Introduction
Spotted scat, Scatophagus argus (Linnaeus, 1766) has a high nutritional value and delicious flavor (Mathew, 1988) and favored by many consumers, especially in Southeast Asian countries (Barry & Fast, 1992; Musikasung et al., 2006; Shao et al., 2004; Wongchinawit, 2007). In addition to its high value for food, this species has also a high market demand for ornamental fish (Barry & Fast, 1992; Gupta & Banerjee, 2014). S. argus is an omnivorous fish, their feeding habits are benthic animals, zooplankton and detritus. On the other hand, the feeding behavior of S. argus can change depending on the available food sources in the habitat (Hashim et al., 2014; Sivan & Radhakrishnan, 2011; Wongchinawit & Paphavasit, 2009). The fish is highly adaptable to fluctuations in salinity, they can live in both freshwater, brackish, and marine environments (Gandhi, 2002; Yoshimura et al., 2003). According to Fast et al. (1989), S. argus is an economically valuable species and has the potential to culture in tropical brackish water areas. In Vietnam, the fish has very high economic value and is commonly raised in coastal areas (Nguyen et al., 2022a).
Up to now, many studies on nutritional and food behavior have been conducted by several authors (Gandhi et al., 2014; Wongchinawit, 2007), but information on the diet, especially the dietary protein level, is very limited. Determination of protein levels in dietary feed plays an important role in aquaculture because it is a very expensive component (Mohseni et al., 2007). In other words, the amount of feed for aquatic animals normally occupies up to 50% total cost (El-Dahhar et al., 2016). Moreover, protein is the main nutritional component that significantly affects the growth and feed cost of fish (Oishi et al., 2010). A diet that insufficient protein will lead to slow growth performance of fish (Alam et al., 2008), on the contrary, if the diet provides too high a protein level for fish’s demand, it will be a wasted investment because fish cannot use it (Kim et al., 2016) and accelerate water environmental pollution (Xia et al., 2015). Normally, the optimal level of protein in a fish diet depends on the nutritional and feeding behavior of each species and the stage of development of the fish (Bowyer et al., 2013; Khoa et al., 2019; Prabu et al., 2017). In Vietnam, S. argus was typically cultured together with shrimp in earthen ponds and intensively cultured in high-density polyethylen (HDPE)-lined ponds. Fish was fed with shrimp pelleted feed or commercial finfish-floating feed, containing around 40% crude protein (Nguyen et al., 2022b). Therefore, accurately determining the optimal protein level in the diet of each species and stage of fish development is very important in aquaculture. The objective of this study was to determine the optimal protein level in diets for S. argus in the first stages of growth-out culture.
Materials and Methods
The present study was conducted in triplicates with four different crude protein treatments by using completely randomized design method. Treatment 1: (30% protein); Treatment 2: (34% protein); Treatment 3: (38% protein); Treatment 4: (42% protein).
Four experimental diets using different materials were prepared with protein levels of 30%, 34%, 38%, and 42% which were designated as CP30, CP34, CP38, and CP42, respectively. The compositions of materials which used for dietary formulation are described in Tables 1 and 2 (Ewan, 1989). The raw materials are used to formulate the experimental diets was analyzed for chemical composition as described by AOAC (2000). The food materials is mixed and extruded into 1 mm pellets using a feed pellet extruder (Model MDV-01, Electronic Equipment, Hanoi, Vietnam).
Adapted from Ewan (1989) with permission of CABI.
Mineral premix (kg–1 diets): Iron (1,262.8 mg); Manganese (454.5 mg); Copper (1,238 mg); Zinc (2,500.5 mg); Iodine (24.8 mg); Selenium (10 mg); Cobalt (100.5 mg).
The experiment was arranged in circle plastic tanks with a volume of 0.2 m3 using a separate circulating filtration system at 400 L/h (Fig. 1), weekly adding new filtered water as compensation for lost by evaporation. S. argus fingerlings from artificial reproduction in good health condition was obtained from trial production project of state-level (code NVQG-2023/DA.01). Fish was brought to the laboratory in oxygen filled polythene bags from Center of Research, Application, and Technology Transfer of the University of Agriculture and Forestry, Hue University. After acclimatization, the fingerling (3.56 cm; 2.94 g) were measured weight and length to determine the initial length and weight of fish, and then randomly stocked 20 fish per tank in triplicate groups. The fish were fed test diets in the form of dry pellets twice a day at a rate of 4% of their body weight per day for 60 days.
Temperature, salinity, pH and dissolved oxygen (DO) in each tank were monitored twice daily between 06:00 and 07:00 am and between 14:00 and 15:00 pm. By using a digital thermometer (model: CE 225908, Thermo Fisher Scientific, Waltham, MA, USA), hand held refractometer (Extech RF20), digital pH meter (Model: CE 224469), digital DO meter (Model: CE 225908), respectively. While, water quality of Total Ammonia Nitrogen (NH4+/NH3) (Hanna HI 700), NO3--N (Hanna HI 96728), PO4--P (Hanna HI 713) were measured once daily at 08:00 am.
At the beginning of the experiment, 30 fishes were randomly collected for initial measurement of length and weight. Experimental indices were calculated as follow:
- Fish growth rates: Specific growth rate (SGR) by length (SGRL) or by weight (SGRW) of fish is calculated according to the formula:
where X1 is the body mass or length at measurement time t1 and X2 is the body mass or length at measurement time t2.
- Survival rates (SR) (%):
- Feed conversion ratio (FCR):
- Protein efficiency rate (PER):
- Net protein utilization (NPU):
At the end of the experiment, fish was deeply anesthetized and then dissected to remove skin and bones (Fig. 2). Nine fish were randomly pooled from each replicate of dietary treatment and three sub-samples of each replicate from the pooled sample (n = 3 × 3) were analyzed for final body composition. Body compositions such as moisture, ash, crude protein and lipid were determined according to AOAC (2000) method. Moisture content was determined by drying in an oven (Memmert, Buchenbach, Germany) at 105°C until constant weight (approximately 24 h). Crude protein content was determined by distillation in a semi-automatic Kjeldahl protein system (UDK 132, VELP, Usmate, Italy). Crude lipid content was determined by solvent extraction method (diethyl ether) in the Soxhlet system (VELP Scientific 148/3). Ash was determined by complete combustion of organic matter at a temperature of 500°C–550°C in a furnace (Nabertherm, Lilienthal, Germany) for 7 h.
Responses of S. argus to different levels of dietary protein were measured by weight gain (%), FCR, PER, SGR (%) and body composition data. These response variables were subjected to one-way analysis of variance (ANOVA). To determine the significant differences among the treatments, multi-comparisons Tukey-Kramer honest significant difference (HSD) post-hoc test was employed by SPSS version 20. Statistical comparison tests were conducted at a level of significance of p = 0.05.
Results
The average temperature of the treatments was in the range of 27.0°C–27.8°C; pH 7.7–8.0; DO 5.5–5.7 mg/L; salinity 15.3; TAN 0.38–0.48 mg/L; N-NO3– 0.36–0.39 mg/L (Table 3). Daily water quality parameters such as temperature, pH, DO, and salinity during the experiment did not vary significantly among different treatments (p > 0.05). However, water quality in relation to nutrition (nitrogen and phosphorus) increased gradually with increasing time of experiment and dietary protein levels of the treatments (p < 0.05). The highest values of TAN, NO3––N, PO4--P were recorded at the highest protein level treatment, whereas, the lowest values of these parameters were also monitored at CP30 group.
At the end of the experimental period (60 days), the growth rates in length and weight, FCR, NPU, and PER of fish were significantly differences among the treatments of protein levels (p < 0.05) (Table 4). Higher protein levels in diets increased the growth performance and reduced FCR of fish (p < 0.05), however, no significance was found in growth rates and FCR of fish between treatment CP38 and CP42 (p > 0.05). On the contrary, PER and NPU increased with increasing the protein levels of diets from CP30 to CP38 (p < 0.05). Lower 34% or higher 38% protein contents in the diets were lower PER and NPU compared with protein contents of 34% or 38% (p < 0.05). No significant difference was found in PER or NPU between CP34 and CP38 treatments (p > 0.05). There were no significant differences (p > 0.05) in percentage survival among the treatments with more than 95%. Based on the second polynomial regression analysis of weight gain (%) and dietary protein levels (%), optimal dietary protein requirement for spotted scat was estimated to be 43.7% of dry matter (Fig. 3).
CP, crude protein; Lb and Wb, the length and weight of fish at the beginning; Lf and Wf, the length and weight of fish at the finish the experiment; SGRL (%/day) or SGRW (%/day) are the specific growth rate of fish in length or weight; SR (%), survival rate; FCR, feed conversion ratio; PER, protein efficiency rate; NPU, Net Protein Utilization.
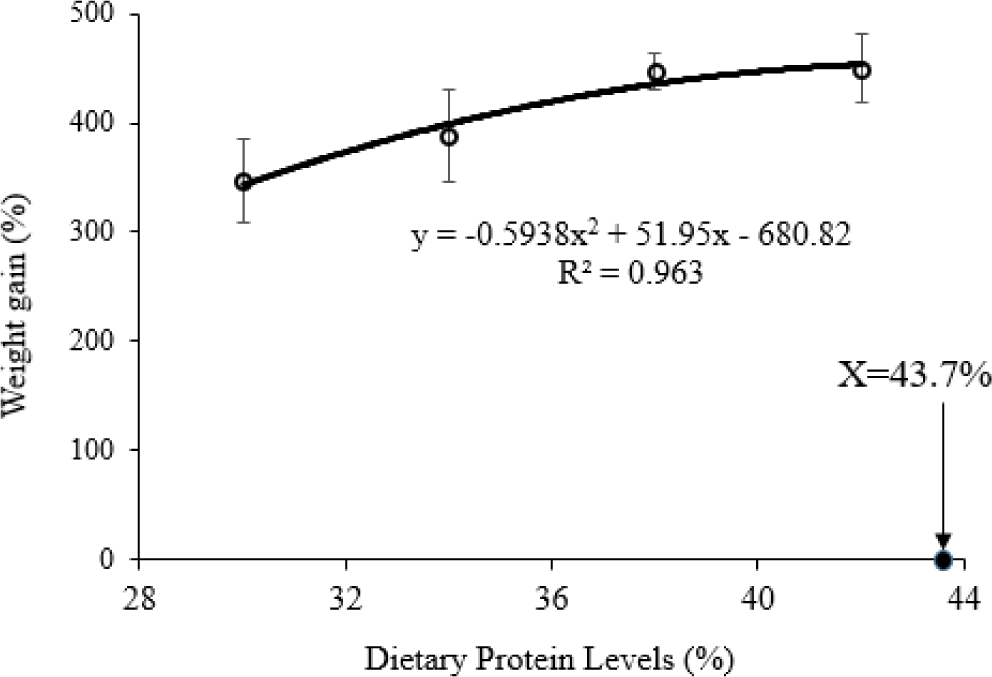
The approximate composition of fish muscle was fed with different dietary protein contents had statistical differences (p < 0.05) as described in Table 5.
The moisture content of fish carcase meat decreased gradually with the increasing protein levels in the diets. However, there were no significant differences in the moisture content of chemical compositions of fish meat at different diet treatments (p > 0.05). In contrast, significant differences were observed in crude protein, crude lipid, and crude ash samples of fish in different dietary protein treatments (p < 0.05). Significantly higher crude protein and crude lipid were found in fish fed with higher protein levels of diets (p < 0.05), but there was no significant in the crude lipid of fish body among treatments of CP34, CP38, and CP42 (p > 0.05). At lower protein contents of the diets of CP30 and CP34, crude ash trended to be significantly higher than those of CP38 and CP42 (p < 0.05).
Discussion
The above environmental conditions are suitable for the growth and development of fish (Boyd & Tucker, 1998) and in optimal range for growth performance of S. argus (Nguyen & Vo, 2021). Environmental data from Table 3 shows a similar trend for all treatments, except that TAN concentration was different between treatment of CP30 and other treatments (p < 0.05). Xia et al. (2015) reported that optimal protein level of diets reduced ammonia excretion. The environmental parameters of this research are within the acceptable limits for normal growth of marine fish (Munday et al., 2009).
According to Nguyen et al. (2022c), S. argus in the central region is different from the species in the southern region of Vietnam, even though they have the same scientific name but the morphology is different. Especially, this species has a very high economic value and is being farmed by polyculture and monoculture in earthen ponds or HDPE ponds in Central Vietnam. Therefore, research on the effects of protein content in diets for raising this fish species has great scientific and practical significance in reducing costs, improving economic efficiency as well as reducing negative impacts on the cultured environment. In addition, determining of optimal dietary protein content of diet also aims to recover as higher a protein content harvesting fish as possible. This study has proven that higher protein levels in the diet promoted fish growth and reduced FCR. Interestingly, the protein content in the 38% diet showed no statistical difference compared to the protein level of 42% in terms of growth rate, FCR, PER, and chemical composition of fish muscle. Our findings are consistent previous results of Ahmed & Ahmad (2020), who report that higher protein levels of the diets had a significant greater in weight gain, SGR, FCR, PER and body deposition of fingerling rainbow trout, Oncorhynchus mykiss. Similarly, Hien et al. (2023) demonstrate that the growth performance of S. argus fingerling was recorded in the treatment of 40% protein, but tended to decrease at higher 40% protein levels. Other similar results were obtained from yellow croaker Larimichthys polyactis juvenile by Zhang et al. (2022), Leopard Coral Grouper Plectropomus leopardus by Xia et al. (2015), and juvenile parrot fish, Oplegnathus fasciatus by Kim et al. (2016). The results of the present study are logical with the fact that S. argus is an omnivorous species (Nguyen et al., 2021), so its requirement for the dietary protein content may not be too high. This research demonstrates that increasing protein levels beyond 38% did not enhance the growth rate, FCR, and protein retention, but resulted in reduction of PER. The similarity results was also reported by El-Dahhar et al. (2016) for European Sea Bass (Dicentrarchus labrax) fingerlings. However, they reported that increasing dietary protein levels decreased the PER (El-Dahhar et al., 2016) and FCR increased after dietary protein exceeded the optimal levels (Zhang et al., 2022). According to Hien et al. (2023), the optimal protein level of the diet for the growth rate of S. argus juvenile was 40%, on the other hand, the lowest FCR was treatment of 35% protein. In contrast, Yangthong et al. (2023) have reported the optimal dietary protein levels for the juvenile stage of spotted scat was around 35% crude protein. These results were different from this study, attributed to the different sizes of fish for the experiment and experimental conditions. The present study showed that NPU was correlated with dietary protein levels, but there was not a significant difference between CP38 and CP42 treatments (p > 0.05). Tran et al. (2022) have documented that NPU was dependent on the balance of crude protein and crude lipid levels in the diets. They have demonstrated that the diets containing 9% crude lipid content increased NPU, compared to other crude lipid level treatments. However, there is no change in NPU as increasing in protein levels in the diets containing 6% or 12% crude lipid levels. This study fixed crude lipids around 9% in the diets, so NPU may only depend on the protein contents.
Fig. 3. Estimation of optimal dietary protein levels of spotted scat by second-order polynomial regression analysis.The chemical compositions of fish in the present study significantly increased with an increase in protein levels of the diets, except moisture content. The protein content of the body increased from lower to optimal protein levels in the diets but decreased from optimal level to beyond levels of diets (Ahmed & Ahmad, 2020). Whereas, protein retention by fish decreased with increasing dietary protein levels (Kim et al., 2016). The lipid content of fish bodies increased with an increase in protein levels of the diets was also reported by (Ahmed & Ahmad, 2020). Deng et al. (2011) suggest that the excessive protein level in the diets was deaminated and deposited into body fat or utilized as energy for metabolism (Wu & Gatlin, 2014). However, El-Dahhar et al. (2016) revealed that excessive dietary protein supplementation results in low body lipid deposition of fish. On the contrary to lipid content, this study shows that crude ash had an opposite trend with higher content at lower protein treatment. Zhang et al. (2022) demonstrate that the ash content of the body was not affected by dietary protein level. This has also been reported by many scientists for many fish species such as Colossoma macropomum (Oishi et al., 2010); O. fasciatus (Kim et al., 2016).
Conclusion
The present study demonstrates that the growth performance of S. argus was better when fed diets containing at least 38% crude protein. This protein level is further supported by a low FCR, PER, and high crude protein and lipid content of the body. The optimal dietary protein level for early stage grow-out culture of S. argus was 43.7%. Further studies about amino acids of the diets as well as body compositions should be conducted to elucidate for better dietary formulation of S. argus.