH extract of CF that exhibited AChE inhibitory activity associated with neuroprotective properties (Fang et al., 2012). Oh & Nam (2019) had reported that hydrophilic glycoproteins of CF protect aging-induced spatial memory impairment in rat dorsal hippocampus (Oh & Nam, 2019). However, very few studies on the neuroprotective mechanism of CF have been conducted till date. The objective is to determine the neuroprotective properties of CF.
Materials and Methods
Materials, such as 3-(4,5-Dimethylthiazol-2yl)-2,5-Diphenyltetrazolium-12-Bromide (MTT), 2′,7′-dichlorofluorescein diacetate (DCFH-DA), 10-nonyl bromide acridine orange hydrochloride solution, Triton X-10, ethylenediaminetetraacetic acid (EDTA), aluminum chloride (AlCl3), and hydrogen peroxide, were from Sigma-Aldrich (St. Louis, MO, USA). Roswell Park Memorial Institute (RPMI) 1640 medium, fetal bovine serum, phosphate-buffered saline (PBS), trypsin, and penicillin-streptomycin were obtained from Hyclone (Logan, UT, USA). Sodium phosphate (NaHPO4), sodium chloride (NaCl), methanol (MeOH), and ethanol (EtOH) were obtained from DUKSAN (Ansan, Korea).
Fresh CF was collected from Jeollanam-do province, South Korea. A voucher specimen was deposited in the herbarium of the Department of Nutraceutical Resources, Mokpo National University, Republic of Korea. CF extracts were produced with subcritical water and several solvents (water, MeOH, and EtOH) to investigate the neuroprotective potential. Fresh CF was thoroughly washed with tap water to remove excess salt. For solvent extraction, 6 g of CF were dissolved in 200 mL of 100% MeOH, 200 mL of 100% EtOH, and 200 mL of water separately. The extracts were filtered, evaporated under vacuum, then freeze-dried for up to 72 h to yield 0.9 g of dry MeOH extract (CFM; 15% yield), 0.05 g of dry EtOH extract (CFE; 0.83% yield), and 1.12 g of water extract powder (CFW; 18.7% yield). For the subcritical water extract preparation, hydrolysis of CF was performed at various temperatures (90°C, 150°C, and 210°C), labelled as CF90, CF150, and CF210. For each temperature, 6 g of CF were soaked in an extraction cell with 250 mL of distilled water, and the botanical extraction equipment (TPR-1, Taiatsu Techno, Tokyo, Japan) was maintained at a constant reaction pressure of 3 MPa for 1 min. The extracts were then filtered and freeze-dried for up to 72 h to yield 1.43 g of CF90 (23.8% yield), 2.09 g of CF150 (34.8% yield), and 2.82 g of CF210 powder (47% yield).
PC12 neuronal cells were obtained from the American Type Culture Collection (Manassas, VA, USA) and cultured in RPMI 1640 medium supplemented with 10% fetal bovine serum at 37°C under 5% CO2. During the experiment, the medium was replaced every two days. When the cells achieved 75%–90% confluence, they were seeded in culture plates.
PC12 cells were seeded in 96-well plates at a density of 2 × 104 cells/well. They were treated with different concentrations of CF extract for 2 h and further treated with H2O2 and incubated for 24 h at 37°C and 5% CO2. Cell cytotoxicity was observed using the MTT solution (250 μg/mL). After 3 h, the MTT solution was removed followed by addition of dimethyl sulfoxide, to dissolve the purple formazan product formed by living cells. The optical density was then measured at a wavelength of 540 nm using a spectrophotometer (Immuno Mini NJ-2300, Biotech, Tokyo, Japan).
The effect of H2O2 on intracellular ROS production was evaluated by culturing PC12 cells in black 96-well plates for 48 h at a density of 2 × 104 cells/well, and then treated with CM-H2DCFDA (10 μM) in growth medium for 30 min. The cells were then washed with PBS and treated with CF extracts for 2 h, followed by treatment with H2O2 (0.25 mM) for 24 h. The cells were subsequently washed with PBS solution, and fluorescence intensity was measured at excitation/emission wavelengths of 485 nm/520 nm using a fluorescence microplate reader (Perkin-Elmer, Waltham, MA, USA).
PC12 cells were seeded in 6-well plates at a density of 0.5 × 104 cells/well for 24 h. They were treated with CF extracts for 2 h, followed by treatment with H2O2 (0.25 mM) for 24 h at 37°C and 5% CO2. The cell extracts were prepared by adding high-ionic strength buffer (10 mM NaHPO4, 1 M NaCl, 10% Triton X-10, and 1 mM EDTA) and centrifuged at 12,000 rpm for 20 min 4°C. The collected supernatant was used to determine the AChE activity.
PC12 cells were cultured in black 96-well plates at a density of 2 × 104 cells/well for 48 h. They were treated with CF extracts for 2 h, followed by treatment with H2O2 (0.25 mM) for 24 h. Thereafter, the culture medium was removed, and the calcium-sensitive fluorescent dye Fluo-3 AM (5 μM) was added by mixing with fresh culture medium for 30 min. After incubation, the cells were washed with PBS, and the intensity was measured at excitation/emission wavelengths of 490 nm/520 nm using a fluorescence microplate reader (Perkin-Elmer, Waltham, MA, USA).
Adult Zebrafish (Danio rerio, AB line) were in-house cultured in a 14-h light/10-h dark cycle with 28 ± 0.5°C and pH 7.0 in a continuously flow-through system (ZebTEC, Tecniplast, Buguggiate, Italy) in the laboratory at Mokpo National University. According to their developmental stage, GEMMA Micro feeds (Skretting; Tooele, UT, USA) of various sizes were supplied to the zebrafish. Adult male and female zebrafish were placed in mating tanks at 1:1 ratio, and embryos were collected the following day. Embryos were cultured in a 96-well cultural plate with E2 embryo medium (15 mM NaCl, 0.5 mM KCl, 1 mM CaCl2, 1 mM MgSO4, 0.15 mM KH2PO4, 0.05 mM Na2HPO4, and 0.7 mM NaHCO3, pH 7.1–7.4) until 6 days’ post fertilization (dpf). At 6 dpf, the zebrafish larvae were exposed to the different concentrations of CF extracts and incubated for 24 h. Viability and morphological changes of the larvae in response to AlCl3 and CF extract exposure were observed under a LEICA MZ10F microscope (Leica, Wetzlar, Germany) by confirming the relative shape and size of the eye, yolk sac, jaw, tail, etc., compared to the normal group, and the observed malformations and mortality rates were calculated.
AlCl3 was used as an inducer of ROS production in zebrafish larvae, as AlCl3 exposure increases the production of free radicals, disrupts the mitochondria, and ultimately leads to oxidative stress and neurodegeneration (Kaur et al., 2022). The latter were exposed to different concentrations of AlCl3 (12.5, 25, 50, 100, and 250 μM) after 4 dpf. AlCl3 powder was dissolved in distilled water, and the final concentration was prepared by diluting with E2 medium. The treatment was continued up to 8 dpf, after which, the AlCl3 solution was treated every 2 d until the experiments were completed.
Based on the method proposed by Park et al. (2021), DCFH-DA reagent was used to stain nucleic acids for measuring intracellular ROS production (Park et al., 2021). After 8 dpf, the solution of DCFH-DA (20 μg/mL) was added to each well of the culture plate and allowed in the dark for 1 h at 28°C, respectively. When the experiment was completed, the zebrafish larvae were washed twice or thrice with embryo medium and used 0.5% MS-222 to anesthetized. Images were obtained using a fluorescence microscope (Leica). Fluorescence intensity of each zebrafish larva was quantified using the ImageJ software (National Institutes of Health, Bethesda, MD, USA).
Total RNA was extracted from pools of 40–50 larvae using a Bullet Blender homogenizer and TRIzol reagent (Molecular Research Center, Cincinnati, OH, USA). RNA primers were purchased from Integrated DNA Technologies (Coralville, IA, USA). Quantitative PCR (qPCR) assays were conducted with the Applied Biosystems StepOneTM Real-Time PCR System Thermal Cycling Block (ABI, Los Angeles, CA, USA) using a SYBR Green Real-Time qPCR Kit (BIOFACTTM, Daejeon, Korea). Sequences of the oligonucleotide primers were as follows: glutathione peroxide (GPx)–forward: 5′-TGAGAAGGGTTTACGCATCC-3′ and reverse: 5′-GCTGCTCCTTCATCCACTTC-3′; superoxide dismutase (SOD)–forward: 5′- ATGGTGAACAAGGCCGTTTG-3′ and reverse: 5′- AAAGCATGGACGTGGAAACC-3′; GSK3β–forward: 5′-GCCACAGAAACCAAGAGAGC-3′ and reverse: 5′-GAATGCAAACGGAAGTGGAT-3′; AChE–forward: 5′-GGGCACCAGGTTTCAGTAAA-3′ and reverse: 5′-CTTTTGGCCGTTGTTCTCAT-3′; BDNF–forward: 5′-CGAGGAATAGACAAGCGGCA-3′ and reverse: 5′-ATCCGTATAAACCGCCAGCC-3′; Trkβ–forward: 5′-TCACCTATGGCAAGCAACCC-3′ and reverse: 5′-CTTTGGGGCAAGTACGAGGT-3′; Aβ–forward: 5′- AGCAGCTGGTGGAGACTCAT-3′ and reverse: 5′-TAGTGGCGGATAGTGTGCTG-3′; and Tau (tau)–forward: 5′-ACTGAGTTCTGGGAGCGA AA-3′ and reverse: 5′-CAAATGGGAATGTTGCTGTG-3′. The qPCR reaction conditions were: 15 min at 95°C followed by 40 cycles of 15 s at 95°C and 45 s at 60°C, followed by plate analysis. In each run, the positive and negative controls were included. The number of gene copies was calculated using the Ct value and standard curve and the significance analysis was performed on the log2 transformed values that were obtained via analysis of variance (ANOVA).
Results
Crude CF extracts at different concentrations (125, 250, and 500 μg/mL) were added to normal PC12 cells and incubated for 24 h; no cytotoxicity was observed, as shown in Fig. 1. Almost all CF extracts showed significant cell proliferation at lower concentrations than at higher concentrations compared to that in the normal group.
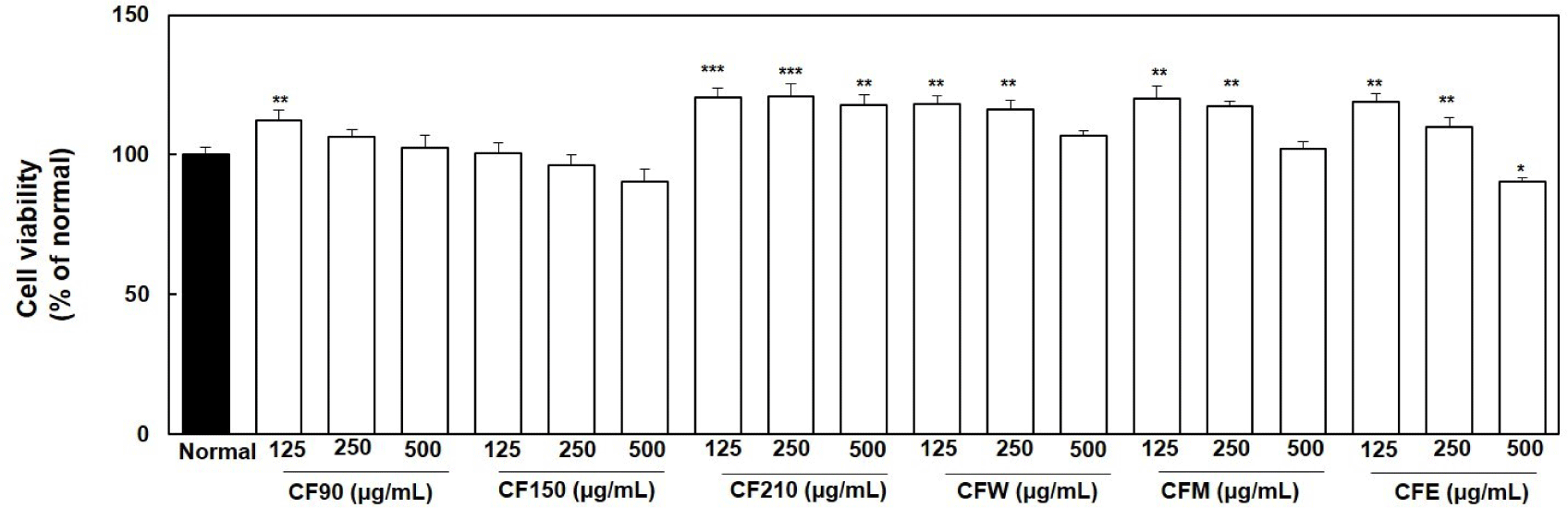
As shown in Fig. 2A, PC12 cells were treated with different concentrations of H2O2. Based on the results of previous studies, we used concentrations ranging from 0.125, 0.5, 1, 2, and 4 mM to evaluate the cytotoxicity of H2O2-induced oxidative stress. Cytotoxicity, according to H2O2 concentration, in PC12 cells was 81.6%, 49.4%, 36.4%, 36.1%, 7.2%, and 2.0% respectively. With reference to previous reports, we selected 0.25 mM concentration to analyze the neuroprotective effects of CF extracts; we found that CF90, CF150, CF210, and CFW extracts showed preventive effect against H2O2 toxicity in PC12 cells (Fig. 2B). Among them, cell viability of PC12 cells was the highest with CF90 and CFW extracts. Therefore, only two extracts were used in subsequent experiments.
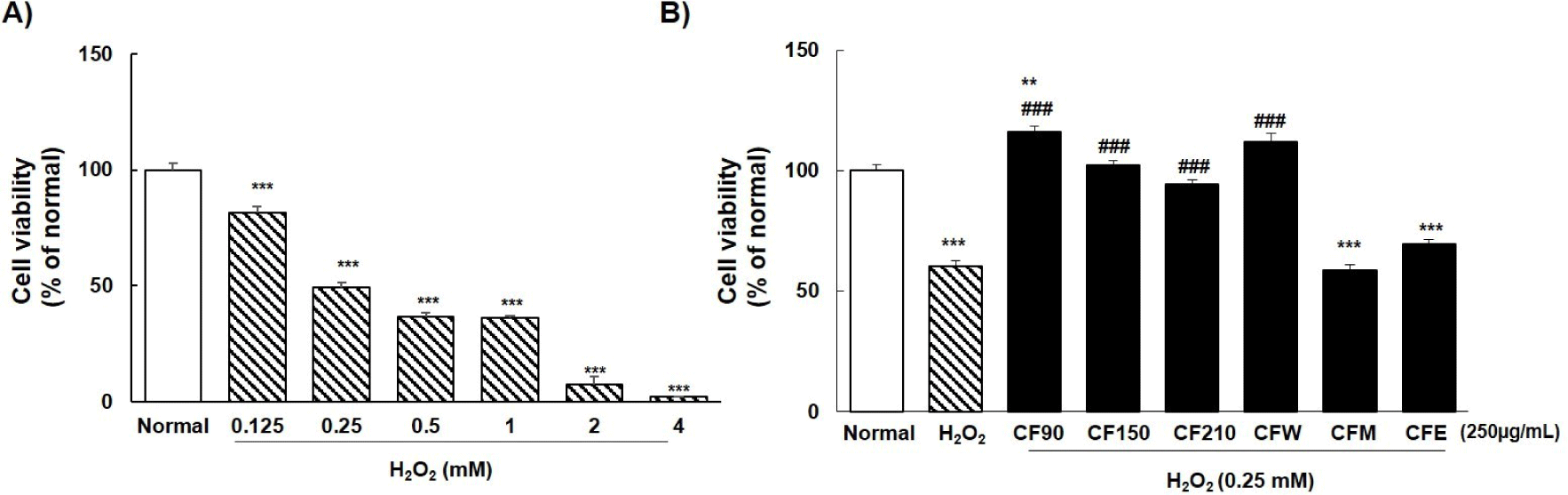
ROS generation in PC12 cells due to H2O2 exposure was significantly (p < 0.001) increased by approximately 76% compared to that in the normal group (Fig. 3A). It decreased effectively in a concentration-dependent manner by the addition of CF90 or CFW extract. However, there was no significant difference between the two groups (CF150 and CF210). Excess AChE enzyme activity and intracellular calcium levels, which are markers of neurodegeneration, were significantly increased to approximately 23% and 20% by 0.25 mM H2O2 treatment compared to that in normal PC12 cells (Fig. 3B and 3C). Addition of CF90 and CFW extracts significantly reduced H2O2-stimulated AChE activity and calcium concentration in a concentration-dependent manner.
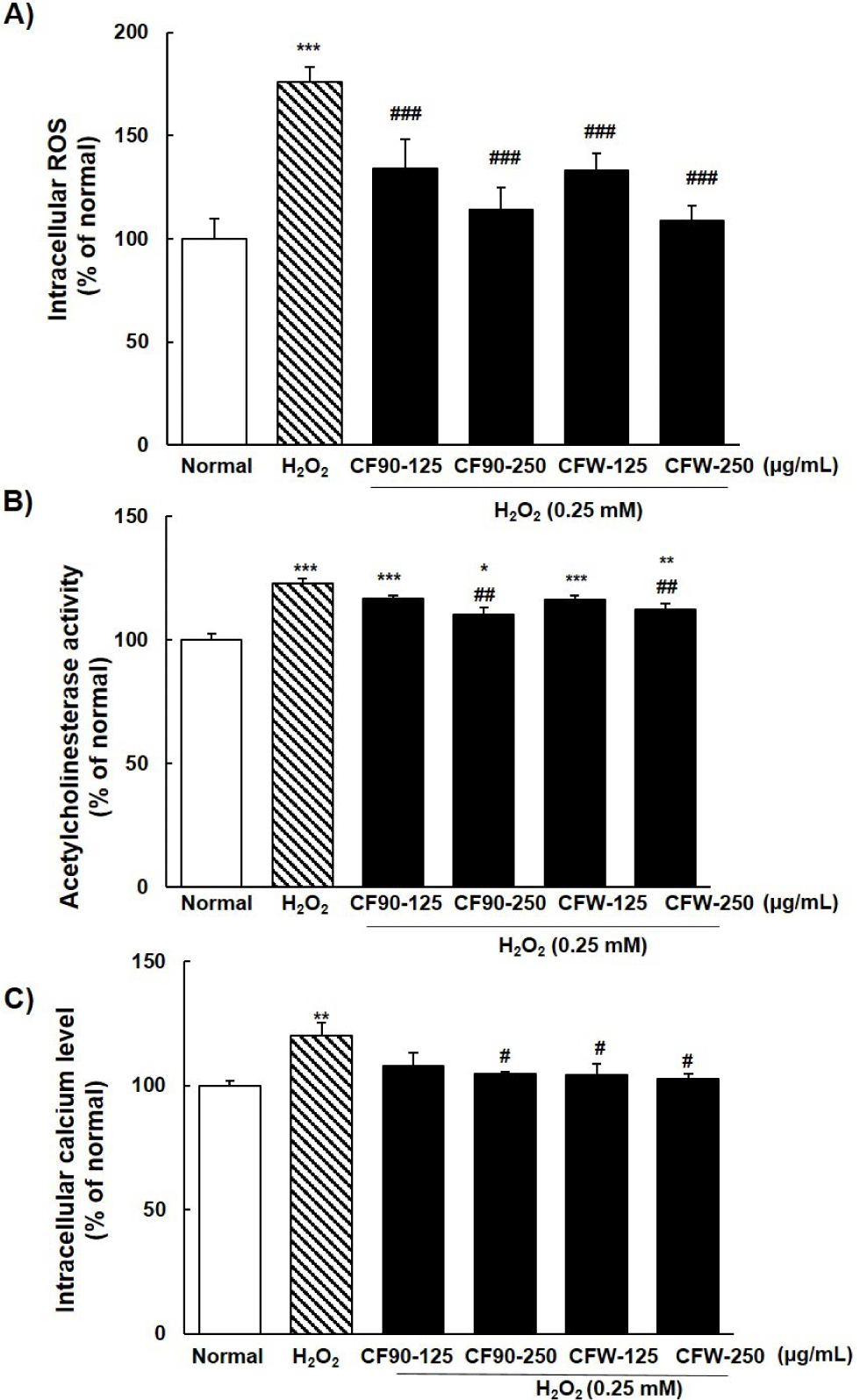
To confirm the neuroprotective effects of CF, we designed an AlCl3-induced neurotoxicity model in zebrafish larvae (Fig. 4). When zebrafish larvae were treated with different concentrations of AlCl3 (12.5, 25, 50, 100, and 250 μM) for 2 days, no toxicity was observed up to 250 μM AlCl3 (Fig. 4A). At a concentration of 25 μM AlCl3, ROS production increased to approximately 19% than in the normal group (Fig. 4B and 4C), which was mainly observed in the liver and gastrointestinal region of the zebrafish larvae. The most suitable concentration for AlCl3-induced neurotoxicity in zebrafish larvae was 25 μM.
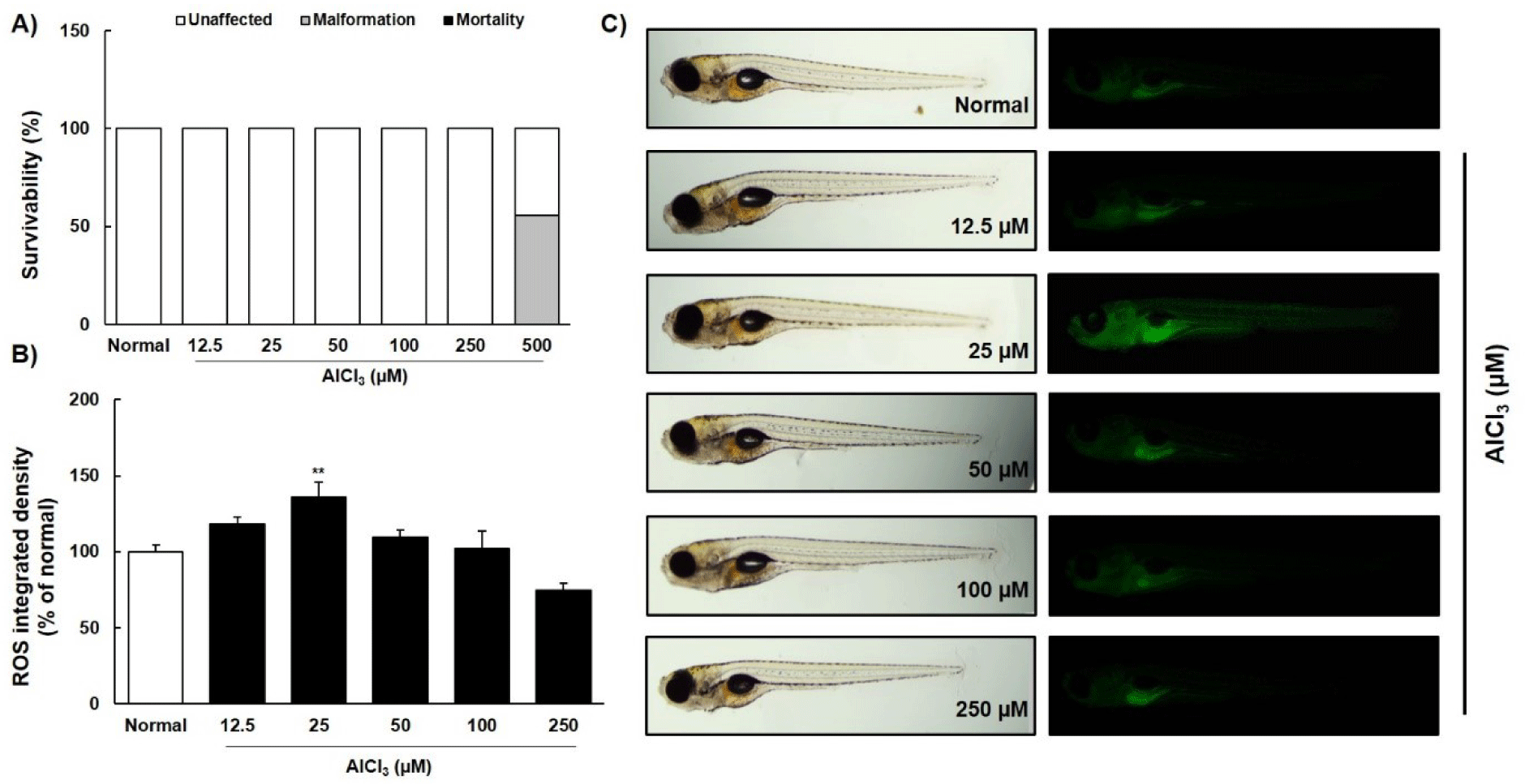
CF90 and CFW extracts treated with AlCl3 (25 μM) did not cause mortality or malformation in zebrafish larvae (Fig. 5A). Intriguingly, the ROS production increased by AlCl3 treatment was significantly suppressed by treatment with the CF90 and CFW extracts (Fig. 5B and 5C).
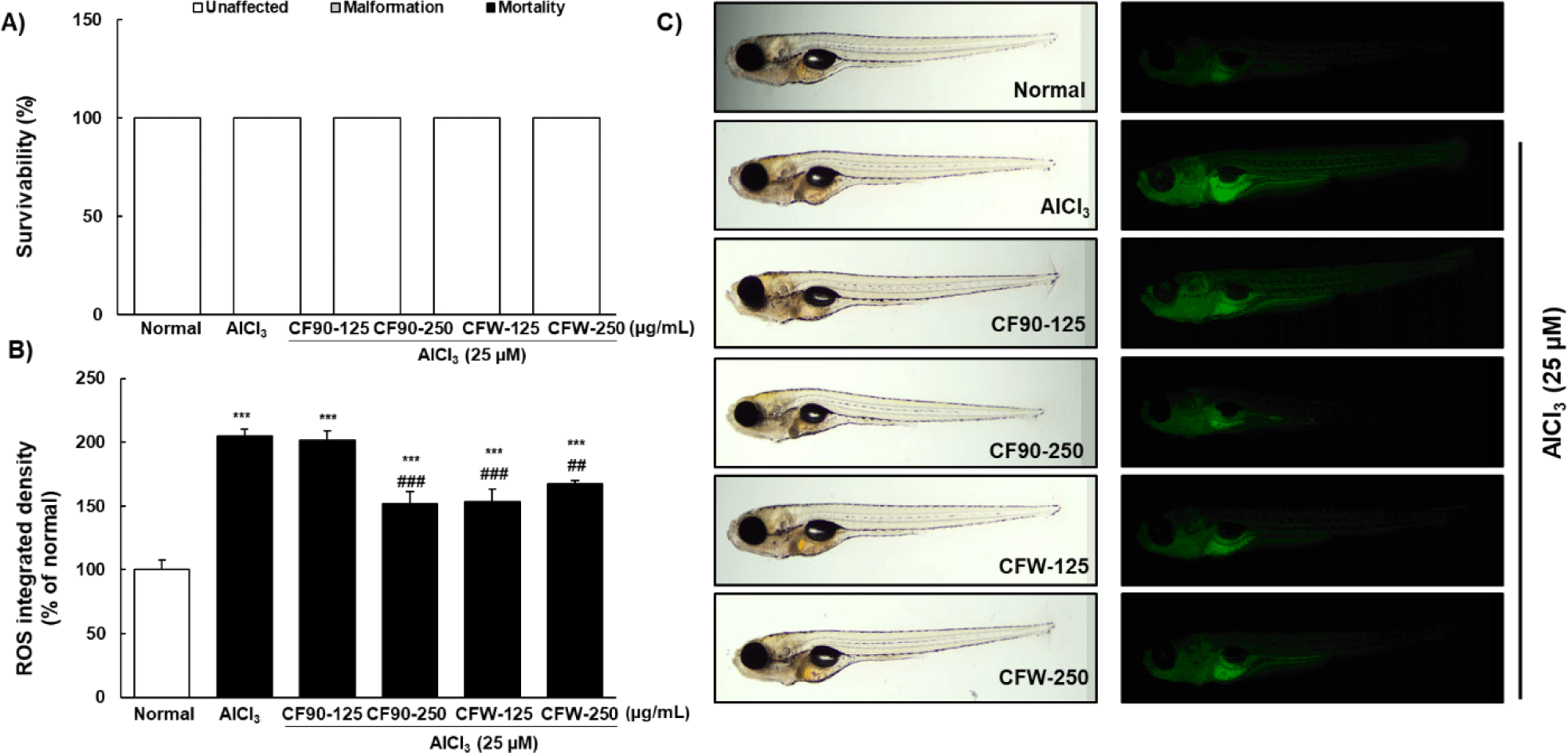
We investigated the mRNA levels of antioxidant enzymes, such as SOD and GPx. Results showed that AlCl3 significantly reduced the mRNA expression of SOD and GPx, and treatment with CF90 and CFW extracts (250 μg/mL) significantly enhanced the activity of both enzymes (Fig. 6). Therefore, our data indicated that CF90 and CFW extracts can restore ROS production and oxidative stress through the regulation of antioxidant enzymes in AlCl3-induced zebrafish larvae.
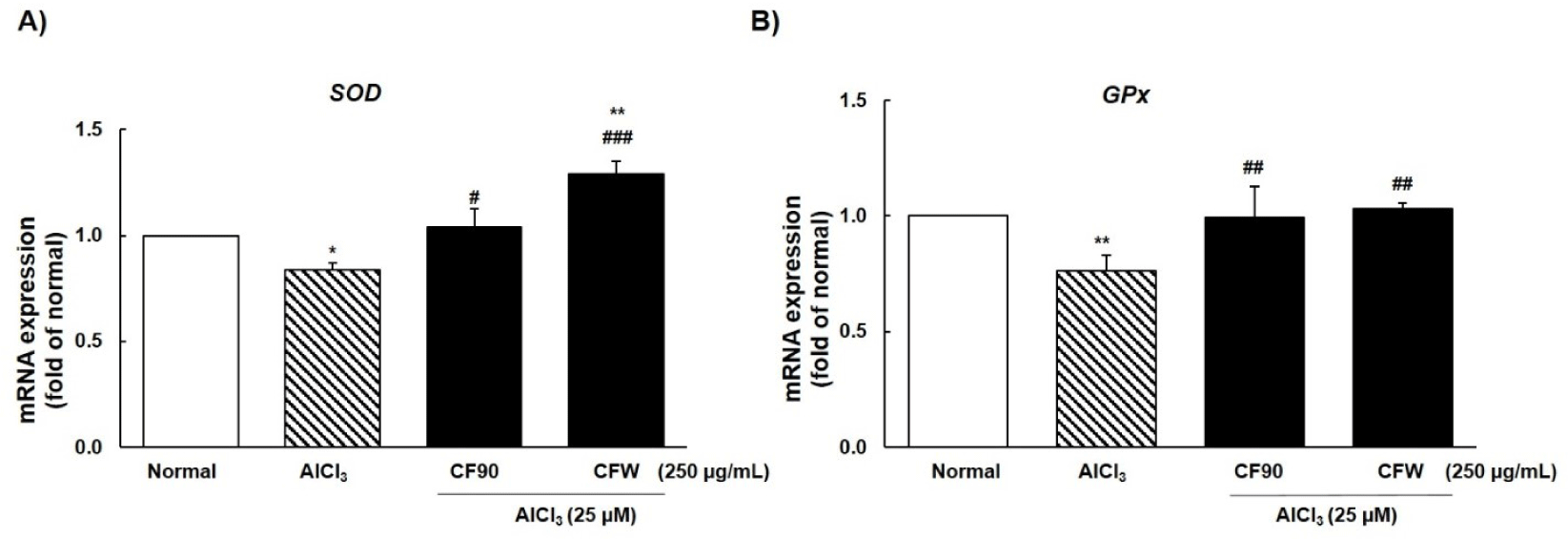
The excess-ROS group showed elevated GSK3β mRNA levels followed by an increase in β-amyloid, tau, and AChE, which cause memory deficits and are the main markers of neurodegeneration. CF90 and CFW extracts showed a significant preventive effect on AlCl3-induced neurodegeneration by downregulating the mRNA expression of GSK3β, β-amyloid, tau, and AChE, hence suggesting their neuroprotective effects (Fig. 7).
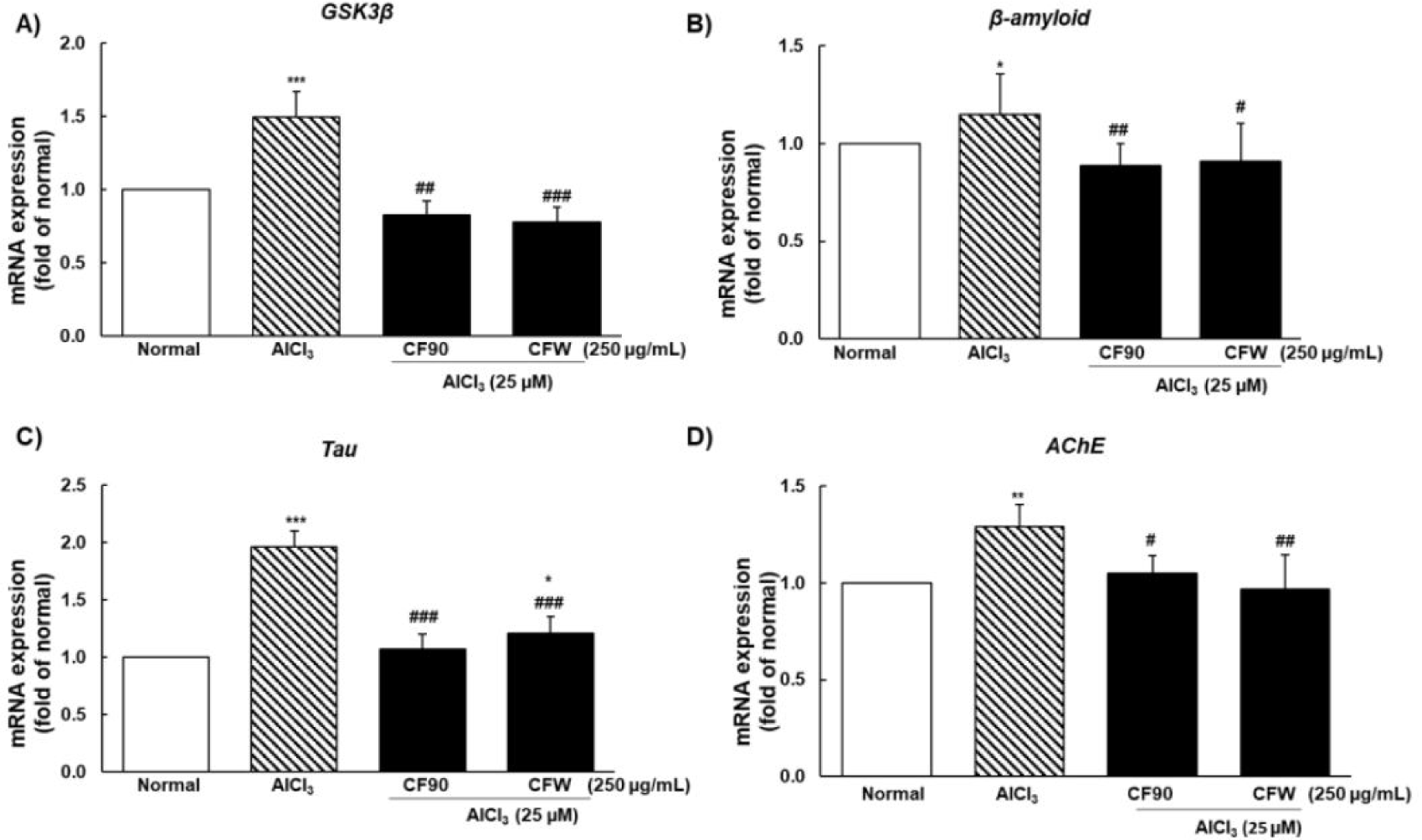
To investigate the neuroprotective effects of CF90 and CFW extracts against AlCl3-induced cognitive dysfunction, we measured the gene expression of BDNF and Trkβ. As shown in Fig. 8, BDNF and Trkβ mRNA expression was significantly decreased due to AlCl3 treatment compared to that in the normal group, and was restored and protected by CF90 and CFW administration.
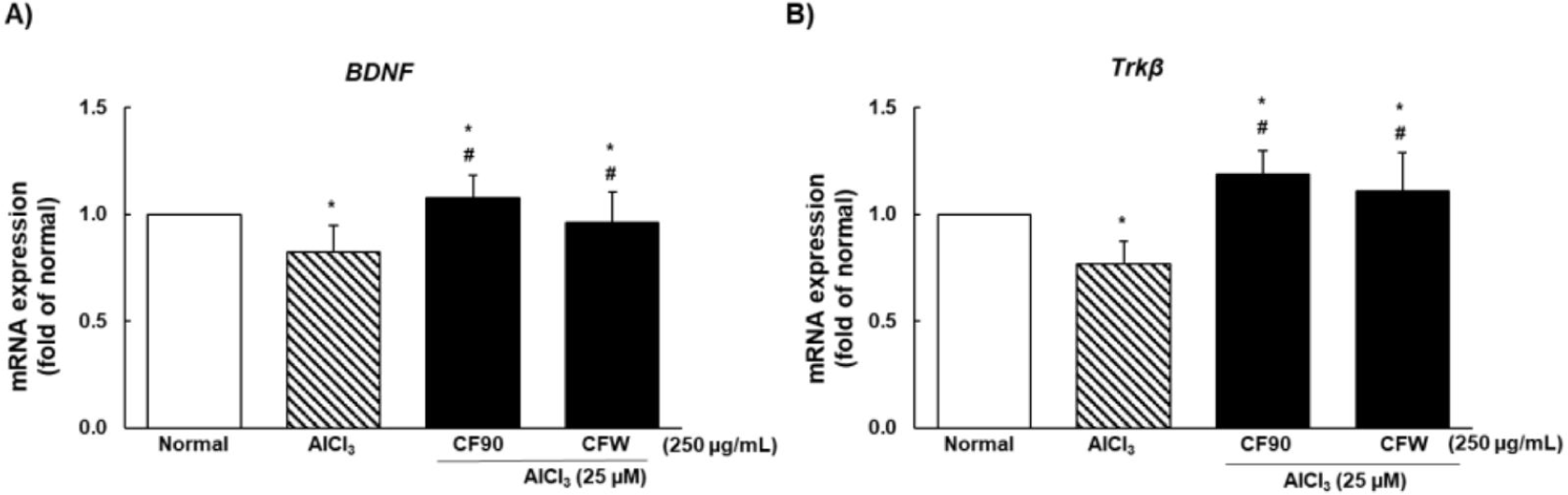
Discussion
The current study investigated the protective effects of CF90 and CFW extracts on oxidative stress-stimulated neurodegenerative dysfunction in PC12 cells and zebrafish larvae. We found H2O2 and AlCl3 to significantly elevate the excessive ROS production in both models, thereby inducing cell death. In addition, the ROS inducers greatly affected the intracellular Ca2+ levels, antioxidant enzyme activity, BDNF/Trkβ signaling, AChE activity, and hallmarks of AD. However, treatment with CF90 and CFW extracts in H2O2-induced PC12 cells or AlCl3-induced zebrafish larvae effectively regulated the excessive ROS production, intracellular Ca2+ levels, and mRNA expression of SOD, GPx, GSK3β, β-amyloid, tau, AChE, BDNF, and Trkβ. Thus, the current study suggested that CF90 and CFW extracts may improve the impairment of cognitive function and synaptic plasticity by regulating ROS generation in neurodegenerative diseases.
Oxidative stress is closely associated with the progression of several neurodegenerative diseases and aging (Singh et al., 2019). Excessive neuronal ROS generation causes lipid peroxidation, DNA repair dysfunction, and loss of the antioxidant defense system (Rodríguez-Fuentes et al., 2015). Several studies have reported that excessive ROS generation in neurons due to external stimuli induces an imbalance in antioxidant homeostasis, stimulates intracellular Ca2+ content, and leads to cell death or apoptosis (Buccellato et al., 2021; Gordeeva et al., 2003; Rico et al., 2007). In addition, neurotoxicity of H2O2 and AlCl3 in various experimental models has been reported to cause oxidative brain damage, trigger apoptosis, and ultimately cause irreversible damage to neurons (Justin-Thenmozhi et al., 2018; Nampoothiri et al., 2015). Therefore, the inducers were considered pathological factors for neuronal development and neurodegenerative diseases, such as AD. In our study, treatment of cultured PC12 neuronal cells with H2O2 showed the induction of cell death in a dose-dependent manner, and significantly amplified intracellular ROS production and Ca2+ content than in normal group. In addition, exposure of zebrafish larvae to AlCl3 significantly altered ROS production as well as mRNA expression of SOD and GPx genes. However, CF90 and CFW extracts restored the imbalance between oxidative stress and Ca2+ content. Our results collectively suggested that CF90 and CFW extracts can ameliorate neuronal damage by modulating oxidative imbalance in H2O2-induced PC12 neuronal cells and AlCl3-induced zebrafish larvae.
Oxidative stress in neurodegenerative process is stimulated by the accumulation of Aβ plaques and hyperphosphorylated tau tangles, leading to signs of cognitive impairment, such as AD (Liu et al., 2015). Therefore, the imbalance between Aβ production and clearance may serve as an important mediator of synaptic loss that promotes NFT and senile infectious disease formation (Kern & Behl, 2009). Hence, oxidative stress is regarded as a critical factor in AD progression. Justin-Thenmozhi et al. (2016) had reported that AlCl3 consumption in rats not only induces Aβ accumulation by increasing oxidative stress, but also increases AChE activity in the hippocampal and cortical regions of the brain, leading to the progression of memory deficits in rats (Justin-Thenmozhi et al., 2016). Cao et al. (2016) had further suggested that AlCl3 can induce neuroinflammation, resulting in learning and memory impairments in rats. BDNF and Trkβ play critical roles as major neurotrophic factors in synaptic plasticity and cognitive function (Cao et al., 2016). Our study showed that excessive ROS generation due to exposure to AlCl3 in zebrafish larvae significantly increased or decreased the mRNA expression of Aβ, tau, GSK3β, AChE, BDNF, and Trkβ genes. However, administration of CF90 and CFW effectively controlled the expression of mRNA genes associated with AD or cognitive function through restoration of normal antioxidant enzyme activity.
In summary, our results revealed that H2O2 or AlCl3 could enhance excessive ROS generation in PC12 neuronal cells and zebrafish, resulting in severe cognitive impairment and loss of synaptic plasticity. In contrast, CF90 and CFW extracts effectively improved neurodegeneration by normalizing antioxidant enzyme activity, inhibiting AChE activity, downregulating Aβ, GSK3β, and tau gene expression, and upregulating BDNF/Trkβ signals.