Introduction
Decapods often comprise species of economic importance. The blue swimming crab, Portunus pelagicus (Decapoda, Brachyura, Portunidae) widely distributed within the Indo-Pacific (Lai et al., 2010), is present in large numbers and are valuable for both recreational and commercial fisheries in Vietnam. As other portunid benthic crabs, their exoskeletons are commonly colonized by an assemblage of symbiotic organisms. These symbionts can be parasitic, epibionts (Shields & Overstreet, 2003), and fouling taxa such as ciliate protozoa, barnacles, red algae, tube and round worms, and hydrozoa (Isaeva et al., 2005).
These symbiotic communities have different degrees of effect on the host (Fernandez-Leborans, 2010). Mutualistic or commensal relationships can assist the host in providing both mimetic protection and cleaning (Fernandez-Leborans, 2010). Fouling organisms such as stalked (mostly Octolasmis spp.) and acorn (Chelonibia patula/testudinaria) barnacles are often reported as abundant in commercial crab species (Faisal et al., 2010; Gaddes & Sumpton, 2004; Jeffries et al., 1982; Shields & Overstreet, 2003). These species typically cause minor effects but may be harmful to respiration, mobility and normal behavior if they occur in high numbers (Jeffries et al., 2005; Waiho et al., 2017). Much attention has been also paid to the egg-reducing parasitic symbionts, which can have severe effects on the fitness of the host, due to decreasing fecundity and subsequent effects on the crab population (Glenner et al., 2008; Kahar et al., 2016; Mouritsen & Jensen, 2006; Waiho et al., 2017). Examples of these include Sacculina granifera (Phillips & Cannon, 1978), the nicothoid copepod Choniosphaera indica (Shields & Wood, 1993), and the nemertean Carcinonemertes mitsukurii (Shields & Wood, 1993; Vo et al., 2013).
A high diversity of symbiotic species have been reported occupying different body parts of P. pelagicus in Australia (Gaddes & Sumpton, 2004; Shields, 1992; Shields & Wood, 1993), the Indian Ocean (Faisal et al., 2010; Khattab, 2017), and other Asian countries (Heirina et al., 2021; Jeffries et al., 1982, 2005; Le et al., 2018a, 2018b; Supplementary Table S1). Shields (1992) reported 15 species of endo- and ectosymbiontic parasites on P. pelagicus in Australia. Jeffries et al. (2005) detected 6 Octolasmis species inhabiting 5 crab hosts (including P. pelagicus) in the Gulf of Thailand, Faisal et al. (2010) found 6 external and internal parasites in P. pelagicus distributed in the Arabian Gulf, and Vo et al. (2013) reported 4 species occurring on P. pelagicus in Vietnam.
Symbioses are important ecological relationships in aquatic ecosystems that still require extensive research on a wide variety of organisms and spatial scales (Fernandez-Leborans, 2010; Santoro et al., 2020). Spatial variation in symbiont diversity has been detected at both large scales such as the Indo-Pacific and Atlantic Oceans (Morand & Krasnov, 2010) and small scales on the order of kilometers (Casariego et al., 2015; Sloan et al., 2010). These spatial variations are also accompanied by variations in feeding behavior, body size and sexes of the host (Gallegos-Navarro et al., 2018; Sloan et al., 2010), as well as parasitic exposure and susceptibility (Gibson et al., 2016). The symbiotic load may have consequent impacts on P. pelagicus host populations such as on growth (Le et al., 2018a; Vo et al., 2013), moulting ability (Gaddes & Sumpton, 2004), reproduction (Khattab, 2017), and behavior (Phillips & Cannon, 1978).
This study aims to (i) provide an inventory and infestation status of ectosymbiotic associations on P. pelagicus from Vietnam, (ii) examine the corelation between host distribution, sex, and size with the diversity of the symbiotic assemblage, and (iii) investigate the effect of symbiont abundance on host crab features such as body weight and distribution.
Materials and Methods
Swimming crabs were collected from coastal Vietnam from Cat Ba Island-Hai Phong, Ha Long Bay-Quang Ninh in the North (north population); Nha Trang and Van Phong Bay-Khanh Hoa, Song Cau and Tuy Hoa-Phu Yen in the Central region (central population), and Phu Quoc Island and Rach Gia-Kien Giang in the South (south population; Fig. 1A). The crabs were transported on ice for fresh dissection in a laboratory.
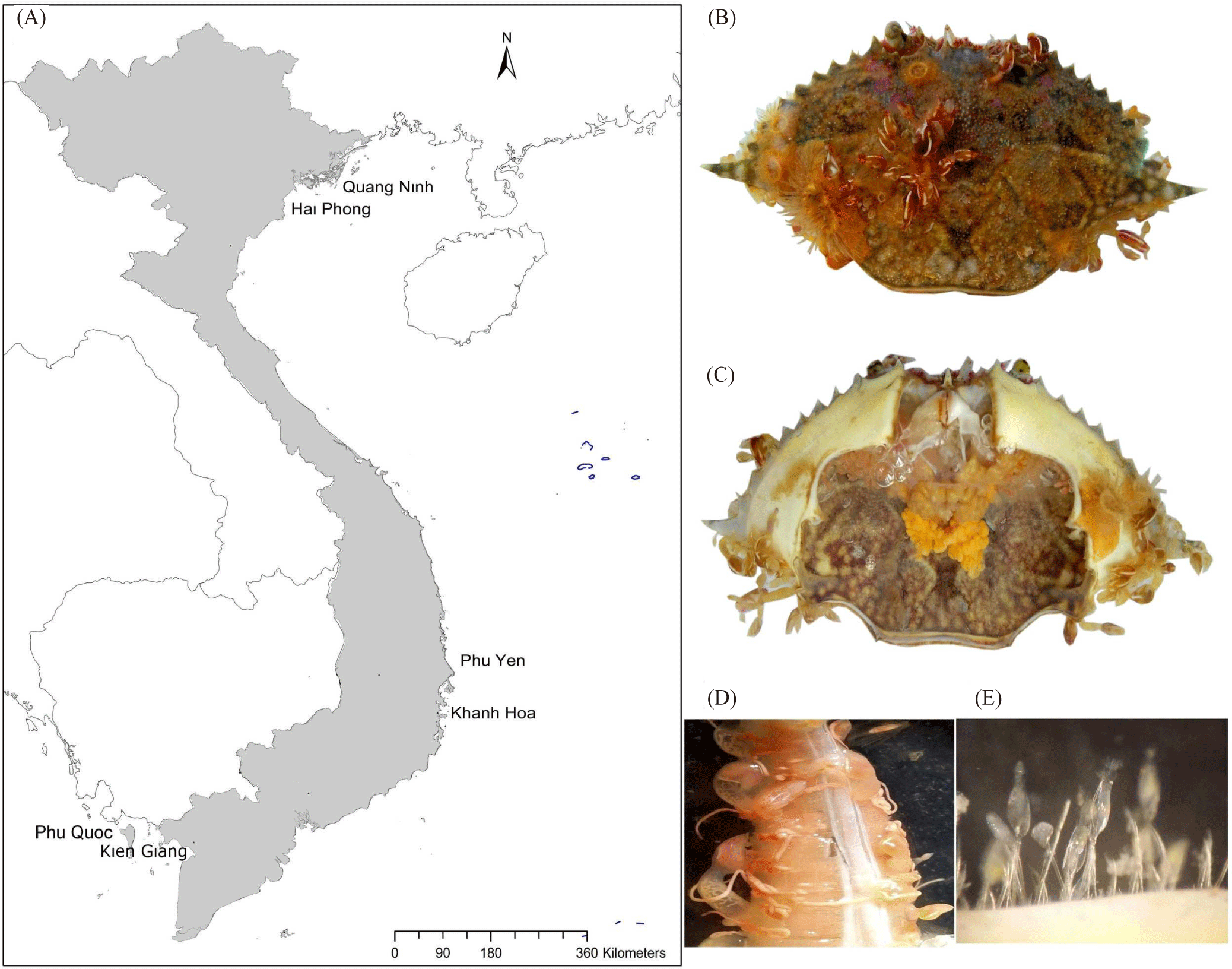
For each crab sex, and size (carapace width [CW] and body weight [BW]) were recorded. Carapace width of 80 mm roughly corresponds to the size-at-maturity for P. pelagicus in Vietnam (Ha et al., 2014). To examine size differences in the infestation pattern, the crabs were subdivided into four groups: CW ≤ 80, 80–100, 100–120, and > 120 mm.
Crabs were examined externally for obvious ectosymbionts. The carapace and abdomen of each crab was lifted off the body for dissection. Crabs were dissected into 5 examination sites: (1) carapace, (2) limbs (maxilliped, chelliped, and swimming legs), (3) abdomen (including egg clutches, if present), (4) mouth parts, and (5) gills (Supplementary Fig. S1). Each part was separated into petri dishes containing clean seawater for gross inspection or by microscopy (Olympus SZX9, Olympus, Hamburg, Germany).
Symbiotic species intended as whole-mounts were transferred to a vial of 70% alcohol (except Protozoa prepared only in the wet mounts) and those for DNA analysis were preserved in 95% EtOH and/or RNAlater (in case of protozoa) and stored at –20°C. Symbiotic species were identified using various keys and species descriptions following Jeffries et al. (2005), Lynn (2007), and Shields & Overstreet (2003). Type and voucher materials were deposited at the National Oceanographic Museum of Vietnam, Nha Trang, Vietnam.
DNA was extracted from individual or protozoa polyp of each collected symbiont species using DNAeasy Tissue Extraction Kit (Qiagen, Hilden, Germany) in accordance with the manufacturer’s instructions. Ribosomal nucleus (28S rRNA) and mitochondrial DNA (cytochrome c oxidase subunit I–COI mtDNA) markers were amplified using the primers LSU-5 and 1500R (Olson et al., 2003), and LCO1490 and HCO2198 (Folmer et al., 1994), respectively. With the 18S rRNA gene, we designed the primers: 18uniF: 5’- CGGAGAATTAGGGTTCGATTCC3’ and 18uniR 5’CTAAGGGCATCACAGACCTG3’ for Protozoan, Anthozoan and Bivalve species.
The basic logical alignment search tool (BLAST, http://www.ncbi.nlm.nih.gov/BLAST) was used to identify the resulting sequences to confirm species identification. For gastropod, bryozoan and ciliate species, PCR reactions failed because of degenerated DNA. Therefore, only morphological identification was considered for those species. Due to the limitation of compatible sequences of symbionts available in biological databases, some of the current sequences were compared with the sequences of species from the same and/or different genera in the same family. Information on gene application, and Genbank accession numbers are presented in Supplementary Table S2.
Data was analyzed according to sampling locations representative for the crab distribution (6 sites) and geographically defined populations (north, central, and south), sexes (male and female) and sizes (4 groups). Infestation pattern including prevalence (P) and mean intensity (MI) were calculated following Margolis et al. (1982) and Rózsa et al. (2000). Data were tested for normality and those of non-normal distribution were log-transformed to reduce differences in variances between groups.
We tested the difference between geographical crab populations, crab sexes, and carapace size groups against prevalence and mean intensity of each symbiont species using one-way ANOVA performed with Tukey’s post hoc tests. Further tests were conducted to investigate the difference in mean intensity between crab populations, sexes, and size groups (two-way ANOVA, Tukey’s post hoc tests), and crab populations, sexes and size groups (three-way ANOVA, Tukey’s post hoc tests). All statistical analyses were performed using SPSS 24.0 (SPSS, Chicago, IL, USA).
We also examined the relationship of symbiotic assemblage diversity based on mean abundance (Margolis et al., 1982) with host features (body weight and distribution). Symbiont species were ranked (Supplementary Fig. S2) based on relative abundance using “goeveg” package performed in R studio. There were 12 species considered common occurring (2), in Table 1) and were representative for symbiotic assemblage of which 4 species were assigned as dominant (relative abundant > 0.1 in all sampling sites [1), Table 1]). For each physical area (6 sites) and population (north, central and south), we calculated five diversity indices from the symbiotic assemblage: species richness (S), evenness (E), Shannon index (H), Simpsons dominance index (D), and Berger-parker dominance index (d) using the Biodiversity Pro 2.0 software (McAleece et al., 1997).
a–d; x-z Different letters mean prevalence and mean intensity was significantly different from geographical locations (north, central, and south) and sexes (male and female), and crab size groups (one-way ANOVA; p < 0.05). Infection sites were marked as (1) carapace, (2) limb, (3) abdomen, (4) mouth part, and (5) gill.
Results
In total, 18 taxa (Table 1) were associated with P. pelagicus in coastal Vietnam. Among them, we identified Triticella flava (bryozoan) based on morphological characters. Two externally morphologically similar individuals of a gastropod and a ciliate (Epistylis sp., Fig. 1E) yielded unsuccessful PCR reactions, and diagnostic morphological characters were not discernable. There were three unidentified species belonging to three genera (Hydroides, Notoplana, and Semibalanus), which were not previously recorded on P. pelagicus showed sequence identity of greater than 90% with available sequences of the same genus. Additionally, two unidentified species (Loxosomella sp. and Zoothamnium sp.), which had been previously reported on P. pelagicus, displayed low (82.8%, COI mtDNA) to moderate (≥96%, 18S rRNA) sequence identity (Supplementary Table S3). We also found an acorn barnacle, C. testudinaria, which is morphologically similar, but recently distinguished from C. patula (Cheang et al., 2013). Our combined 16S rRNA+COI mtDNA sequences supported two distinct species (4.4% and 5.6%, respectively) (Dang et al., 2022). The sequences of well-known C. mitsukurii also showed low identity (85%, COI mtDNA, and approx. 92%, 28S rRNA) to known and unknown species of the same genus (Supplementary Table S3).
The most common symbionts were the pedunculate barnacle O. angulata (75.57%), its two congeners (O. neptuni and O. warwickii), Dianajonesia tridens (Young, 2001), and an acorn barnacle Chelonibia testudinaria; all of which were found in moderate abundance (> 10%). The egg-eating species C. indica, and the round worm, C. mitsukurii (Fig. 1D), were found in 2.09%, and 39.67% of specimens, respectively. A less common occurring group of symbionts (≤ 10%) included the ciliates Zoothamnium sp., and Epistylis sp. The prevalence of the remaining symbionts was low, and several occurred on a single crab (Semibalanus sp., Electroma papilionacea, and unknown gastropod, and Verrillactis paguri; Table 1). Symbiotic species colonized a variety of external sites of their crab hosts. The number of sites ranged from 1–3, and most common and dominant species occupied 2–3 sites (eg. Octolasmis species; Table 1). Only the rare ones were found on a specific site (ex. Notoplana sp. on the gill, Semibalanus sp., E. papilionacea on the carapace).
Female crabs hosted 18 species while only 12 species were found on male crabs. The central region had the highest number of symbionts (17) of which three species (Hydroides sp., E. papilionacea, and unknown gastropod) were unique to this area. In the north and south, 13 and 12 species, respectively, were found; Triticella flavar found only the north, while C. testudinaria occurred both in the central and in the southern crab populations. Five symbiont species (O. angulata, C. mitsukurii, C. testudinaria, Epistylis sp., and Zoothamnium chlamydis) were found only on small crabs (CW < 80 mm). The symbiont diversity increased with host size; 13, 12, 18 symbiont species were found in the 80–99 mm CW size group, the 100–119 mm size group and the > 120 mm size group, respectively. Nearly half (46.13%) of the P. pelagicus specimens carried one (22.96%) or two (23.17%) symbiont species. Uncontaminated crabs were 17.95%; while 3–5 symbionts were found on 27.35% hosts, and 8.56% were infected with more than 5 species (Supplementary Fig. S3). A single individual host was found to be infected with 8 different symbiont species (Fig. 1B and 1C).
The most six common symbionts (O. angulata, O. neptuni and O. warwickii, D. tridens, C. testudinaria, C. mitsukurii) and two lesser common ones (Zoothamnium sp., and Z. chlamydis) differed in prevalence and/or mean intensity among host crab populations according to area (north, central, and south – see Table 1). Infestation pattern also depended on crab gender. Five common species (three Octolasmis species, D. tridens, and egg predator C. mitsukurii) had significant differences either in prevalence and/or in mean intensity. Infestation of symbiont species increased with crab sizes as well. Six species showed significant differences in prevalence, and three species in mean intensity (Table 1).
When comparing crab populations and sexes with mean intensity, no species had statistically significant differences; while 8 (6 common, 2 less common) and 3 (2 common, 1 less common) displayed differences when comparing crab populations and sizes, crab sexes, and crab sizes (two-way ANOVA, p < 0.05). Multiple comparisons in three-way ANOVA (considered crab populations, sexes, and size groups) revealed only D. tridens showing a significant difference (Supplement File). No significant differences among host populations and sizes were observed.
There was variation in diversity indexes of symbiont assemblage at sites and populations. The central population (KH and PY) showed higher Shannon index and Evenness values, the north (HP and QN) population had higher values in the Simpson and Berger-Parker indices (more dominant, less diversity), while the south (KG and PQ) showed a higher species richness value. However, only the Simpsons index (proportion of individual numbers of dominant species) presented a significant difference between central and north populations (Table 2).
Discussion
The blue swimming crabs (Portunus spp.) are valuable as both a food source and an aquaculture product, playing a crucial role in marine ecosystems by contributing to the food chain and serving as habitats for various symbionts. Our study found no significant strong positive or negative effects of symbionts on the crabs’ features, although correlations between host traits and symbiont assemblage structure and diversity were observed without definitive patterns.
The high diversity of external and internal fauna was recorded from most body parts of the host species, P. pelagicus, with 18 ectosymbiotic species (belonging to 10 phyla) documented in Vietnam compared 15 species (from 3 phyla) in Australia (Shields, 1992). Both studies agree on the dominance of taxa such as Protozoa, Helminthes (Platyhelminthes and Nemertea), and Crustaceans, each represented by 4–6 symbiont species. Micro-symbionts, such as ciliate protozoa (Epistylis sp., Zoothamnium sp.) and Entoprocta (Loxosomella sp.), are frequently recorded in various crustacean hosts. However, species identification remains challenging due to the lack of distinguishing taxonomic characteristics and the tendency for their DNA to denature easily. For less abundant macro-symbionts (e.g., Hydroides sp., Notoplana sp., Semibalanus sp.), the problem is compounded by limited reference sequences available in biological databases (Valkiūnas et al., 2008). Notably, there is no GenBank sequence available for C. mitsukurii, a widely distributed roundworm species (Supplementary Table S3). Despite the recent increased focus on parasitology and symbiont studies, which are crucial for understanding biodiversity and ecology, many species remain undescribed. We advocate for comprehensive studies that combine morphological and molecular identification techniques to rapidly expand the database and gain thorough insights into these ecologically important taxa.
Morand (2015) proposed an ecological theory explaining the richness of parasite species and their effects, linking determinants such as latitudinal gradients, host body size, and geographical range. The symbiotic community structure is shaped by the biological characteristics and distribution of their hosts, closely related to the ecological environment (Morris et al., 2019). In this study, we observed that the composition of symbiotic species fluctuates according to geographical populations (North, Central, and South), as well as the sex and size of P. pelagicus. Positive relationships were detected between symbiont infection patterns (mean intensity) and crab sizes across different populations (8 species), as well as between infection patterns and crab sexes (3 species). These observations may be attributed to the fact that fully mature and intermolt hosts (large-sized crabs) provide a longer-lasting substrate for symbionts (Ameer Hamsa, 1982). Previous studies have also demonstrated significant variations in infection based on sex, size, and molting condition (Gaddes & Sumpton, 2004; Shields, 1992).
While a trend of symbiont species richness versus host geographical range was observed, the variation along the latitudinal gradient was not clear, as the central population displayed the highest diversity compared to populations in both lower (south) and higher (north) latitudes. This could indicate either an unclear trend at a small geographical scale or a hypothesis that requires further testing, particularly in the context of global climate change. These findings highlight the need for additional temporal and spatial sampling to better understand these patterns.
Overall, when each symbiotic species, especially common species, is evaluated separately, infection differences are clearly influenced by crab sex, size, and geographic range. In nature, hosts often harbor a complex symbiotic community (in this case, 18 species on P. pelagicus), which can have varied and complex effects on host populations. The analysis of 12 symbiont species (excluding rare species) revealed no clear tendencies in the data. However, diversity indexes may provide deeper insights into the biodiversity of symbiont assemblages. In this study, symbiont indexes correlated with the geographic ranges of hosts along the Vietnamese coastline. Notably, only the Simpson dominance index showed significant differences between two of the three host geographic populations. These findings highlight the necessity for further temporal and spatial sampling to gain a better understanding of these patterns.
The current significant findings partially reveal the symbiotic diversity and ecological interactions between symbionts and their natural host, P. pelagicus. Fine-scale geographical variations in symbiont community structure and diversity in relation to host traits enhance our understanding of their biology and help predict host-symbiont evolutionary dynamics. Further investigation into additional factors, such as intra- and interspecific competition and host exposure, especially concerning macro-symbionts, is needed to deepen our insights.