Introduction
Reactive oxygen species (ROS) are chemically reactive materials that can interact with cellular biomolecules, among which are oxygen-containing free radicals, such as peroxyl (ROO·), hydroperoxyl (HO2·), alkoxyl (RO·), sulfonyl (ROS·), hydroxyl (OH·), superoxide (O2·), thiyl peroxyl (RSOO·), and nitric oxide (NO·), and oxidizing agents, including hypochlorous acid (HOCl), singlet oxide (1O2), hydrogen peroxide (H2O2), and organic hydroperoxides (ROOH) (Fernando et al., 2016). However, the excessive production of ROS and free radicals cause an imbalance in the oxidant/antioxidant system, resulting in oxidative stress that is, in turn, highly associated with various diseases such as chronic kidney, cardiovascular, and neurodegenerative diseases (Chen et al., 2020; Gyurászová et al., 2020; Lee et al., 2014; Podkowińska & Formanowicz, 2020; Rekatsina et al., 2020).
Inflammation is a general immunologic defense response that activates specific physiological and pathological conditions in the human body. Normally, it plays crucial role in non-specific immunity, including detection of bacteria, viruses, and chemical stimuli. However, excessive and chronic inflammation can lead to detrimental inflammatory diseases such as diabetes, arthritis, cancer, and cardiovascular and bowel diseases (Libby, 2007). According to previous reports, excessive production of inflammatory mediators and cytokines is detrimental to exacerbated inflammation. Therefore, the reduction of inflammatory mediators and cytokines may be an effective strategy to treat inflammatory diseases.
Ecklonia maxima (E. maxima) is a brown alga belonging to the genus Ecklonia that is mainly found on the southern coast of Cape Town, South Africa (Rothman et al., 2017), representing a major kelp bed that forms seaweed in this region. Bolton et al. reported the distribution and seasonal habitat changes of E. maxima in South Africa for over 70 years (Bolton et al., 2012). However, this has not been fully explored, except in an ecological study (Anderson et al., 1997; Bolton & Levitt, 1985; Ciepiela et al., 2016; Featonby-Smith & Van Staden, 1984; Feng & Aldrich, 2004; Kocira et al., 2018; Mayombo et al., 2019; Papenfus et al., 2012; Papenfuss, 1942; Rothman et al., 2006; Rouphael et al., 2017), and biochemical basic analysis of its antioxidant, neuroprotective, and antidiabetic activities (Kannan et al., 2013; Kulkarni et al., 2019; Mwangi et al., 2013; Rengasamy et al., 2013; Wang et al., 2018; Zhou et al., 2019). In recent decades, large-scale biochemical studies were performed to identify the bioactive compounds of E. maxima (Olasehinde et al., 2019; Zhou et al., 2019). In our previous study, a sulfated polysaccharide from E. maxima showed antioxidant, anti-melanogenesis, and ultraviolet protective activities (Wang et al., 2020). However, most of the previous studies focused on the evaluation of the bioactivity of E. maxima blades or its whole body, and the potential bioactivities of the two main structures of E. maxima (blades and stipe) have not been clarified. Accordingly, the comparative studies of two structures of E. maxima blades and stipe have been urged for industrial application.
Therefore, in the present study, enzyme assisted hydrolysate from the two structure of E. maxima blades and stipe were prepared by enzyme-assisted hydrolyzation and screened for their potential antioxidant and anti-inflammatory activities. We aimed to establish a comparative evaluation of two structure of E. maxima blades and stipe for antioxidant and anti-inflammatory activities, which may help increase their use as functional ingredients in the food and functional food industries.
Materials and Methods
Dulbecco’s Modified Eagle’s Medium (DMEM), Roswell Park Memorial Institute medium (RPMI) 1640, Fetal vobine serum (FBS), penicillin-streptomycin, and trypsin were purchased from gibco (Mississauga, Ontario, Canada). Bicinchoninic acid (BCA) protein assay kit (Thermo Scientific, Pittsburgh, PA, USA), bovine serum albumin (Bovogen, VIC, Australia). Barium chloride dihydrate, ethanol, iron (II) sulfate heptahydrate, acetic acid, hydrochloric acid, nitric acid, ammonium sulfate, sodium hydroxide, phenol, and sulfuric acid were purchased from Daejung (Seoul, Korea). Gallic acid, glucose, Folin & Ciocalteu’s phenol reagent, peroxidase, 2’,7’-dichlorofluorescein-diacetate (DCF-DA), 3-(4,5-dimethylthiazol-2-yl)-2,5-diphenyltetrazolium bromide (MTT), gum arabic, and lipopolysaccharide (LPS) were purchased from Sigma-Aldrich (St, Louis, MO, USA). Hydrogen peroxide (Junsei, Japan), dimethyl sulfoxide (VWR International, West Chester, PA) were purchased for experiments. Commercial food grade enzymes (Viscozyme® L; Vis, Celluclast® 1.5 L; Cellu, AMG 300L; AMG, Termammyl 120L; Ter, Ultraflo Max; Ult) were purchased from Novozyme (Bagsvaerd, Copenhagen, Denmark).
The E. maxima (E. maxima) was collected and provided from the University of Cape Town, South Africa. The 10 g of E. maxima blades and stipe were hydrolyzed with carbohydrases (Vis [pH 4.50, 50°C], Cellu [pH 4.50, 50°C], AMG [pH 4.50, 60°C], Ter [pH 6.00, 60°C], Ult [pH 7.00, 60°C]) for 24 h. After 24 h, the mixtures were clarified by centrifugation (12,298×g, 15 min, 4°C) to remove residue. Then the supernatants were filtered with Whatman filter paper under vacuum. The filtrates were inactivated (100°C, 10 min) and the pH was neutralized at pH 7.00 using 1 M NaOH solution. Then the enzyme-assistant extracts were stored at –80°C freezer and lyophilized for further experiments.
The chemical compositions were analyzed on the enzyme assisted hydrolysate from the two structure of E. maxima, blades and stipe followed the Association of Official Analytic Chemists (AOAC) method (AOAC, 1999). Total polysaccharide contents were determined with the phenol-sulfuric acid analysis which is most applicable to measure the total glucose levels. Glucose was used as a standard (Dubois et al., 1956). The protein contents were measured by Lowry analysis. Bovine serum albumin was used as standard (Waterborg, 2009). The polyphenolic contents were analyzed by the Folin-Ciocalteu method. Gallic acid was used to measure polyphenol contents (Chandler & Dodds, 1983). The sulfate contents were measured with BaSO4 assay (Dodgson & Price, 1962).
The monosugar analysis was performed with modified methods from Kang et al. (2019). The monosugar contents were analyzed by liquid chromatography (HPAEC-PAD system, Dionex, Sunnyvale, CA, USA) equipped with CarboPac PA1 column (4.5 mm × 50 mm). The analytic samples were hydrolyzed with 4 M of trifluoracetic acid (TFA) and subjected to CarboPac PA1 column. The relative monosugar contents were calculated with standard mixtures containing (Fucose, Rhamnose, Arabinose, Galactose, Glucose, Mannose, Fructose).
The potential free radical scavenging activity was determined with the hydrogen peroxide scavenging method (Kim et al., 2014). Briefly, 100 µL of the sample solution and 1 M phosphate buffer (PBS) were mixed in a 96-well plate. Then, 20 µL of hydrogen peroxide (10 mM) were added to all of the wells and incubated for 5 min. After 5 min of incubation, 30 µL of ABTS (1.25 mM) and peroxidase (1 unit/mL) were added and additionally incubated for 10 min at 37°C. The absorbance was measured using the microplate reader (Synergy HT Multi-Detection microplate reader, Bio-Tek, Winooski, VT, USA) at 405 nm.
Vero cells and Raw 264.7 cells were purchased from the Korean Cell Line Bank (KCLB, Seoul, Korea). The Vero cells were cultured in RPMI medium containing 10% FBS and 1% penicillin and streptomycin (P/S). The Raw 264.7 cells were cultured in DMEM medium supplemented with 10% FBS and 1% of P/S. Cells were maintained in the incubator (37°C, 5% CO2) and periodically sub-cultured every 2 days interval.
MTT assay was adopted to determine the cytotoxic effect of the tested samples (Lee et al., 2021). Briefly, the cells were seeded in 96 well plates (1 × 105 cells/well) and incubated for 24 h. When the cell confluence was reached 70%, the different concentrations of samples were treated before stimulant induction and incubated for 24 h. After 24 h of incubation, the MTT stock solution (2 mg/mL) was added to each well and incubated more an additional 3 h. Following 3 h, the supernatant was discarded, and 200 µL of DMSO was added to calculate the amount of formazan contents. The absorbance was measured using microplate reader at 540 nm.
The intracellular ROS levels were measured by 2’,7’-dichlorofluorescein diacetate (DCF-DA) fluorescent assay as following previous methods described by Kang et al. (2019). Briefly, Vero cells were seeded in a 96 well plate and incubated for 24 h. After 24 h incubation, the tested samples were treated before 1 mM of H2O2 treatment and incubated for 24 h. 24 h later the DCF-DA (500 μg/mL) was added to each wells. The fluorescent measurement was detected by microplate reader (Synergy HT Multi-Detection microplatereader, Bio-Tek) at an excitation and emission wavelength of 485 mm/535 nm.
The nitric oxide (NO) production was measured in LPS-stimulated Raw 264.7 cells by Griess assay (Wang et al., 2020). Briefly, Raw 264.7 cells were seeded in a 96 well plate and incubated for 24 h. after 24 h of incubation, the tested samples were treated before 1 μg/mL of lipopolysaccharide (LPS) treatment and incubated more additional 24 h. After 24 h of incubation, the 100 µL of Griess solution was added and the measurement were detected by microplate-reader (Synergy HT Multi-Detection microplate reader, Bio-Tek) at 500 nm.
A total 1×105 cells/wells were incubated and treated with different concentrations (31.25-250 µg/mL) of tested samples before LPS (1 µg/mL) treatment and subsequently incubated for 24 h. After 24 h of incubation, the cell culture medium was collected to analyze the pro-inflammatory cytokine production. The pro-inflammatory cytokines (IL-6 and TNF-α) were analyzed with commercial cytokines analysis ELISA kits (R&D System, Minneapolis, MN, USA).
All data are expressed as the mean ± SE and the statistical analysis was performed with GraphPad prism (Version5.01; GraphPad Software, San Diego, CA, USA). The significant differences were expressed as follows: *p < 0.05, **p < 0.01, ***p < 0.001, ****p < 0.0001 compared with negative control group, #p < 0.05, ##p < 0.01, ###p < 0.001, ####p < 0.0001 compared with control group.
Results
Measurement of extraction yield, chemical composition and hydrogen peroxide scavenging activity of enzyme assisted hydrolysate from E. maxima blades and stipe.
The extraction yield and chemical composition results give an important nutritional knowledge on enzyme-assisted hydrolysate from E. maxima. The extraction yield, chemical composition, and hydrogen peroxide scavenging activity are summarized in Table 1. Accordingly, the extraction yields were significantly increased in enzyme assisted hydrolysate compared with the water extracts and the extraction yields of the blades were higher than stipe. In addition, high polysaccharide and relatively low polyphenol contents were obserbed in all of the enzyme assisted hydrolysate from E. maxima. Among the enzyme assisted hydrolysates, celluclast assisted hydrolysate from E. maxima blade (EMBC) and viscozyme assisted hydrolysate from E. maxima stipe (EMSV) showed the highest polysaccharide contents (EMBC, 49.64 ± 0.34%) and (EMSV, 20.17 ± 0.21%) respectively. The potential antioxidant activities of EMBC and EMSV were determined using a hydrogen peroxide scavenging assay. According to the results, the relatively strong hydrogen peroxide scavenging activity was observed in EMBC and EMSV. Therefore, they were chosen for further experiments. The IC50 values were 60.68 ± 0.01 µg/mL and 79.81 ± 0.18 µg/mL for EMBC and EMSV, respectively.
As indicated in Fig. 1, monosugar compositions of EMBC and EMSV indicated that the EMBC were generally composed of glucose (72.10%), fucose (27.68%), arabinose (0.22%), and EMSV composited of six monosugar such as fucose (47.19%), mannose (22.69%), galactose (15.46%), glucose (12.91%), rhamnose (1.64%), and arabinose (0.12%). Especially the high glucose contents were detected in EMBC, while the EMSV contained high fucose contents.
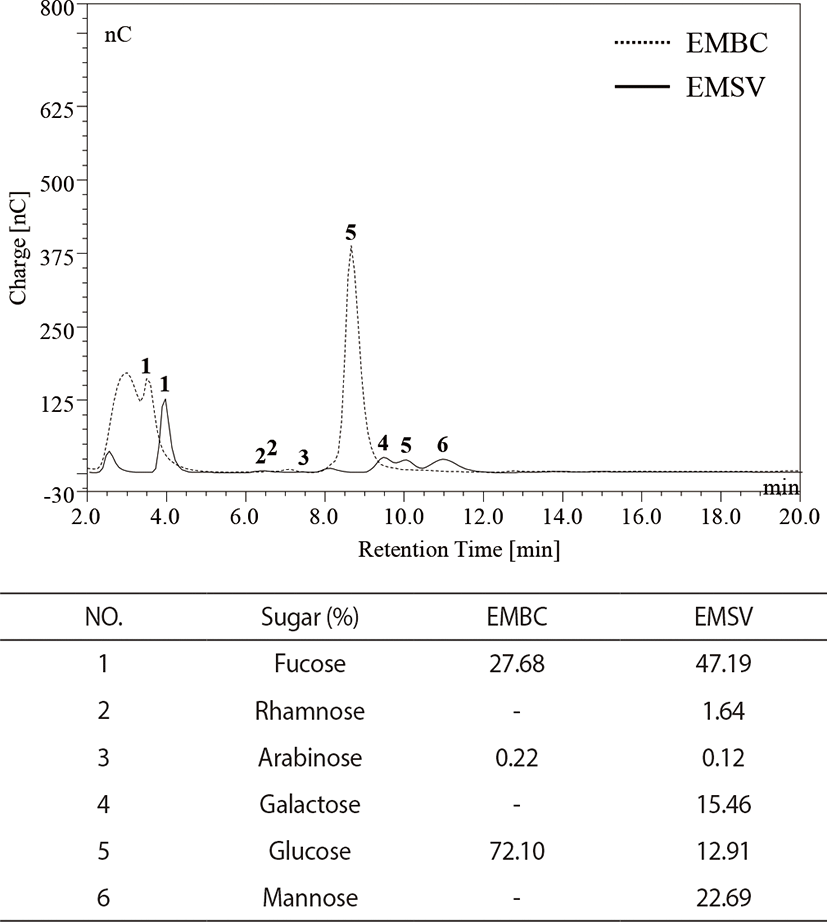
To evaluate the potent antioxidant activities of EMBC and EMSV, the cytotoxicity, H2O2-induced cell viability and intracellular ROS production were measured in Vero cells (Fig. 2). Fig. 2A demonstrates that the concentration ranges of EMBC and EMSV (125, 250 mg/mL) did not show cytotoxic effects on Vero cells. Thus, the given concentrations (125 and 250 µg/mL) were chosen for further studies. To evaluate the protective effect of EMBC and EMSV against H2O2 exposed oxidative stress we performed an MTT and DCF-DA assay to measure the cell viability and intracellular ROS production. As shown in Figs 2B and 2C, the cell viability significantly diminished to 70.10 ± 1.78% by H2O2-exposure. However, EMBC and EMSV remarkably decreased H2O2-induced cell death. Also, EMBC and EMSV remarkably decreased intracellular ROS production in H2O2-exposed Vero cells compared to H2O2-treated group. However, the 125 mg/mL of EMSV was not effective on H2O2-induced cell viability and ROS production. These results indicated that EMBC and EMSV possessed significant protection on H2O2-induced oxidative damages and the potential ROS scavenging activity was higher in EMBC than EMSV.
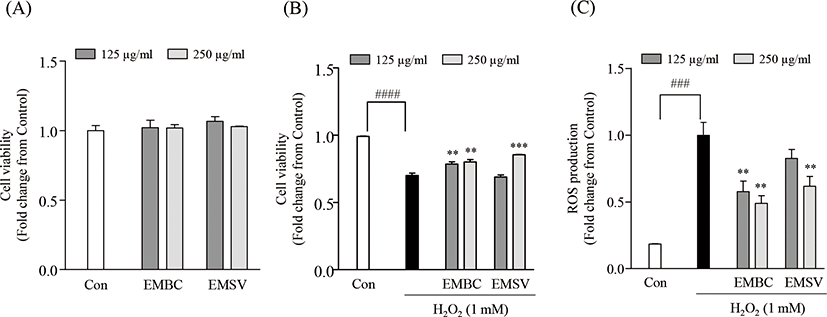
To determine whether the anti-inflammatory effect of EMBC and EMSV, the cytotoxicity, lipopolysaccharide (LPS)-induced cell viability and nitric oxide (NO) production were measured in Raw 264.7 cells. In Fig. 3A showed that the EMBC and EMSV did not express cytotoxicity on Raw 264.7 cells. As shown in Fig. 3B and 3C the cell viability and NO production were significantly up-regulated. While the EMBC and EMSV treatment significantly reduced NO production and increased cell viability in LPS-stimulated Raw 264.7 cells. Accordingly, the results demonstrated that the EMBC and EMSV showed anti-inflammatory activity by suppression of LPS-stimulated NO productions in Raw 264.7 cells.
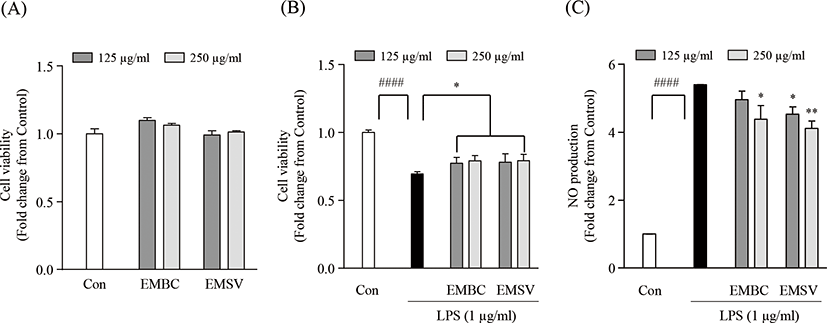
To elucidate the mechanisms of the anti-inflammatory effect of EMBC and EMSV the pro-inflammatory cytokine productions were analyzed in LPS-stimulated Raw 264.7 cells. As shown in Fig. 4, the LPS stimulation significantly increased inflammatory IL-6 and TNF-α productions. However, the LPS-activated IL-6 and TNF-α were significantly lowered with EMBC and EMSV treatment. EMBC showed strong IL-6 inhibitory activity compared to EMSV. These results indicated that EMBC and EMSV showed anti-inflammatory properties by downregulation of the pro-inflammatory cytokine productions in LPS-treated RAW 264.7 cells.
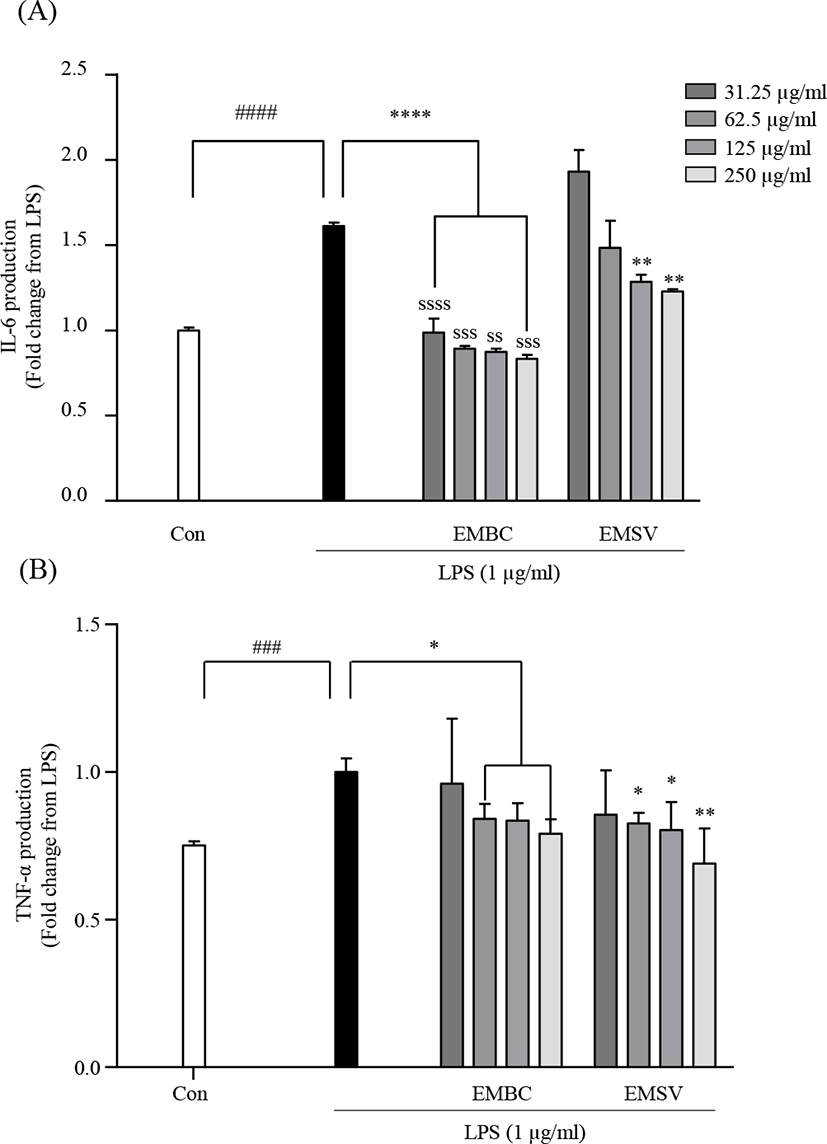
Discussion
Marine brown seaweeds have been recognized as a traditional food widely consumed in Asian countries, including Korea, Japan, and China (Pereira et al., 2020). Recently, marine natural products have received great attention in the functional food, nutraceutical, and cosmeceutical fields due to their antioxidant and anti-inflammatory properties (Nguyen et al., 2020). There is substantial evidence that marine-derived natural products have a broad range of biological activities (Carroll et al., 2020). Indeed, marine organisms are renowned for their biological and nutritional richness, comprising polysaccharides, polyphenols, fatty acids, lipids, peptides, essential vitamins, and minerals, which exhibit valuable proprieties including antidiabetic, antihypertensive, anti-inflammatory, antioxidant, and antiviral activities (Fernando et al., 2017; Peñalver et al., 2020). Hence, these functional ingredients from seaweeds have potential health benefits for human cardiovascular and chronic diseases (Cardoso et al., 2015; Lordan et al., 2011). Further, they have been used in drug discovery for the development of alternative strategies to synthetic medicine, which is often accompanied by undesirable adverse effects. Several different types of marine-derived bioactive compounds have been industrially discovered and commercialized (Pereira et al., 2019), with pre-clinical and clinical trials being performed on numerous marine-derived bioactive compounds (Newman & Cragg, 2004).
The present study investigated the potential antioxidant and anti-inflammatory properties of enzyme-assisted hydrolysate from EMBC and stipe (EMSV) to determine their relative antioxidant and anti-inflammatory activities. Enzymatic hydrolysis was carried out using different carbohydrases, including Viscozyme, Celluclast, AMG, Termammyl, and Ultraflo. According to the results, the enzymatic extraction yields were higher in the blades than in the stipe. Further, the total polysaccharide content was slightly higher in the blades than in the stipe. Monosaccharide analysis revealed that EMBC and EMSV consist of fucose, arabinose, and glucose contents. Especially the higher fucose content was observed in EMSV and highest glucose content was detected in EMBC respectively. In previous publications indicated that the fucose rich hydrolysates highly attribute to their bioactivities especially antixodant and anti-inflammatory activities and Castro et al. (2014) also reported the potential antioxidant and anti-inflammatory activities of fucose containing sulfated polysaccharide from Lobophora variegata (Castro et al., 2014).
Each of the five enzyme-assisted hydrolysate obtained from E. maxima blades and stipe had the strong hydrogen peroxide scavenging activity. Among them, EMBC and EMSV showed excellent hydrogen peroxide scavenging activity. In addition, it has significant protection against H2O2 induced oxidative damage in Vero cells. However, there is no significant difference in antioxidant activities between EMBC and EMSV. Moreover, the EMBC and EMSV significantly downregulated the NO production in LPS-stimulated Raw 264.7 cells by downregulation of pro-inflammatory cytokine (IL-6 and TNF-α) production. In particular, EMBC considerably inhibited the IL-6 production compared to EMSV. The pro-inflammatory cytokines such as IL-6 and TNF-a involve the initiation and inflammatory responses. Contrary to the LPS is a stimulator of immune responses which induce macrophage activation and cytokine production. Therefore the modulation of IL-6 and TNF-α production may be an effective strategy to ameliorate the excessive immune responses against LPS exposure in Raw 264.7 cells. Taken together, these results indicated that the enzyme assisted hydrolysate from the two structures of E. maxima blades and stipe are potential candidate for the development of antioxidant and anti-inflammatory materials and could be used as a functional ingredient in functional food industry.