Introduction
Many natural products have been identified and characterized; however, the primary obstacle to the development of natural products is the sourcing of natural products in the quantities needed for drug development (Berrue et al., 2011). Accordingly, research is needed to ensure the sustainable production of natural products, starting with an understanding of their biosynthetic origin. Since many of these molecules are not only novel but also complex and challenging to synthesize, one of the biggest obstacles facing researchers and pharmaceutical companies today in the production and development of successful natural products is the supply issue (Berrue et al., 2011). Alternative methods for providing adequate quantities of pharmaceutical compounds for clinical testing and commercialization include genetic modification or microbial fermentation, as well as aquaculture of molluscs producing medicinally significant bioactive compounds. Molluscs have become a significant source of many distinct secondary metabolites that may be utilized in the creation of novel medications to treat a variety of fatal, both communicable, and non-communicable illnesses. It is difficult to commercialize new medications, and during the phases of development and approval, economic viability for long-term production must be taken into account.
Molluscs are incredibly diverse animals that have given humans access to a variety of resources, including food, colours, medicines, and shells. After arthropods, molluscs make up the second biggest animal phylum on Earth, accounting for about 7% of all living things. An estimated 200,000 species exist (Bouchet, 2006), of these about 5,000 species are found in fresh water (Seddon, 2000). Because of their diversity and ability to adapt to a wide range of habitats, including freshwater, brackish water, the marine environment, and terrestrial regions, molluscs have developed a variety of lifestyles and feeding niches. In addition to being regarded as a delicacy and enjoyed in many cultures, molluscs are a rich source of chemicals with medicinal activity. Molluscs have been utilized for food and medical purposes since the beginning of time. Throughout history, molluscs have been a valuable source of medicinal products for numerous cultures worldwide. Molluscs are used in several traditional medicines from ancient Roman medicine, China, India, South Africa etc. (Bonnemain, 2005; Prabhakar & Roy, 2009). Many bioactive natural products derived from molluscs are being developed for use as pharmaceuticals. The majority of research on natural products derived from molluscs concentrates on marine species, with few studies available regarding the natural products chemistry of freshwater and terrestrial molluscs.
Gastropods including slugs and snails make up the largest class of molluscs, accounting for over 80% of all species (Strong et al., 2008). Gastropods habitats are highly diverse and found in both freshwater and marine environments. Since, gastropods are highly biologically adaptive, it is possible that their secondary metabolism or chemistry is diverse. Gastropods have long piqued the interest of many researchers due to their intriguing biological activities and distinctive adaptations. Gastropods are frequently utilized as an affordable food source and in traditional medicine. The bioactive compounds of snails may find use in pharmaceutical and therapeutic research. Gastropods are also known for their high protein value, essential amino acids and low fat content. Gastropods, particularly snails, have long been utilized by numerous ethnic groups as food and medicine (Jadhav et al., 2023). Since ancient times, snails have been prized as delicacies, provided an inexpensive and sustainable source of animal protein in both developed and developing nations worldwide, and they are found throughout Asia and Africa (DeMarco et al., 2017). Gastropods particularly the snails may offer an affordable and sustainable source of animal protein for the people of developing nations.
Cipangopaludina spp., a vivipard gastropod, is a common freshwater snail found in India, China, and numerous other South East Asian nations (Baghele et al., 2022; Zhao, 2011). This species lives in pools, lakes, streams, and other bodies of water, where it feeds on organic particles and microorganisms. Its fresh meat is traditionally eaten as food and medicine in China (Zhao, 2011) and by different ethnic groups in the states of northeast India (Tripathy & Mukhopadhayay, 2015). C. chinensis is one of the many edible mollusc species found in Asia, Africa, and Europe. The flesh of C. chinensis has been regarded as a delicious and delicate food in many countries for more than a millennium. Moreover, Chinese traditional medicine has long used C. chinensis to treat liver and diabetes disorders (Zhao, 2011). Numerous studies, mostly in China, have already been conducted on the bioactive compounds of C. chinensis. Another member of the Viviparidae family, C. lecythis, is used both as food and medicine (Zhao, 2011). C. lecythis has been used as food and traditional medicine by numerous ethnic groups in the northeastern part of India. However, the uses of C. lecythis for treating various medicinal conditions are not scientifically substantiated till date and little attention has been devoted to the bioactive compounds from C. lecythis. This study was primarily aimed at preliminary screening of medicinally important bioactive compounds as there was no any earlier reports on the types of bioactive compounds present in C. lecythis. Hence, the purpose of this study was to identify and assess C. lecythis’s bioactive compounds in order to determine the nature of the primary ingredient that gives its medicinal properties. C. lecythis flesh and shell extracts using chloroform and methanol solvents were analysed using liquid chromatography-mass spectrometry (LC-MS) and gas chromatography-mass spectrometry (GC-MS). The presence of various medicinally important bioactive compounds justifies it uses for treating various ailments by traditional practitioners. Nevertheless, isolating and quantifying individual bioactive compounds and testing them for biological activity will undoubtedly produce positive results and aid in the discovery of new medications. This study provides the first report on the scientific validation of bioactive compounds from C. lecythis.
Materials and Methods
Adult specimens of C. lecythis (Fig. 1A) were collected from Waithou, Manipur, India during August 2023 and used for preparing sequential lipophilic (chloloform) and polar (methanol) solvent extracts. Snails were held in aerated water tanks for a maximum of 24 hours before processing. The shell of C. lecythis was removed by fracturing using a pressure applied at the point between the primary body whorl and spire. After removing the shell (Fig. 1C), opercula (Fig. 1B) were carefully separated from the snail flesh (Fig. 1D) using a sharp scalpel. The shell/ opercula combined and flesh were used for LC-MS and GC-MS analysis.
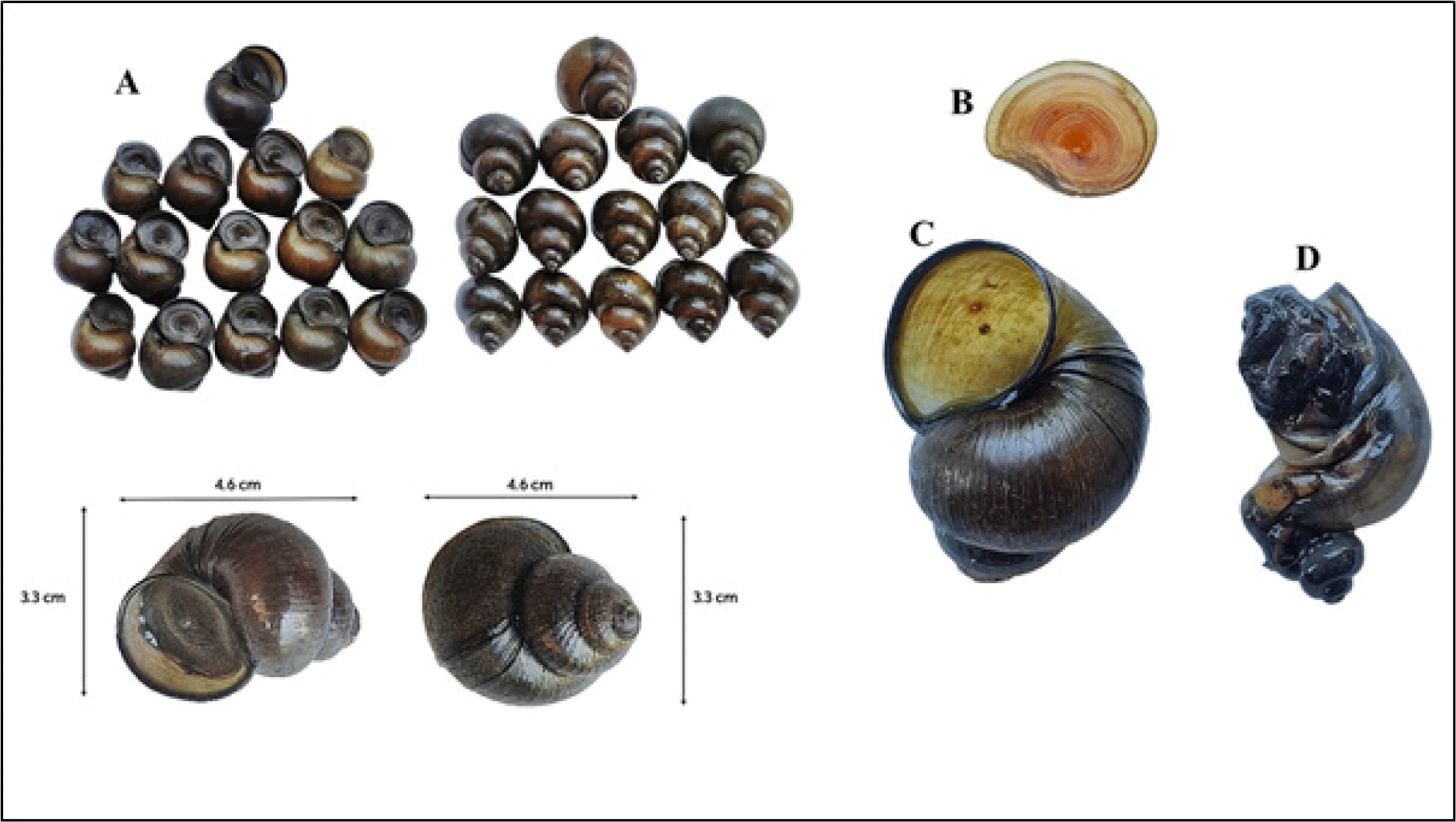
Shell-operculum and flesh tissue (10 g) of C. lecythis extracts were prepared using chloroform and methanol solvents (50 mL). Chloroform and methanol were HPLC grade (Sigma-Aldrich, St. Louis, MO, USA). The shell-operculum and flesh tissue from snails were homogenised using mortar and pestle in respective solvents and transferred to a glass vial. The solvent was decanted and replaced after every 2 h. This step was repeated for four times until the filtrate becomes colourless, with a final soak in solvent overnight in a fridge (Videocon 190 L, Videocon, Mumbai, India) at 4°C. The solvent extracts were combined and filtered using Whatman No.1 filter paper (Whatman, Buckinghamshire, UK). The resulting filtrate was dried under vacuum in rotary evaporator (Buchi, Flawil, Switzerland) at 40°C and 150 mb pressure and then solvents were removed. Dried extract (0.5 g) was dissolved in respective solvent (5 mL) for transfer into already pre-weighed glass vials. The tissue extract was weighed before storing at –20°C (Blue Star, India). The bioactive compound analysis of the snail extracts was performed by LC-MS using an Agilent 1260 Infinity II/LC-MSD iQ and volatile compounds of the snail extracts were separated using GC-MS Agilent 7890A GC (Agilent Technologies, Palo Alto, CA, USA) equipped with a MS (5977B VL MSD, Agilent Technologies).
C. lecythis chloroform and methanol extracts were analysed using LC-MS for the production of bioactive compounds. LC-MS analysis was undertaken using an Agilent 1260 Infinity II/LC-MSD iQ (Agilent Technologies) instrument according to the procedure described by Nongmaithem et al. (2017), using a 2.1 × 50 mm C18 column and gradient elution over 7 min with 5:95 acetonitrile/water with 0.1% formic acid to 95:5 acetonitrile/water with 0.1% formic acid. Mass spectrometry data were recorded 100–800 m/z in ESI+ and ESI-modes. The injection volume was 10 µl for all samples. Agilent ChemStation software was used to analyze the LC-MS data and predicted chemical formulae were used as input in the National Library of Medicine, USA when looking for matching bioactive metabolites. Statistical analysis was represented as mean with standard deviation in triplicate.
Volatile compounds from the snail extracts were separated using an Agilent 7890A GC (Agilent Technologies) equipped with a MS (5977B VL MSD, Agilent Technologies) according to the procedure described by Nongmaithem et al. (2017). The column temperature was programmed to increase from 40°C (after a 5-min hold) to 120°C at a rate of 3°C/min (after a 2-min hold), and then to 250°C at a rate of 8°C/min with a 10-min hold. The total running time of GC was 60 min. Identification and interpretation of the bioactive compounds present were done using the library database of National Institute Standard and Technology 2017 (NIST). Statistical analysis data were represented as mean with standard deviation in triplicate.
Results and Discussion
This study provides the first chemical assessment of flesh and shell-opercula of C. lecythis. The chemical analysis of flesh and shell-opercula of C. lecythis clearly showed the presence of numerous compounds with bioactive properties of medicinal significance. For the flesh and shell-opercula of C. lecythis, the bioactive characteristics or uses along with their retention time, molecular formula, molecular weight, and concentration of each component using polar (methanol) and lipophilic (chloroform) solvents were displayed in Tables 1–3.
Compounds | Molecular formula | Retention time (min) | Major ion m/z | Area % compound ± SD1) | Bioactive properties or uses | References |
---|---|---|---|---|---|---|
EicosaneCE | C20H42 | 36.09 | 281.1; 261.1 | 1.5 ± 0.29 | Anti-inflammatory, analgesic, and antipyretic and antifungal | Ahsan et al. (2017) |
1-OctadecanolCE | C18H38O | 45.86 | 295.3; 279.3 | 11.33 ± 0.45 | Antibacterial, antifungal, anti-larval | Falodun et al. (2009) |
OctacosanolCE | C28H58O | 47.07 | 292.2; 281.1 | 4.83 ± 0.55 | Anti-fatigue, anti-hypoxia, antioxidant, Anti-inflammatory, antitumor | Zhou et al. (2022) |
OctadecaneCE | C18H38 | 47.47 | 295.3; 286.0 | 4.2 ± 0.24 | Unknown | ND |
Cyclohexylmethyl octadecyl esterCE | C25H50O3S | 48.23 | 285.1; 277.1 | 5.39 ± 0.36 | Unknown | ND |
Heneicosane, 5-methylCE | C22H46 | 48.29 | 292.3; 281.2 | 3.94 ± 0.25 | Unknown | ND |
2-Azido-2,4,4,6,6,8,8-heptamethylnonaneCE | C16H33N3 | 49.40 | 284.1; 269.0 | 4.76 ± 0.17 | Unknown | ND |
Eicosane, 2,4-dimethylCE | C22H46 | 49.49 | 295.3; 287.1 | 3.88 ± 0.23 | Unknown | ND |
Nonyl tetracosyl etherCE | C33H68O | 50.39 | 295.3; 278.2 | 3.38 ± 0.37 | Unknown | ND |
2-(1-Methylcyclohexyloxy)-tetrahydropyranCE | C12H22O2 | 50.89 | 295.3; 281.1 | 3.80 ± 0.30 | Unknown | ND |
2-methylhexacosaneCE | C27H56 | 50.92 | 291.1; 281.1 | 2.92 ± 0.14 | Antibacterial and antifungal | Chakraborty et al. (2022) |
Dotriacontane, 1-iodoCE | C32H65I | 51.06 | 290.1; 275.2 | 3.88 ± 0.29 | Unknown | ND |
2-Methyl-cis-7,8-epoxynonadecaneCE | C20H40O | 51.31 | 291.1; 281.1 | 3.21 ± 0.39 | Unknown | ND |
Eicosane,9-cyclohexylCE | C22H52 | 52.08 | 291.1; 281.1 | 3.09 ± 0.07 | Antioxidant, laxative, antimicrobial, antitumor, anti-nociceptive | Mohamad et al. (2018) |
Nonyl tetracosyl etherCE | C33H68O | 52.39 | 292.2; 278.1 | 3.016 ± 0.15 | Unknown | ND |
Nonahexacontanoic acidCE | C69H138O2 | 52.41 | 294.3; 278.1 | 2.93 ± 0.20 | Unknown | ND |
Heneicosyl pentafluoropropionateCE | C24H43F5O2 | 52.59 | 294.3; 278.0 | 2.34 ± 0.37 | Unknown | ND |
Heneicosyl heptafluorobutyrateCE | C25H43F7O2 | 52.71 | 295.3; 279.3 | 1.5 ± 0.19 | Unknown | ND |
Cyclotetrasiloxane, octamethylME | C8H24O4Si4 | 22.22 | 281.0; 207.0 | 1.6 ± 0.24 | Hair conditioning agent, antiseptic, antimicrobial, and skin-conditioning | Mary & Giri(2016) |
2,6-Difluorobenzoic acid, 4-nitrophenyl esterME | C13H7F2NO4 | 27.18 | 207.1; 191.1 | 1.40 ± 0.31 | Unknown | ND |
4-Chlorobutyric acid, cyclohexylmethyl esterME | C11H19ClO2 | 39.85 | 283.0; 266.9 | 0.35 ± 0.07 | Unknown | ND |
Cyclotrisiloxane, hexamethylME | C6H18O3Si3 | 46.88 | 295.0; 281.0 | 5.52 ± 0.39 | Antimicrobial | Ibrahim et al. (2022) |
2-Methyldipyrrolo[1,2-a:2’,1’-c]pyrazine-3-carbonitrileME | C12H9N3 | 53.50 | 285.0; 271.0 | 4.73 ± 0.21 | Unknown | ND |
1,4-Benzenediol,2,3,5,6-tetrafluoro-,bis(butyl)etherME | C14H18F4O2 | 55.50 | 285.1; 270.9 | 3.83± 0.20 | Unknown | ND |
Our results clearly indicated that the major volatile compounds in the C. lecythis flesh contains 1-Octadecanol, chloroform extract (CE) cyclotrisiloxane, hexamethyl methanol extract (ME). The most prevailing volatile compounds in the flesh by the peak area obtained were identified as and hexamethylcyclotrisiloxane and 1-Octadecanol (Table 1 and Fig. 2). According to previous reports, hexamethylcyclotrisiloxane was reported to have antimicrobial, antioxidant, and anti-inflammatory properties (Ibrahim et al., 2022). These properties have also been reported from snails, Eobania vermiculata, Theba pisana, and Monacha obstructa (Sallam et al., 2009). Remarkably, hexamethylcyclotrisiloxane was the main compound found in C. lecythis flesh. There have been reports of the antibacterial, antifungal, and anti-larva properties of the bioactive compound, 1-Octadecanol (Falodun et al., 2009). Pringgenies et al. (2015) also reported the antibacterial property of 1-Octadecanol from the soft-bodied gastropod nudibranch, Jorunna funebris. Recently, it was also reported that snail extract could be used in treating wrinkled or burned skin (Noothuan et al., 2021). The biological properties of snail extracts are well recognized, and they are utilized in traditional medicine to treat a wide range of illnesses.
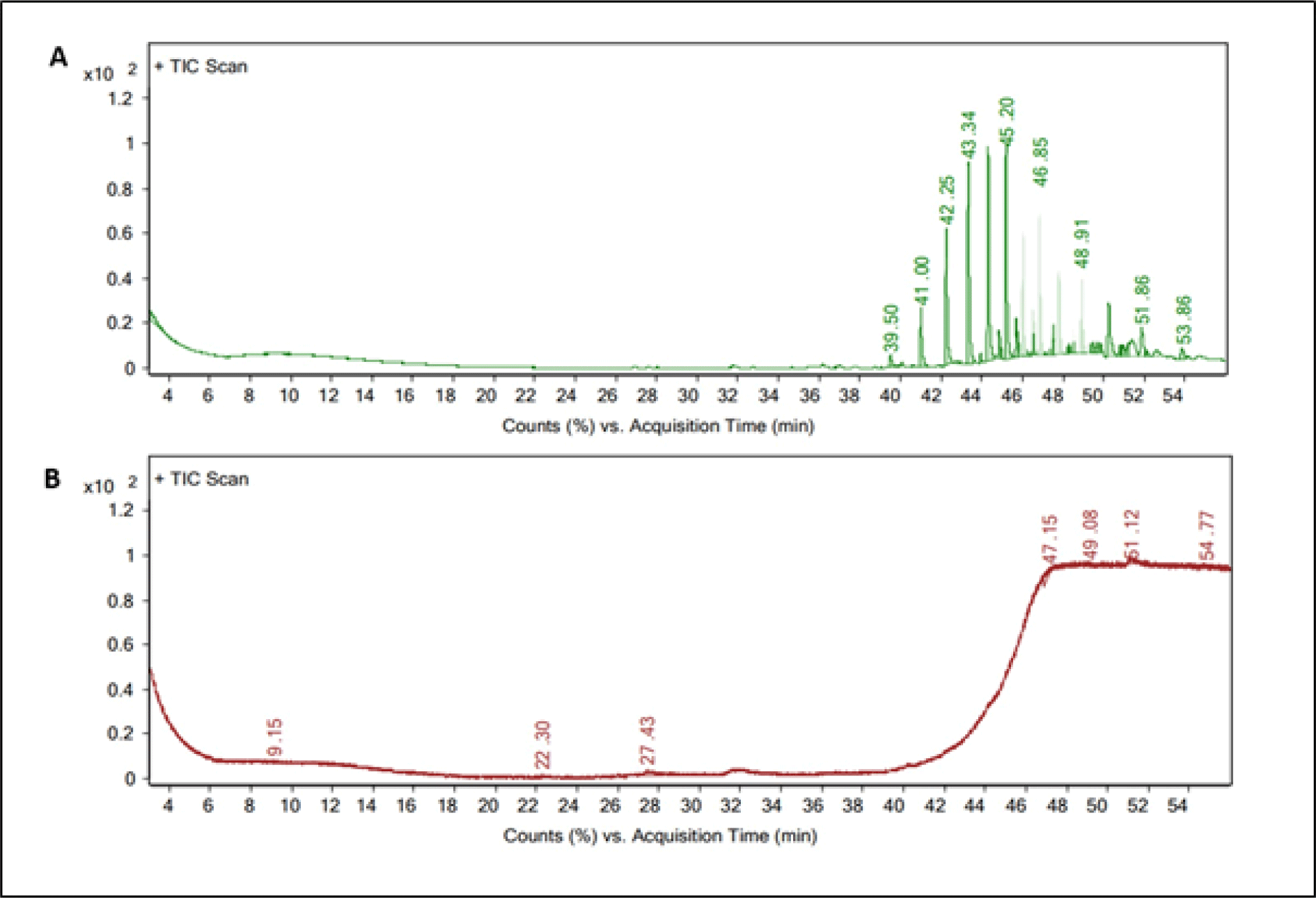
The main bioactive component found in the shell-opercula of C. lecythis extract was dihydrotachysterol (ME) (Table 2). A number of additional minor bioactive volatile compounds was also found (Table 2 and Fig. 3). Dihydrotachysterol, a synthetic hydrogenated analog of vitamin D is required for normal bone mineralization, calcium and phosphate absorption and utilization, and for enhancing calcium deposition (Cordy, 1977). Light catalyzes the rearrangement of ecdysterone in the enol form to penta-hydroxycholecalciferol or hexahydroxy-dihydrotachysterol, and has been previously reported that ecdysterone plays a role in the shell calcification of snails (Whitehead & Saleuddin, 1978). Dihydrotachysterol, which has been found in the shell and opercula of C. lecythis, might have originated from the environment or from food sources.
Compounds | Molecular formula | Retention time (min) | Major ion m/z | Area % compound ± SD1) | Bioactive properties or uses | References |
---|---|---|---|---|---|---|
EicosaneCE | C20H42 | 36.10 | 261.1; 253.2 | 2.42 ± 0.27 | Anti-inflammatory, analgesic, and antipyretic and antifungal | Ahsan et al. (2017) |
HeneicosaneCE | C21H44 | 39.43 | 281.1; 267.2 | 3.34 ± 0.42 | Unknown | ND |
Pyran-4-carboxylic acid, 4-(4-methoxypenyl)-tetrahydroCE | C13H16O4 | 44.51 | 293.1; 282.0 | 9.05 ± 0.14 | Unknown | ND |
Sulfurous acid, cyclohexylmethyl octadecyl esterCE | C25H50O3S | 48.20 | 292.2; 283.0 | 3.03 ± 0.16 | Unknown | ND |
Eicosane, 2,4-dimethylCE | C22H46 | 48.27 | 295.3; 279.3 | 4.95 ± 0.14 | Unknown | ND |
2-Thiopheneacetamide, N-(2-ethylhexyl) CE | C14H23NOS | 49.41 | 289.1; 281.1 | 2.94 ± 0.15 | Unknown | ND |
Cyclohexane, docosylCE | C28H56 | 50.39 | 292.2; 279.3 | 1.58 ± 0.35 | Unknown | ND |
1-EicoseneCE | C20H40 | 50.69 | 296.3; 281.1 | 2.03 ± 0.21 | Unknown | ND |
2-MethylhexacosaneCE | C27H56 | 50.90 | 295.3; 281.1 | 1.42 ± 0.33 | Antibacterial and antifungal | Chakraborty et al. (2022) |
22-Tricosenoic acidCE | C23H44O2 | 51.40 | 291.1; 273.0 | 4.22 ± 0.25 | Unknown | ND |
Docosyl octyl etherCE | C30H62O | 52.58 | 292.3; 279.2 | 1.69 ± 0.22 | Unknown | ND |
1-Dodecanol, 2-octylCE | C20H42O | 54.50 | 293.2; 278.3 | 1.33 ± 0.28 | Unknown | ND |
Hexane, 3,3-dimethylME | C8H18 | 14.44 | 127.1; 113.0 | 1.01 ± 0.12 | Unknown | ND |
Butanoic acid, 2-methyl-, 1,2-dimethylpropyl esterME | C10H20O2 | 21.36 | 207.0; 126.0 | 2.99 ± 0.31 | Unknown | ND |
Nonane, 5-(1-methylpropyl)ME | C13H28 | 26.79 | 253.0; 281.1 | 3.95 ± 0.17 | Unknown | ND |
Oxirane, 2-methyl-3-propyl-,cisME | C6H12O | 26.96 | 155.0; 130.1 | 0.92 ± 0.14 | Unknown | ND |
Fumaric acid, pent-4-en-2-yl propyl esterME | C12H18O4 | 27.25 | 207.0; 191.1 | 4.56 ± 0.38 | Unknown | ND |
1,4-Benzenediol,2,3,5,6-tetrafluoro-, bis(butyl) etherME | C14H18F4O2 | 32.71 | 281.0; 207.1 | 1.59 ± 0.21 | Unknown | ND |
Cyclotrisiloxane, hexamethylME | C6H18O3Si3 | 42.33 | 281.0; 267.0 | 3.31 ± 0.40 | Antioxidant and antidiabetic | Ibrahim et al. (2022) |
OctacosanolME | C28H58O | 48.09 | 292.3; 284.0 | 1.82 ± 0.36 | Anti-fatigue, antioxidant, anti-inflammatory, antitumor | Zhou et al. (2022) |
Eicosane, 2,4-dimethylME | C22H46 | 48.29 | 294.4; 282.1 | 2.54 ± 0.24 | Unknown | ND |
Dotriacontane, 1-iodo ME | C32H65I | 49.39 | 295.3; 282.1 | 4.88 ± 0.43 | Unknown | ND |
Dihydrotachysterol ME | C28H46O | 50.86 | 275.2; 282.0 | 12.84 ± 0.12 | Synthetic analogue of vitamin D, bone mineralization | Cordy (1977) |
Nonadecyl heptafluorobutyrateME | C23H39F7O2 | 52.40 | 294.3; 279.3 | 3.89 ± 0.13 | Unknown | ND |
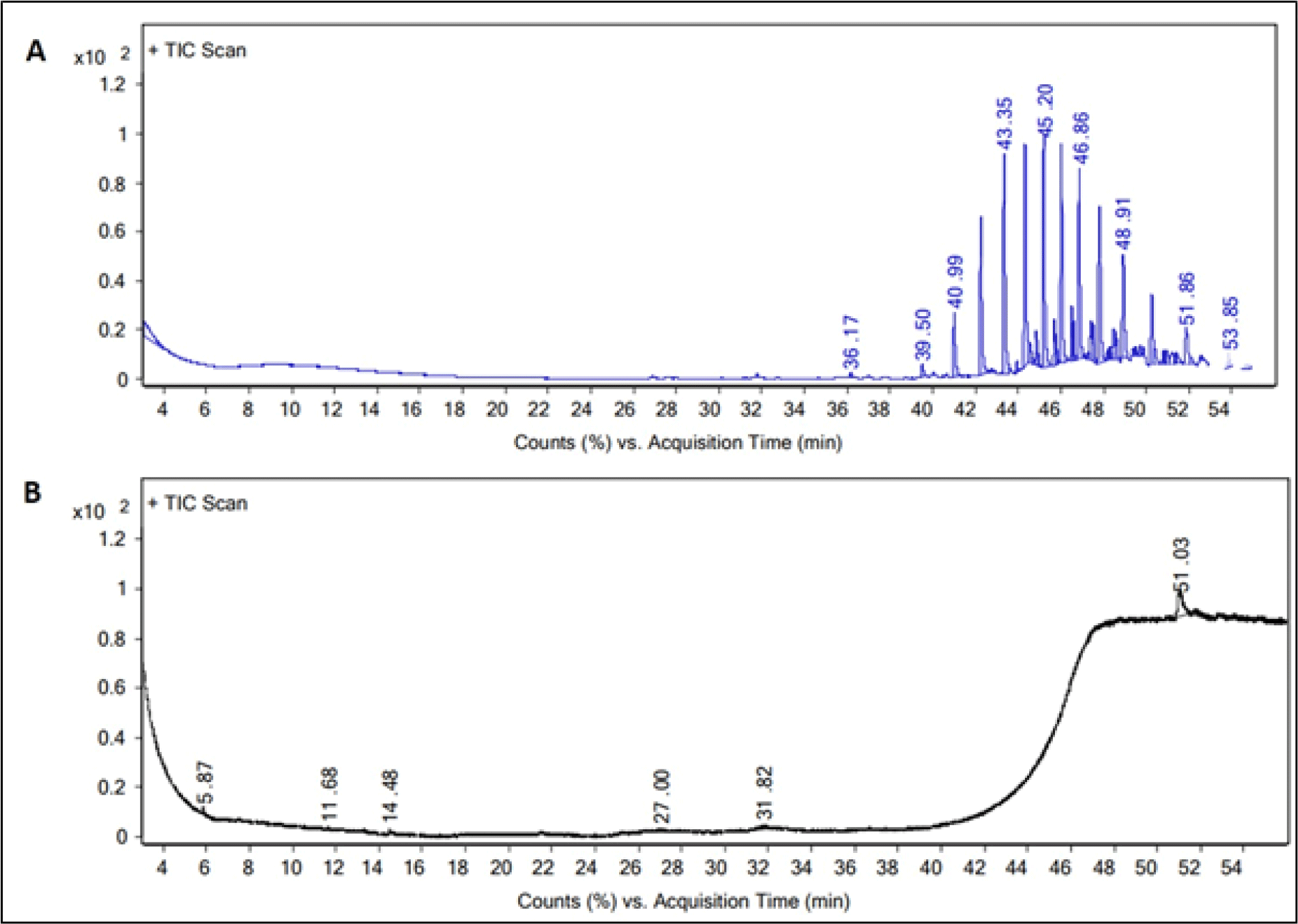
Chitin and 6-gingerol were among the major compounds identified in the flesh using LC-MS methanol extract (Table 3). Based on the peak area obtained, chitin was the most prevalent compound in the flesh (Fig. 4). It is interesting to note that chitin was found in the flesh of C. lecythis rather than in the shell (Fig. 4). Chitin has been reported to be present in the snail foot muscle of Charonia tritonis, a giant triton snail, and Chrysomallon squamiferum, a scaly-foot snail (Zhang et al., 2021). The mandible of Helix pomatia was found to contain chitin, according to Tóth & Zechmeister (1939), and the snail’s gut contained the chitin-degrading enzyme chitinase. According to Yonezawa et al. (2016), chitin in mollusc shells was known to be crucial to the calcification of the shell. Chitin, a natural polysaccharide, is frequently present in the arthropods, including crabs, shrimp, and insects, as well as in the shells of bivalves, but it is less common in the shells of snails. Chitin is recognized for possessing special biochemical qualities, non-toxic, biocompatible, and biodegradable biopolymer with potential uses in medicine. Chitin is also found to be utilized in tissue engineering, wound dressings, antimicrobial agents, anti-aging cosmetics, and vaccine adjuvants (Ali-Komi & Hamblin, 2016).
Sample details | Compounds | Molecular formula | Retention time (min) | Major ion | Area % compound ± SD1) | Bioactive properties or uses | References |
---|---|---|---|---|---|---|---|
Flesh | ChitinCE | (C8H13O5N)n | 1.283 | 203.1; 202.0 | 29.21 ± 0.41 | Tissue engineering, wound dressings, Antimicrobial agents, antiaging cosmetics, and vaccine adjuvants | Ali-Komi & Hamblin (2016) |
Flesh | 6-gingerolCE | C17H26O4 | 1.729 | 294.7; 236.0 | 29.03 ± 0.17 | Anticancer activities, anti-inflammation, and anti-oxidation | Wang et al. (2014) |
Flesh | ChamazuleneME | C14H16 | 1.176 | 188.9; 185.0 | 16.45 ± 1.01 | Antioxidant properties and reverses Osteoarthritic inflammation | Capuzzo et al. (2014) |
Flesh | Carotene-ZetaME | C40H60 | 1.488 | 541.7; 540.8 | 7.07 ± 0.78 | Unknown | ND |
Flesh | HesperidinME | C28H34O15 | 1.719 | 742.6; 637.0 | 5.45 ± 0.47 | Antioxidant, antibacterial, antimicrobial, anti-inflammatory and anti-carcinogenic | Pyrzynska (2022) |
Flesh | Linoleic acidME | C18H32O2 | 2.374 | 279.7; 201.6 | 7.12 ± 0.33 | Anti-inflammatory properties | Özçiçek et al. (2023) |
Shell/opercula | AllantoinCE | C4H6N4O3 | 1.822 | 159.7; 116.9 | 20.31 ± 0.38 | Antioxidant, anti-inflammatory Cytoprotective |
Dinica et al. (2021) |
Shell/opercula | LupanineME | C15H24N2O | 1.117 | 249.0; 232.9 | 8.26 ± 0.09 | Antioxidant, anti-cholinergic and antidiabetic activity | Kolak et al. (2011) |
Shell/opercula | Syringic acidME | C9H10O5 | 1.228 | 197.5; 195.8 | 12.22 ± 0.25 | Anti-oxidant, antimicrobial, anti-inflammatory, antiendotoxic | Srinivasulu et al. (2018) |
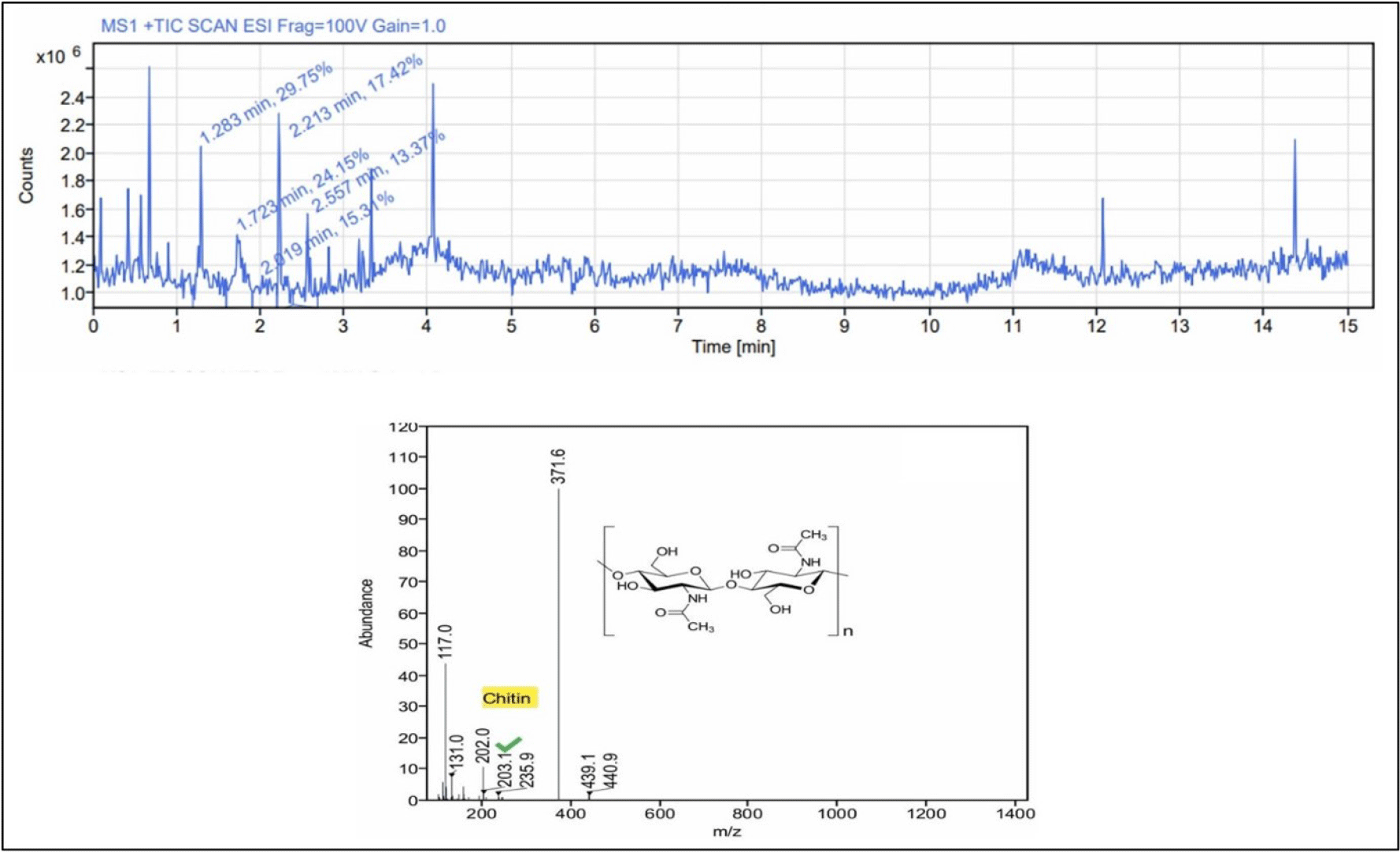
This study also found that linoleic acid, which is commonly present in snails and slugs, has anti-inflammatory qualities (Özçiçek et al., 2023). Essential fatty acids such as linoleic acid play a crucial role in maintaining nerve blood flow by being a structural component of the phospholipids that make up neuronal membranes (Zhu et al., 1994).
This investigation also unveiled 6-gingerol, another significant bioactive substance from the flesh of C. lecythis. 6-gingerol from the snail Achatina fulica was also reported by Ajisafe & Raichur, (2023), and their findings suggested that 6-gingerol may be useful for cartilage tissue engineering and therapeutic repair. The mucus secretion from A. fulica exhibited wound-healing and anti-apoptotic properties due to it. Anticancer, anti-inflammatory, and anti-oxidant properties of 6-gingerol have earlier been reported by Wang et al. (2014). The fact that C. lecythis contains 6-gingerol demonstrates how this snail is used in traditional medicine.
The shell and opercula of C. lecythis extract contained major bioactive compounds that were likely to be allantoin as well as several other minor bioactive compounds of medical significance (Table 3 and Fig. 5). Allantoin was the most prevalent compound in the shell and opercula extract based on the peak area obtained (Fig. 5). Allantoin was known to possess cytoprotective, gastroprotective, antioxidant, and anti-inflammatory properties (Dinica et al., 2021). Allantoin, an imidazolidine-2,4-dione, is a safe and efficient active ingredient for skin protection that is primarily used in modern medicine and cosmetics. It is utilized in over-the-counter cosmetics as well as other commercial goods like shampoos, conditioners, sun protection products, and oral hygiene products (Dinica et al., 2021).
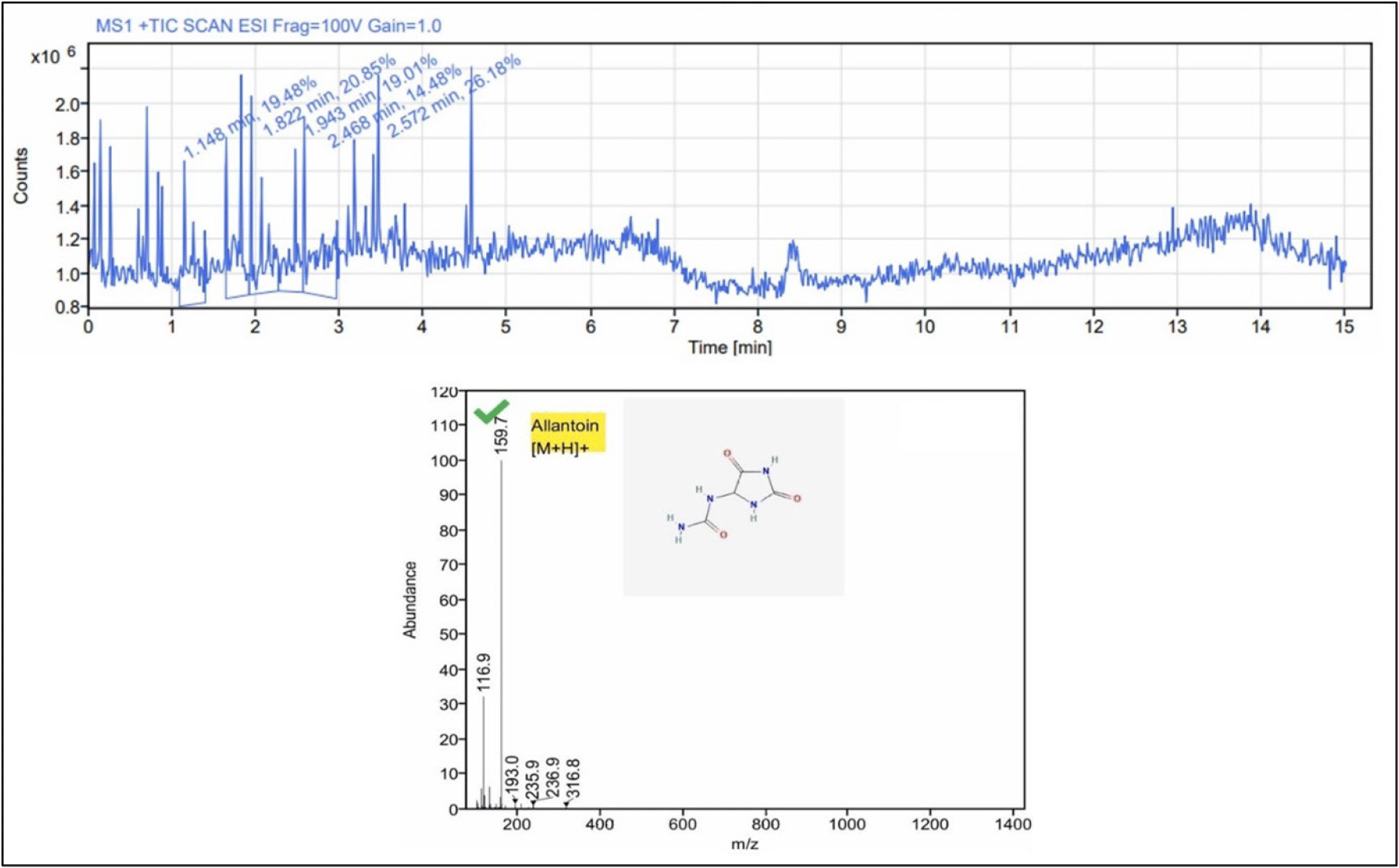
Allantoin was used as an ingredient in cosmetic skin creams, was previously identified as coming from the garden snail, Helix aspersa. El Mubarak et al. (2013) established a protocol for the simultaneous detection of allantoin and showed that there was a notable variation in the amounts of these compounds amongst various cosmetic creams, which may indicate the product’s quality or the effectiveness of the snail mucus content. The positive bioactive effect of the compound was largely driven by the quantity/volume used for specific efficacy (El Mubarak et al. 2013). Given the toxicity of certain natural products, one should never assume that using traditional or natural medicine is safe. Therefore, quantification of the specific dose is a must needed step of any drug discovery protocol. This may be the reason that the traditional preparation techniques employed the method which may be able to lessen or eliminate the harmful elements in some traditional medical systems. The pharmaceutical industry uses only the shells of the mollusc species with toxic venoms such as neogastropods and cone snails for harnessing only the good compounds while leaving the toxic ones present in the flesh. Since C. lecythis extracts could be make widely available, highly affordable, and utilized in traditional medicine, they may prove to be a promising natural alternative to synthetic drugs in therapeutic products.
The chemical studies on molluscs have led to the isolation of several bioactive primary and secondary metabolites. The molluscs themselves may have synthesized the bioactive metabolites, or they may have obtained them from food sources or the microbial symbionts (Ngangbam et al., 2019). Snails are known to produce slime, a viscous secretion, which is valuable and gaining interest due to its potential applications in medicine. There are numerous studies which have examined and verified the beneficial effects of bioactive compounds found in gastropods, such as their antibacterial, anti-inflammatory, antioxidant, anti-tumoral, anti-aging, tissue regeneration, wound healing, and numerous other attributes (Dinica et al., 2021). The biological activities of snails have garnered a lot of attention lately because of their diverse and possibly beneficial properties.
Conclusion
This study provides the first evidence of the presence of medicinally significant bioactive compound from C. lecythis, a freshwater snail. Bioactive compounds of pharmacological importance such as chitin, 6-gingerol, linoleic, hexamethylcyclotrisiloxane, eicosane, 1-octadecanol, octacosanol, eicosane, 9-cyclohexyl, nonyl tetracosyl ether and octamethyl were among the main compounds found in the flesh; whereas, allantoin, dihydrotachysterol and 2-methylhexacosane were identified in the shell and opercula extract of C. lecythis. The presence of these bioactive compounds justifies the use of these snails for treating various human ailments by traditional healers. Further investigation is required in finding the concentration, quantification and methods standardization of the target bioactive compound that may be used for future medical research and comprehending their biosynthetic origin through the use of an omics approach. Overall, C. lecythis, an underexplored freshwater snail could be a valuable source of bioactive compounds for application in human medicine and also significant for the pharmaceutical industry in terms of sustainable drug manufacturing.