Introduction
The occurrence of microplastics (MPs) in both freshwater and marine environments is wide-ranging, with varying concentrations from a few particles per liter to thousands of particles per cubic meter (Eerkes-Medrano et al., 2015). As the production and use of plastic products continue, it is anticipated that the quantity of MPs in water bodies will increase in the future (Jambeck et al., 2015). MP pollution is a complex and multifaceted issue that necessitates combined efforts from individuals, governments, and industries to reduce its negative effects and bring sustainable solutions. The small size of MPs makes them easily ingestible, and their persistence in the environment facilitates their entry into the food chain. These characteristics render MPs potentially hazardous to both human health and the environment. Fish subjected to MP exposure are susceptible to a range of detrimental effects, including tissue damage, oxidative stress, disruptions in the expression of immune-related genes, and diminished antioxidant defenses. Furthermore, these fish may exhibit signs of neurotoxicity, experience growth retardation, and display abnormal behavioral patterns (Lee et al., 2023). In the case of human exposure to MPs, it can occur via multiple routes such as ingestion, inhalation, or direct skin contact. Following exposure to MPs, humans may undergo oxidative stress, cytotoxicity, neurotoxicity, disturbances in the immune system, and the potential migration of MP particles to other bodily tissues (Bhuyan, 2022).
Plastic waste finds its way into oceans and can accumulate in riverbeds, where it may either settle or be re-suspended during periods of high-water flow (Waldschläger & Schüttrumpf, 2019). Environmental stressors like physical abrasion, high temperatures, and exposure to UV-B radiation contribute to the breakdown of plastic waste into smaller plastic particles known as MPs (Song et al., 2017). The presence of MPs was initially observed in coastal waters of North America during the 1970s (Carpenter et al., 1972). Nonetheless, the presence of MP pollution remains a substantial worldwide issue, with their detection reported in various aquatic settings such as oceans, rivers, lakes, estuaries, reservoirs, and even in harsh environments like Antarctica and the Arctic Ocean (Bellasi et al., 2020; Cunningham et al., 2022; Henny et al., 2022; Villegas et al., 2021). The durability, accumulation, and harmful effects on aquatic organisms make MPs an increasingly concerning environmental issue (Wardrop et al., 2016). The small size range of MPs, similar to natural food particles, leads to inadvertent ingestion by organisms (Wright et al., 2013). Species inhabiting aquatic ecosystems, spanning various trophic levels, readily ingest MP particles resembling their natural food sources like plankton. This ingestion leads to physical complications such as intestinal obstruction, diminished predator evasion capabilities, modified feeding patterns, and decreased energy levels. MPs have negative impacts on the growth, survival, and reproductive success of affected individuals (Dris et al., 2015). MPs not only impact individual organisms but also alter their habitats and influence population dynamics (Wright et al., 2013). Moreover, MPs can absorb other environmental pollutants like heavy metals, polychlorobiphenyls, polycyclic aromatic hydrocarbons, and dichlorodiphenyltrichloroethane as well as serve as vectors for the accumulation and transfer of toxins to organisms (Ziccardi et al., 2016).
The escalating concern regarding the impact of MP pollution on aquatic ecosystems and public health has gained prominence in Bangladesh. Recent studies have uncovered the occurrence of MPs in diverse marine and freshwater environments throughout the country (Rezania et al., 2018). Significant level of MP abundance was found in the Meghna River estuary (Hossain et al., 2023), Buriganga River (Islam et al., 2022), and Pasur River in Bangladesh (Nawar et al., 2023). The Karnaphuli River, which originates in Mizoram state, India, holds immense significance for navigation, fishing, transportation, and industrial activities in Chattogram City and the hill tracts regions of Chattogram, Bangladesh (Siddique & Akter, 2012). However, the hydrobiology of the river has been profoundly affected by pollutants from its tributaries, canals, and smaller rivers, which carry a significant volume of contaminated water, solid garbage, sewage discharge, and other types of pollutants (Hossain et al., 2005). Additionally, the banks of the Karnaphuli River accommodate approximately 800 industrial units, including chemical industries, textile mills, and fish processing plants, which discharge pollutants indiscriminately, exceeding safe limits and contributing to the generation of MPs. While the study of MPs in marine environments has been conducted for over 45 years, research in freshwater environments remains limited (Blettler et al., 2018), and investigations on MPs in Bangladesh are still in the nascent stage. Therefore, it is of utmost importance to obtain an extensive understanding of the identification and measurement of MPs particles in the aquatic ecosystems of Bangladesh. This study aims to assess the current abundance of MPs, seasonal variations in abundance, and physicochemical characteristics such as type, shape, size, color, and polymer composition at two highly industrialized areas, Avoimitro Ghat, and Kalurghat, along the Karnaphuli River in Chattogram. The findings of this research will provide essential information about MP pollution, enabling policymakers, environmentalists, and researchers to formulate effective strategies for mitigating MP pollution and safeguarding the health of the Karnaphuli River ecosystem.
Materials and Methods
The Karnaphuli River in Bangladesh is an ideal site for MPs research because of its high level of urbanization and industrialization, ecological significance, high population density, and lack of research (Sarker et al., 2020). There are several polluted areas of the Karnaphuli River including Chaktai Khal, Rajakhali Khal, Avoimitro Ghat, Kalurghat, and several others. For this study, the highly industrialized areas: Avoimitro Ghat and Kalurghat were chosen because of the presence of fertilizer factories, oil refineries, ship-breaking, and fish processing industries play a substantial role in the pollution of the Karnaphuli River by MPs.
A map of the study area is shown in Fig. 1.
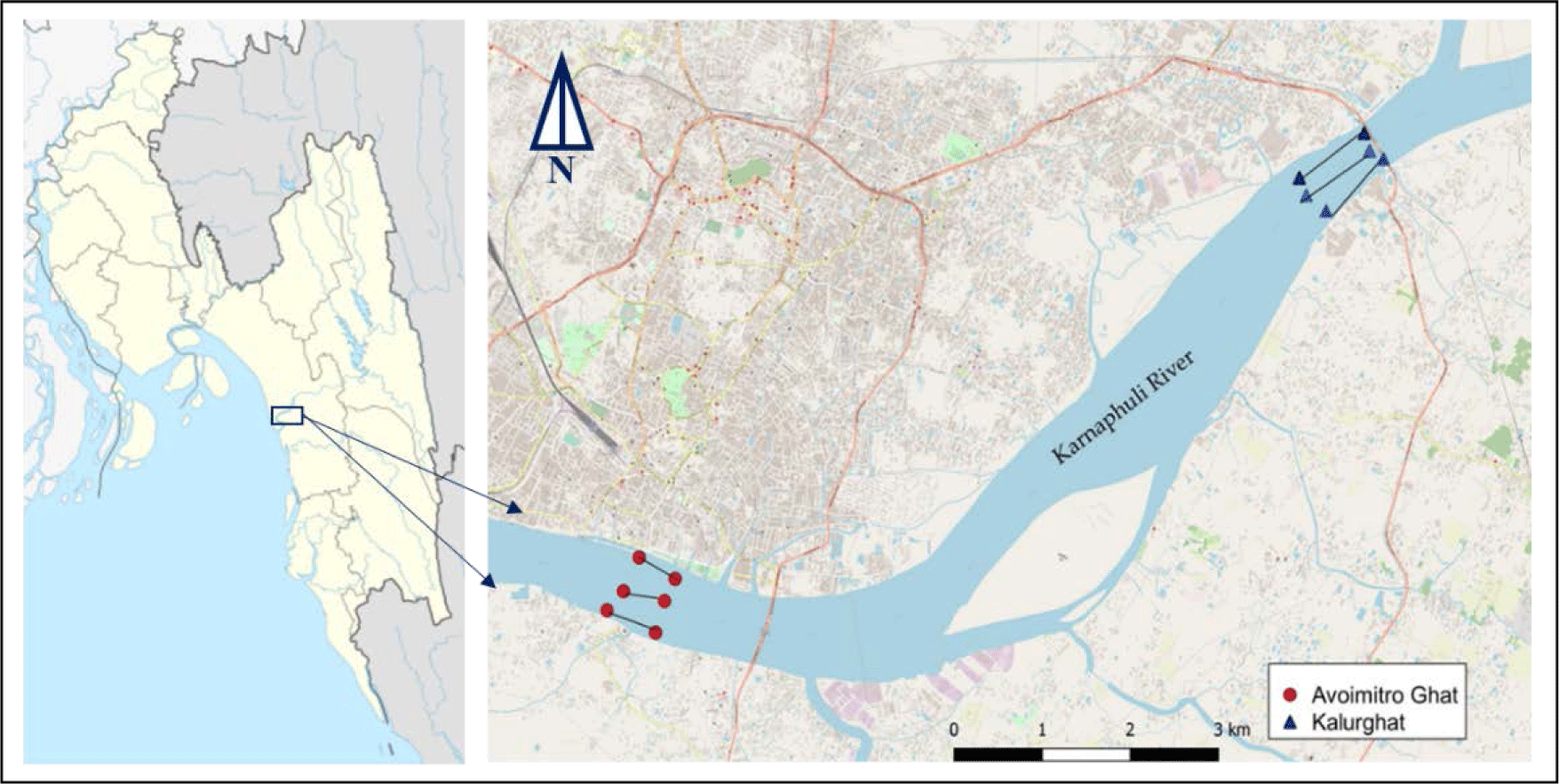
The sampling period, spanning 8 months, was divided into two distinct seasons: (1) the wet season, occurring from July to October 2022 and (2) the dry season, spanning from November 2022 to February 2023, based on Bangladesh’s temperature and rainfall patterns. Water samples from the surface were collected from the designated study areas, as the surface layer is recognized as the most contaminated (Willis et al., 2017). Stainless steel buckets were utilized for sample collection, and precautions such as wearing gloves and cotton clothing were taken to minimize potential external MP contamination during the sampling process. For sample collection, three transects were established, each covering a length of 500 m. Two of these transects were located near the river banks and the third was positioned in the middle of the river. Each transect was sampled twice to obtain replicates. Immediately after collection, the samples were transferred into airtight 500 mL jars. To preserve the physicochemical characteristics, the samples were promptly transported to the laboratory and stored at a temperature of 4℃ (Leslie et al., 2017).
The extraction of MPs was conducted following the protocols of the National Oceanic and Atmospheric Administration with certain modifications (Masura et al., 2015). The samples were passed through sieves with pore sizes of 5 mm and 300 µm, adhering to the ISO standard (ISO, 2017). Plastics trapped in the 5 mm sieve were taken in a glass jar, while those retained in the 300 µm sieve were gathered in a pre-weighed 500 mL beaker. To remove organic matter and biological tissues from the supernatant, a mixture of 0.05 M Fe2SO4·7H2O (20 mL) and 30% H2O2 (20 mL) was utilized and this procedure was continued until the solution turned clear. The digestion mixture was subjected to hot digestion on a hotplate, maintaining a temperature below 70℃. Once the digestion process was finished, the mixture was taken off the hotplate and left to cool down. To separate low-density MPs (below 1.20 g/cm3), a saturated sodium chloride (NaCl) solution of 1.2 g/cm3 density was prepared, mixed with the sample water, and heated to 70℃. For high-density MPs, a ZnCl2 solution (1.6 g/cm3) was used as a flotation medium. The density separation process followed the method of Coppock et al. (2017) with some modifications, employing a PVC pipe density separator (100 × 200 × 480 mm). The supernatant, separated by density, was gathered and passed through a special funnel with a paper filter made of cellulose nitrate, which had a width of 47 mm and pore size of 0.45 µm, making the filtration process slow. The solid particles that stayed on the filter were moved to a fresh glass dish for further examination.
A total of 4,098 MP particles were analyzed in this study. MPs were identified through two methods: direct observation with naked eyes for MPs greater than 3 mm, and microscopic examination for MPs smaller than 3 mm in size. MPs less than 3 mm were placed in a petri dish that had been previously cleaned, and their particle size was determined using ImageJ software (1.52u, NIH, Bethesda, MD, USA), following the approach outlined by Qi et al. (2020). Additionally, a compound microscope equipped with a digital camera (NLCD-120E; Shanghai SELON Scientific Instrument, Shanghai, China) was utilized to assess the shape, color, and quantity of MPs, as described by Nor & Obbard (2014). A covered plate sample was observed at a magnification of ×10, and the MPs were identified and measured according to their physical traits, following the methodology described by Nor & Obbard (2014). Throughout the examination, the count, size, shape, and color of MPs were documented.
The polymeric identification of the MP samples was performed using Fourier-transform infrared spectroscopy (FTIR: NICOLET IS20, Thermo Scientific, Waltham, MA, USA) in attenuated total reflection mode within the range of 500–4,000 cm–1. One hundred (100) MP particles, 50 from each station were taken for polymer type identification. The particles were compacted into pellets using manual pressure, and the analysis settings were adjusted to know the percentage transmittance (% T). The instrument’s parameters were fine-tuned based on the instructions provided by the manufacturer, and spectral analysis was carried out. The obtained spectral peaks were scrutinized and interpreted in conjunction with relevant literature to determine the composition of plastic polymers present in the water samples.
To ensure a clean laboratory environment and minimize the risk of background contamination, several precautions were implemented. Researchers wore cotton lab coats and gloves during the experimentation process. All solutions employed in the experiments underwent filtration using a 0.45-μm filter. Utmost care was taken to thoroughly rinse glassware, tools, and containers with deionized water, and they were diligently wrapped with aluminum foil after each of the steps to ensure their cleanliness was maintained. Furthermore, synthetic clothing, which could potentially introduce contaminants, was avoided during microscopic analysis. These measures aimed to maintain the integrity of the samples and minimize any potential sources of contamination.
Statistical analyses were performed using Microsoft Excel and IBM SPSS (version 26, IBM, Chicago, IL, USA). To assess if there were significant variations in MP abundance between the wet and dry seasons, a Mann-Whitney U test was employed, with values presented as the mean ± SD. The quantity and abundance of MPs were reported as the number of particles per square kilometer (MPs/ km2).
Results
Station-wise abundance (MPs/ km2) was found higher in Avoimitro Ghat (94,861 ± 57,126) compared to Kalurghat (31,343 ± 23,183) (Fig. 2). The mean abundance was found greater in the wet season (76,134 ± 49,641) than the dry season (63,101 ± 39,174). According to the Mann-Whitney U test, the abundance of MPs in the wet season (mean rank = 315.05) was significantly higher than in the dry season (mean rank = 261.95) (U = 33,824.50, z = –3.836, p = 0.0001). Among the months, the MPs abundance was highest in August while the lowest abundance was found in January (Fig. 3).
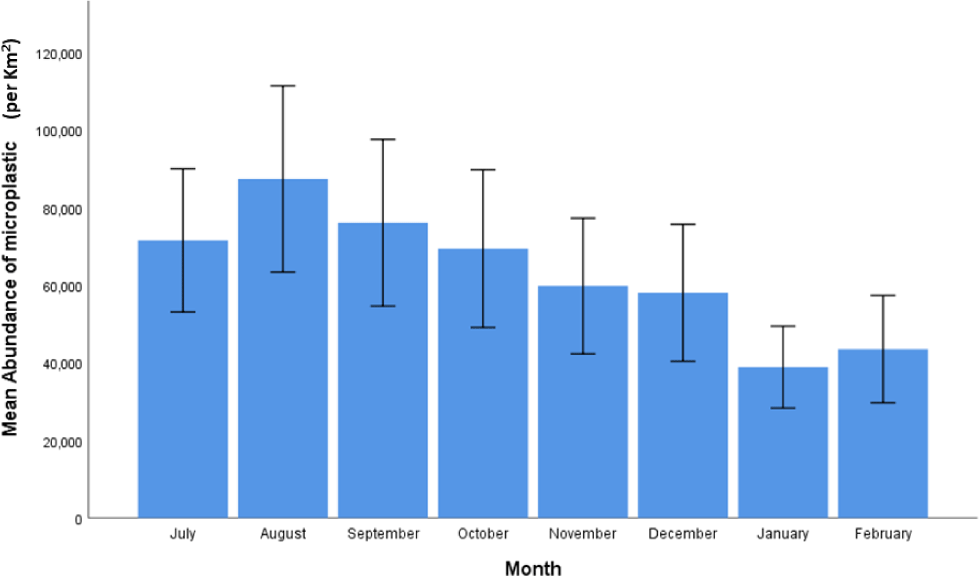
Six types of MPs, including fragments, filaments, films, foams, granules, and pellets were identified (Fig. 4) in the collected samples. Fragments (132,291 ± 89,051) were the most abundant type, while pellets (8,264 ± 5,037) were the least abundant type of MPs found in this study (Table 1).
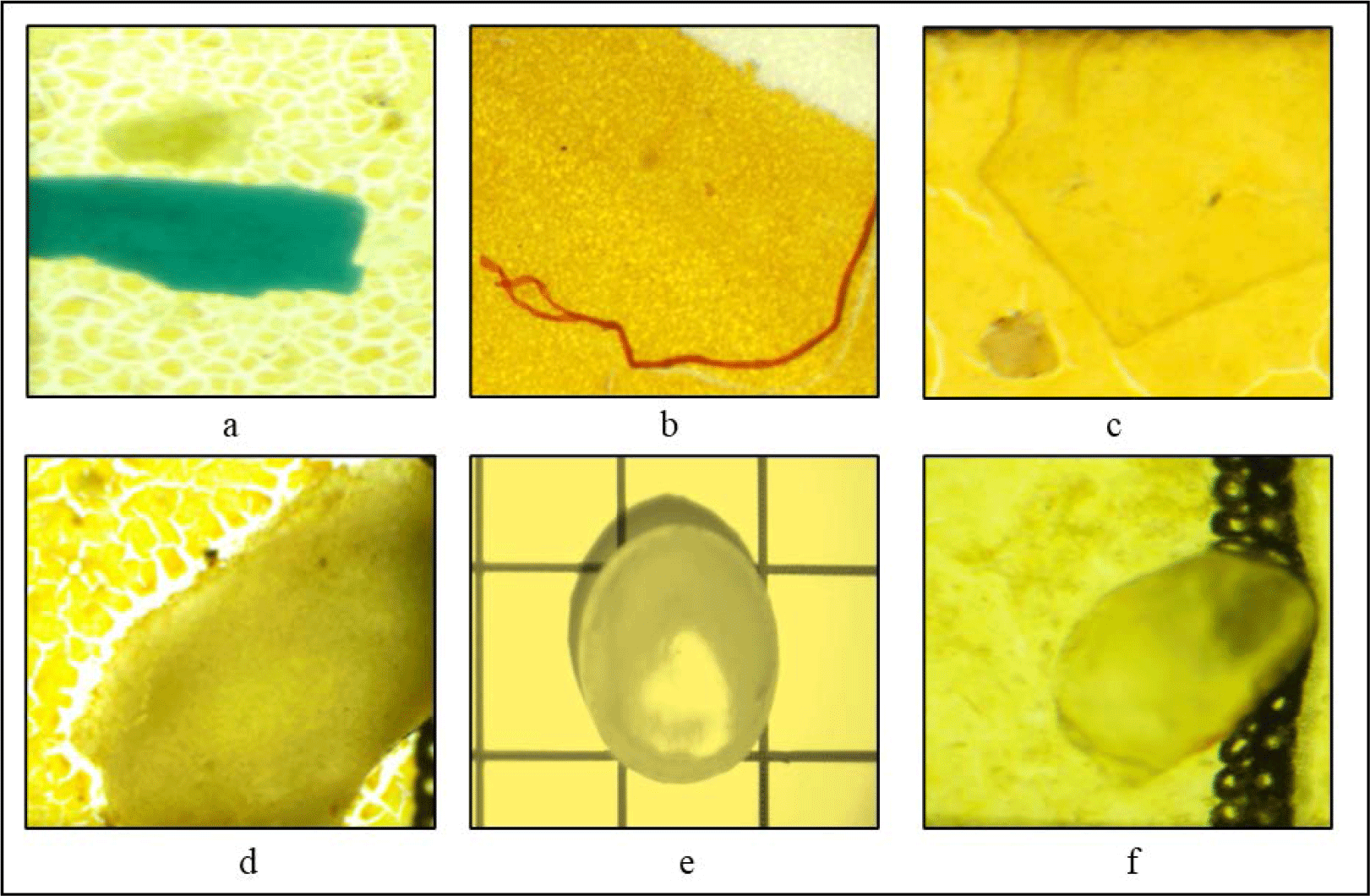
Type | Mean (MPs/ km2) |
---|---|
Fragment | 132,291 ± 89,051 |
Filament | 117,430 ± 95,028 |
Film | 69,444 ± 51,060 |
Foam | 38,611 ± 20,922 |
Granule | 12,569 ± 9,602 |
Pellet | 8,264 ± 5,037 |
Five different size categories of MPs were identified, ranging from 300 to < 500 µm, 500 µm to < 1 mm, 1 to < 2 mm, 2 to < 3 mm, and 3 to < 5 mm. The mean count of MPs varied for different size classes between the stations and seasons. Avoimitro Ghat had the highest abundance of size class 1 to < 2 mm, while Kalurghat had the highest abundance of 500 µm to < 1 mm sized MPs (Fig. 5). The MPs of the size class 1 to < 2 mm were the most common in both seasons. MPs of the size class 2 to < 3 mm were least abundant in the wet season while MPs of the size class 300 to <500 μm were least abundant in the dry season (Fig. 6).
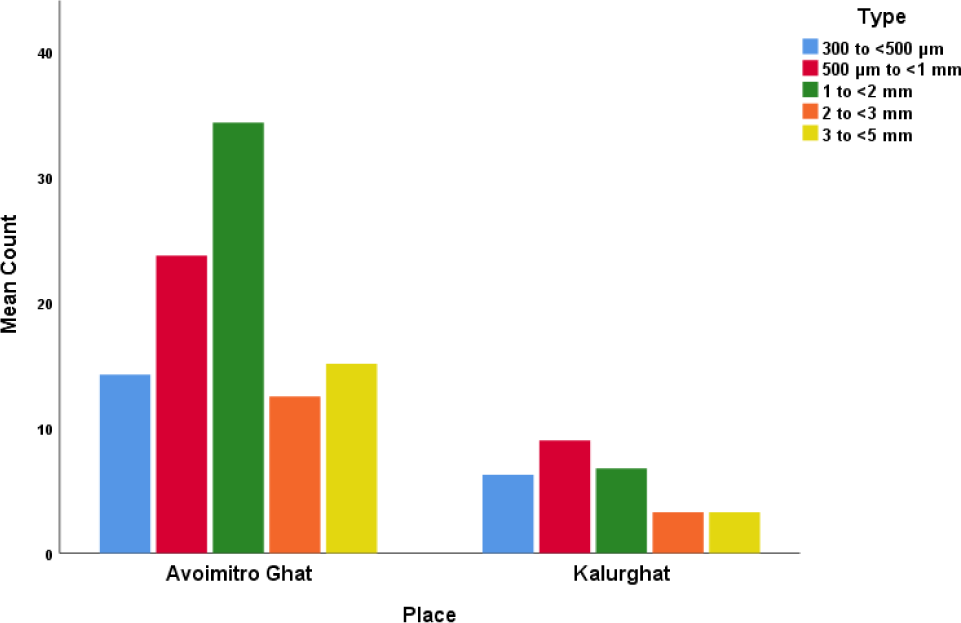
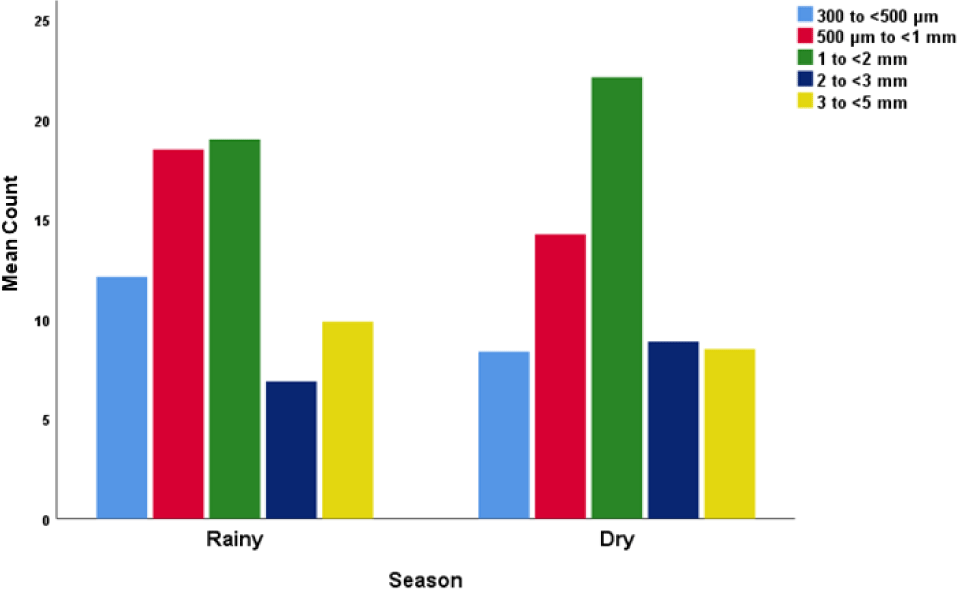
Ten different colored MPs were observed, including brown, red, black, white, green, transparent, pink, orange, blue, and yellow. Among them, the highest mean count of brown MPs was found in Kalurghat, while the Avoimitro Ghat had the highest count of blue MPs (Fig. 7).
Six (6) shapes of MPs were identified which were irregular, round, elongated, rectangular, cylindrical, and angular. The irregularly shaped MPs were highly abundant in Avoimitro Ghat while elongated-shaped MPs were high in Kalurghat. The highest mean counts of elongated-shaped MPs were found in both the wet and dry seasons. Cylindrical-shaped MPs had the lowest count in both seasons and both stations (Figs. 8 and 9).
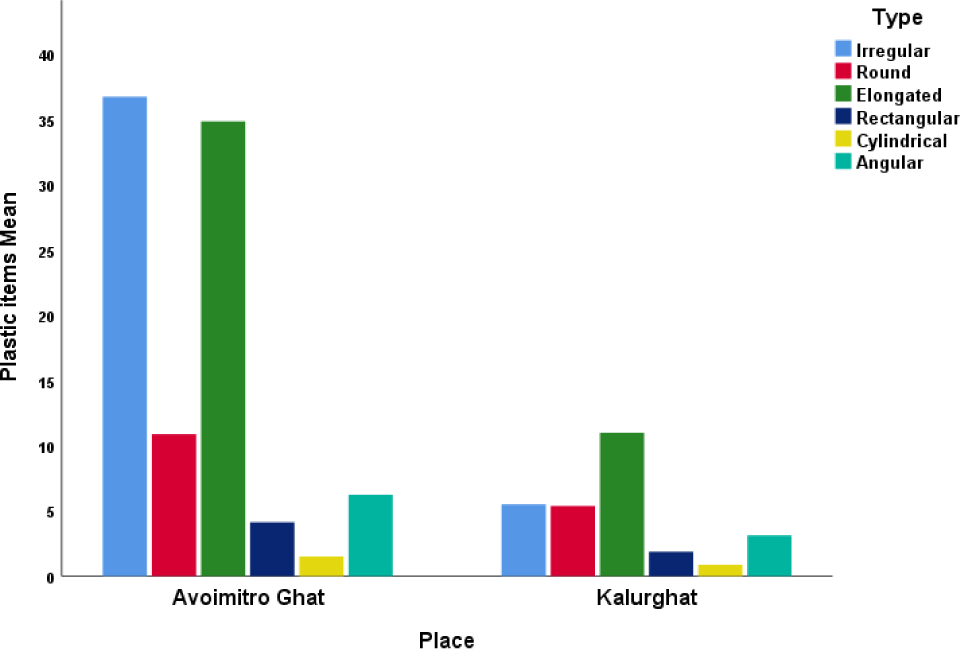
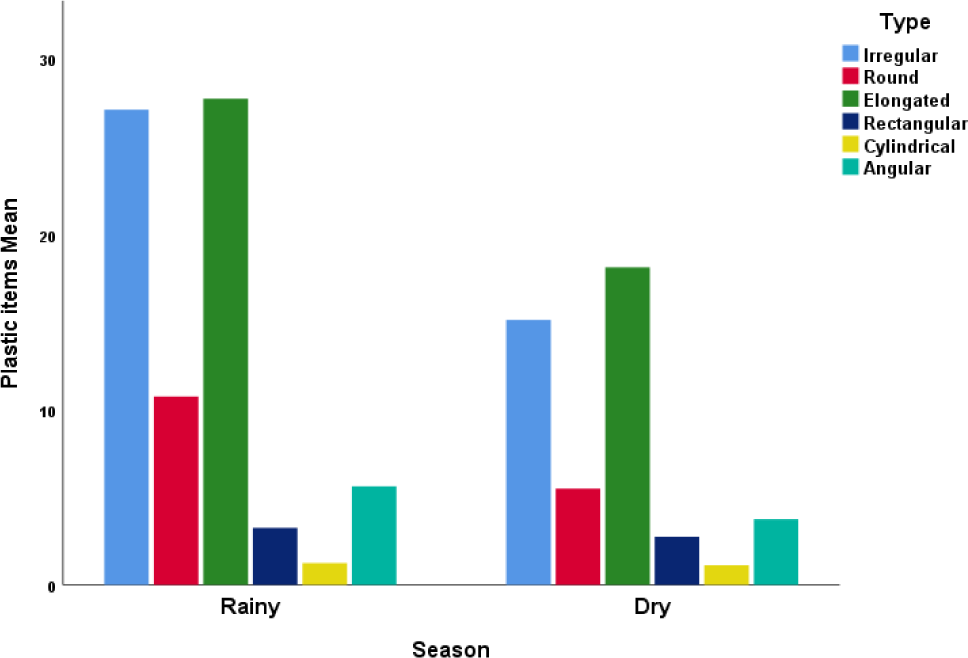
A total of 10 polymer compositions of MPs were found from FTIR analysis in the study area, with eight (8) types in each station (Table 2). Among the polymer compositions, polypropylene atactic was found in the highest proportion at both Avoimitro Ghat (32%) and Kalurghat (17%). On the other hand, polypropylene syndiotactic and alkyd resin were found in the lowest proportion at Avoimitro Ghat (4%) and Kalurghat (2%) respectively (Table 2).
Discussion
The Karnaphuli River is heavily contaminated with MPs due to its proximity to urban areas and industrial sectors (Fatema et al., 2023; Rakib et al., 2022). The presence of MPs in the river water poses a significant threat to aquatic organisms as they can ingest or absorb these particles, leading to physical harm, toxicity, and negative impacts on growth and reproductive capabilities (de Sá et al., 2018; Issac & Kandasubramanian, 2021). Furthermore, the accumulation of MPs in sediments can have long-term ecological and ecosystem consequences (de Sá et al., 2018; Mehra et al., 2020; Rakib et al., 2022). Consequently, it is crucial to investigate various aspects of MPs, including their abundance, type, shape, color, size, and polymer composition. The present study revealed notable variations in MP abundance (per Km²) across different locations, with Avoimitro Ghat (94,861 ± 57,126) exhibiting comparatively higher levels, while lower levels in Kalurghat (3,1343 ± 23,183). Rakib et al. (2022) reported MP quantities ranging from 22.29 to 59.5 items per kilogram (kg) based on dry weight in the sediment of the Karnaphuli River estuary. This investigation focused exclusively on MP abundance in sediment, whereas the present study examined MPs in surface water. Hossain et al. (2023) found a range of MP concentrations from 33.33 to 316.67 items/m³, with a mean value of 128.89 ± 67.94 items/m³ in the Meghna River estuary in Bangladesh. Islam et al. (2022) identified MP concentrations in surface water ranging from 4.33 ± 0.58 to 43.67 ± 0.58 items per liter in the Buriganga River, Bangladesh. Nawar et al. (2023) found an average of 2.66 × 10³ MP particles/liter in water samples from the Pasur River, Bangladesh. These findings differ from the present study regarding MP levels but share a common observation of a significant presence of MP in river environments. These findings highlight the widespread occurrence of MPs in the aquatic ecosystems of Bangladesh, complementing the observations made in the present study.
During the wet season, the mean abundance of MPs per km2 was higher (76,134 ± 49,641) in this study than in the dry season (63,101 ± 39,174). The finding is consistent with previous studies by Li et al. (2021), Rakib et al. (2022), Warrier et al. (2022), and Wei et al. (2022) who also found a higher abundance of MPs in the wet season. However, Malla-Pradhan et al. (2022) found a higher average count of MPs during the dry season (2.96 ± 1.83 particles/L) than in the wet season (1.51 ± 0.62 particles/L) in urban Lake Phewa, Nepal. Fatema et al. (2023) found a higher abundance of MPs in winter (152,222.22 items/km2), and early summer (164,444.44 items/km2) than in the wet season (120,111.11 items/km2). The elevated MP concentrations during the wet season can be attributed to heavy rainfall and increased runoff, which resuspends and transports previously deposited MPs within the river basin. Conversely, lower precipitation and reduced surface runoff during the dry season result in decreased MP concentrations (Eo et al., 2019). The current study identified six different types of MPs, with fragments being the most abundant type. In a study by (Islam et al., 2022), fragment-type MPs were predominant in the surface water (72.7%) and sediment (85.5%) respectively in the Buriganga River, Bangladesh. The finding of the present study also aligns with previous research conducted by Takarina et al. (2022) and Fatema et al. (2023). In contrast, the number of fibers and fragments remained rather low at the confluence of the Elbe and Mulde Rivers in Germany (Laermanns et al., 2021).
The prevalence of fragments and fibers in the surface water samples suggests that secondary MPs, resulting from the disintegration and fragmentation of larger plastics, are more common in the studied area. Filaments originate from laundry wastage and the remaining fishing net, while fragments were likely produced through the degradation of larger plastic items. The various types of MPs identified in the samples were found to originate from different sources, including both land-based and sea-based sources (Prabhu et al., 2022). Notably, the abundance of pellet-type MPs was found to be the lowest, indicating that primary MPs may not be a major contributor to the MPs in the studied area. This study provides valuable insights into the types and sources of MPs present in surface water samples.
In terms of MP size distribution, smaller particles, ranging from 1 to less than 2 mm, were the most frequently observed in this. Matjašič et al. (2023) similarly discovered MPs in the size range of 0 to 0.99 mm in two catchments in Slovenia. In the Pasur River of Bangladesh, Nawar et al. (2023) found the majority of MP particles measured less than 1 mm. Moreover, studies by Laermanns et al. (2021) documented MP size ranges of 0.5 mm to 1 mm and 0.05 mm to 0.1 mm at the Confluence of the Elbe and Mulde Rivers in Germany, respectively. The findings of the size range are in agreement with previous research conducted by Fatema et al. (2023), Hossain et al. (2022), and Wei et al. (2022), as well. The combination of strong water flow and abrasion resulting from tidal movements in river systems were identified as key factors contributing to the fragmentation of larger plastic pieces. This process ultimately leads to a higher prevalence of MPs. The elevated presence of these MPs in our study area implies an increased risk of aquatic organisms mistaking them for food. Additionally, this study revealed that brown and blue MPs were the dominant color types, aligning with the prevalence of blue-colored MPs found by Fatema et al. (2023) and differing from the dominant color types reported by Hossain et al. (2022) and Nawar et al. (2023), who found white and black, respectively.
Variations in the dominant color type of MPs observed in different studies can be attributed to differences in the sources of MPs and environmental conditions. In the present study, the most prevalent polymer composition found in both stations was polypropylene (PPA), which aligns with findings from other studies conducted in the Karnaphuli River (Fatema et al., 2023; Rakib et al., 2022) and globally (Takarina et al., 2022; Talbot et al., 2022). Fatema et al. (2023) identified polyethylene terephthalate as the most abundant polymer type, Rakib et al. (2022) reported PPA, polystyrene, polyethylene terephthalate, and cellulose, and Hossain et al. (2022) reported polyethylene and polyethylene terephthalate were the most abundant polymer in the surface water of the Karnaphuli River. These similarities indicate that various water bodies worldwide harbor similar types of polymer compositions, underscoring the urgent need for global initiatives to address plastic pollution. The study also revealed that irregular and elongated MPs were the most frequently observed shapes across seasons and stations, consistent with the findings of previous studies (Al Nahian et al., 2023; Hossain et al., 2021). Cylindrical-shaped MPs were the least common in this study. Factors such as the source of waste, breakdown of MPs, quality of debris, exposure to UV-B radiation, and wind drift may contribute to variations in the morphology of MPs (Prabhu et al., 2022).
Understanding the distribution and characteristics of different MP shapes is crucial, as they can influence the movement and buildup of MPs in the environment and their interactions with biota. Irregular and elongated MPs, due to their larger surface area and roughness, are more likely to accumulate in sediment or be ingested by aquatic organisms. Therefore, knowledge of MP shapes can provide valuable insights into their sources, transport pathways, and ecological impacts. However, future research should also investigate other sources of MPs, including industrial waste, household garbage, and shipping practices. Furthermore, there is a need for a comprehensive understanding of the impact of MPs pollution on the health of local populations and the aquatic ecosystem of the Karnaphuli River in Bangladesh. Conducting further research in this area is essential for gaining a comprehensive understanding of the issue and developing effective solutions.
Conclusion
This study focused on investigating the occurrence and impact of MP pollution in the Karnaphuli River in Bangladesh. The selection of this research site was based on its high level of urbanization, industrialization, ecological importance, and population density. The results revealed the presence of MPs in the sampled sites, indicating significant risks to aquatic organisms, the environment, and the overall ecosystem. These findings establish an important foundation for assessing the extent of MP pollution in the Karnaphuli River. The information obtained from this study carries substantial value for various stakeholders, including policymakers, scientists, ecologists, environmental activists, hydrologists, non-governmental organizations, and governments. It can inform their strategic planning and facilitate the implementation of conservation and management measures for the river. However, it should be emphasized that further research and continuous monitoring efforts are essential to comprehensively understand the long-term consequences of MP pollution and to develop sustainable solutions to address this urgent global issue.