Introduction
Fish are a crucial resource in human societies, and understanding their behavioral traits and seasonal habitats is important. However, they not only move horizontally but also vertically, making it quite challenging to track their movements. After the first fish-tracking study on salmons (Trefethen et al., 1956), various devices have been developed. We can now study various fish species with a wide range of devices. There are many kinds of devices for tracking the horizontal location of the fish (Brownscombe et al., 2019; Hussey et al., 2015; Lowerre-Barbieri et al., 2019) and also collecting swimming depth or environmental data around them (Block et al., 1998; Coffey & Holland 2015). We can now explore fish ecology questions more effectively using these diverse bio-logging devices.
Advanced bio-logging study is important for constructing fisheries management plans (Allen & Singh 2016; Crossin et al., 2017; Secor, 2015) and for reducing disease and stress of individuals in aquaculture (Mei et al., 2022). Fish tracking studies reveal the habitat selection and seasonal migration patterns of fish according to their developmental stages (Hazen et al., 2012). For instance, a study with an acoustic telemetry device provided movement data of various commercially important fish species, such as Atlantic cod (Gadus morhua; Cote et al., 1998) and winter flounder (Pseudopleuronectes americanus; DeCelles & Cadrin 2010). In the case of pelagic fish study, satellite mediated telemetry system provides a broad movement scale of large fish kinds of Bluefin tuna (Thunnus thynnus; Block et al., 1998) and greater amberjack (Seriola quinqueradiata; Tone et al., 2022). Furthermore, monitoring fish swimming behavior has the potential to enhance the quality of products in the aquaculture industry. Swimming data collected within an aquaculture environment could be a valuable indicator representing water quality or population densities (Alfonso et al., 2020; Martins et al., 2012).
Here, we aimed to review the previous bio-logging research and introduce the different types of bio-loggers for fish tracking. Using the bio-logging keywords, we estimated the number of publications on fish tracking studies. Then, we categorized the earlier studies into different groups depending on the data collecting and transmitting methods. We look forward to contributing our review and a case study report to future studies on the widespread application of fish bio-logging.
To estimate the publication changes in fish bio-logging, we browsed the Web of Science (https://www.webofknowledge.com) and searched for research articles containing the keywords. Because some researchers used “biologging” instead of “bio-logging”, we included both. In addition, we included the terms to indicate bio-logging devices (“biologger” and “bio-logger”). Because bio-logging in fish is often called “telemetry”, we include the terms (“animal telemetry” and “animal-borne telemetry”). In addition, more generally, we included “tagging” and “tracking”. To restrict our study in fish, we only allowed articles with “fish” or “shark” or “Actinopterygi” or “Chondrichthyes”). This yields 9,411 publications by 2022). Among the total 9,411 articles, 1,487 were using “Acoustic” and 892 were using “Satellite”. The results are presented in Fig. 1.
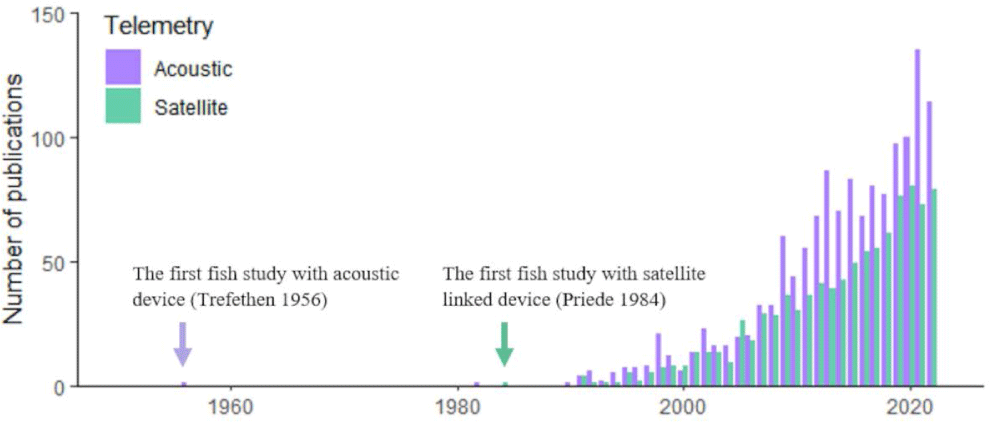
Since the first study with individual tagging on salmons (Trefethen, 1956), fish tracking has begun. Due to the technical limitations, however, only a few studies were reported. Then, Priede et al. (1984) tracked a basking shark (Cetorhinus maximus) by Advanced Research and Global Observation Satellite (ARGOS) satellite with a ultra-high-frequency (UHF) radio transmitter. This study proved that large aquatic animals can be monitored for a long-term period with satellite tracking. In the 21st century, the number of publications in both telemetry and satellite tracking has annually increased.
In fish bio-logging, we categorized the bio-loggers according to the sensor types for fish movement research (Table 1). The main research purpose of data is to locate the animals. Researchers used acoustic-and radio-transmitters. By inserting a small device into the body, it communicates with the stationary or moving receivers when passing at a close distance (Fig. 2A). Passive telemetry systems are based on scanning the coded information from the receivers. Although the underwater stationary communicating methods are still being commonly used and efficient, more direct methods at the water surface are communicating with satellite technologies. Since Block et al. (1998) applied pop-up satellite tags on Atlantic bluefin tuna (T. thynnus), many researchers have used this system for collecting long-term data logging by releasing the tags to the surface and communicating with the satellites (Fig. 2C). It provides data collected under the water and sends information to the ARGOS satellites. The released location can be determined from the ARGOS communication places (which are called, ‘Collect Localisation satellites (CLS)’ with ARGOS transmission). Still, it is not collecting the movement locations in water. The swimming locations before release should rely on other sensors, such as a sunlight sensor (which is often called a ‘geolocation system (GLS)’ with light intensity). To determine the accurate locations, one of the most reliable geolocation devices is Global Positioning System (GPS). Because GPS collects latitude and longitude data from multiple satellites (more than three) by estimating the distances from the receiver to each satellite. Thus, it enables researchers to use highly accurate (< 10 m) (see Hulbert & French 2001). Despite the high accuracy, GPS has not been applied to swimming fish due to the satellite communication under the water. For better communication with GPS satellites, buoy-based GPS telemetry was used in shallow swimmers (sunfish in Sims et al., 2009; New Zealand eagle ray in Riding et al., 2009) (Fig. 2B).
Sensor | Target data | Selected previous studies |
---|---|---|
Acoustic-transmitter | Location | ∙ The first acoustic tracking on chinook salmon (Oncorhynchus tshawytscha) (Trefethen, 1956) ∙ American eel (Anguilla rostrata) migration (Béguer-Pon et al., 2014) ∙ Migration study on Sockeye salmon (Oncorhynchus nerka) and steelhead (Oncorhynchus mykiss) (Furey et al., 2015) |
Collect localization satellites (CLS) with Argos transmission | Location | ∙ The first ARGOS satellite tracking on basking sharks (Cetorhinus maximus) (Priede, 1984) ∙ Migration study on whale sharks (Rhincodon typus) (Sleeman et al., 2010) |
Passive integrated transponders (PIT) | Location | ∙ The first PIT test study on chinook salmon (Oncorhynchus tshawytscha), and coho salmon (Oncorhynchus kisutch) (Prentice & Park 1983) ∙ Winter movement study on Atlantic salmon (Salmo salar) (Stickler et al., 2011) ∙ Winter survival study on brown trout (Salmo trutta) and European sculpin (Cottus gobio) (Weber et al., 2016) |
Radio-transmitter | Location | ∙ Sauger (Stizostedion canadense) (Pegg et al., 1997) ∙ Tracking study on escaping rainbow trout (O. mykiss) from aquafarm (Patterson & Blanchfield, 2013) |
Ambient water temperature | Ambient water temperature | ∙ Daily water temperature study on bluefin tuna (Thunnus thynnus) with the first pop-up (pop-off) satellite device (Block et al., 1998) ∙ Temperature selection study on lake charr (Salvelinus namaycush) (Jasonowicz et al., 2022) |
Geolocation System (GLS) with light intensity | Location | ∙ Basking shark (Southall et al., 2006) ∙ Whitetip shark (Carcharodon longimanus) (Howey et al., 2016) ∙ Greater amberjack (Seriola dumerili) (Tone et al., 2022) |
Body temperature | Body or visceral warming | ∙ Behavioral thermoregulation study on leopard shark (Triakis semifaciata) (Hight & Lowe, 2007) ∙ Feeding ecology study on bluefin tuna (Thunnus maccoyii) (Bestley et al., 2008) |
Global Positioning System (GPS) for high accuracy | Location | ∙ GPS-bouy on sunfish (Mola mola) (Sims et al., 2009) and New Zealand eagle ray (Riding et al., 2009) ∙ GPS-AUV receiver with an acoustic transmitter on chinook salmon (Oncorhynchus tshawytscha) (Eiler et al., 2019) ∙ Whale shark (Rhincodon typus) (Andrzejaczek et al., 2021) |
Accelerometer for movement estimation | Acceleration | ∙ Mating behavior study on Nurse shark (Ginglymostoma cirratum) (Whitney et al., 2010) ∙ Daily hunt strategy study on Blacktip reef shark (Carcharhinus melanopterus) (Papastamatiou et al., 2015) |
Time-depth recorder (TDR) | Swimming Depth | ∙ Greenland sharks (Somniosus microcephalus) (Fisk et al., 2012) ∙ Lake whitefish (Coregonus clupeaformis) (Bergstedt et al., 2016) |
Video-camera | Moving images | ∙ Tiger shark (Galeocerdo cuvier) (Heithaus et al., 2002) ∙ White shark (C. carcharias) (Semmens et al., 2019) |
Dissolved Oxygen (DO) | Oxygen density dissolved in water | ∙ First DO from bluntnose sixgill sharks (Hexanchus griseus) (Coffey & Holland 2015) ∙ PSAT-TDR-DO blue marlin (Makaira nigricans) and sailfish (Istiophorus platypterus) (Logan et al., 2022) |
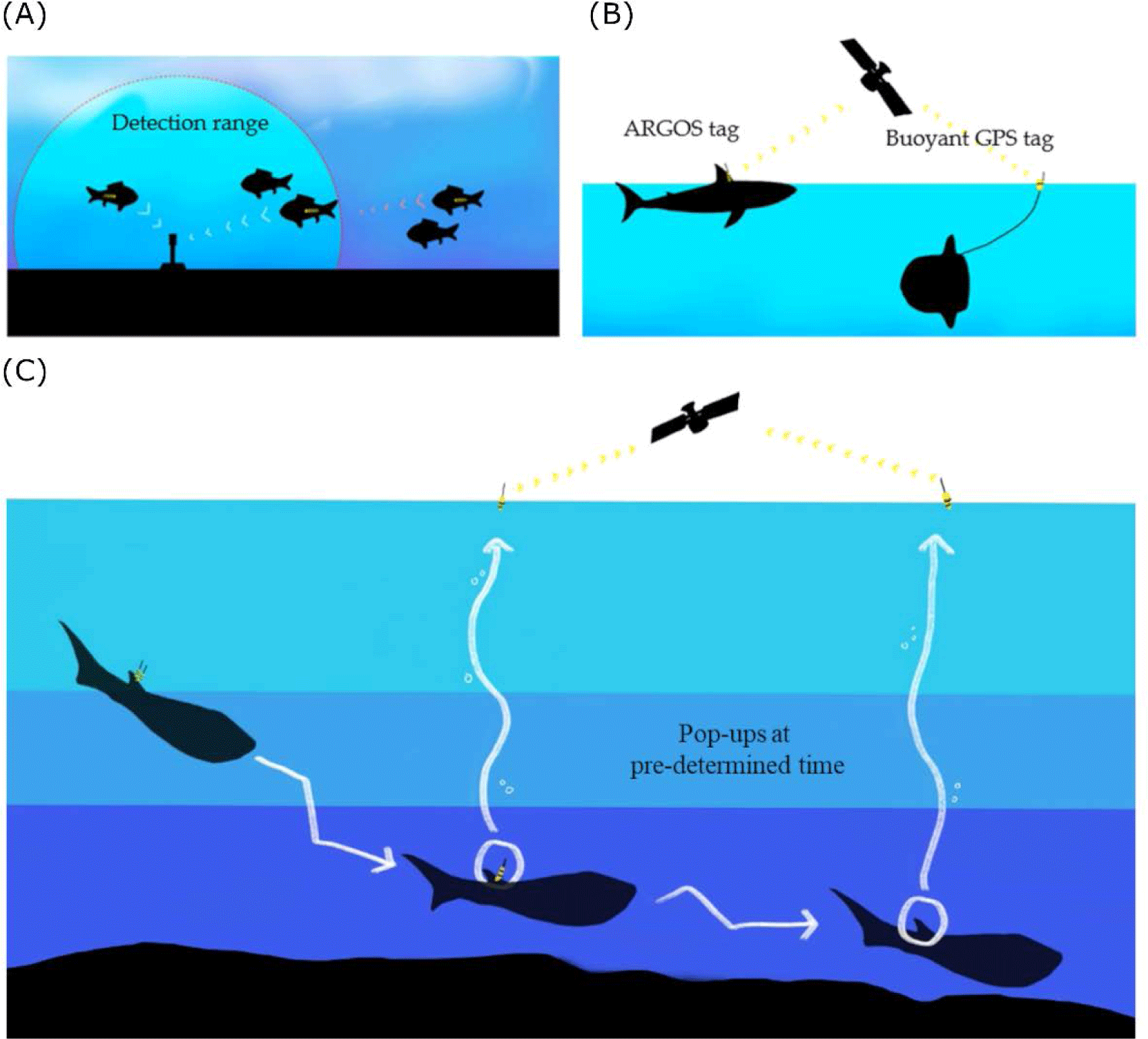
Together with locations, depth and temperature sensors are the main data for estimating the dive depths and ambient water environment. Since Kooyman (1966) used time-depth recorders on marine mammals (Weddell Seal, Leptonychotes weddelli), it has been miniaturized and applied to fish as well for vertical movement in time series. For 3D movement, accelerometers were used to estimate the detailed movement and energy expenditure. Acceleration has been used to examine the Blacktip reef shark (Carcharhinus melanopterus) by counting the bite rates for studying their daily hunting behavior (Papastamatiou et al., 2015).
Fish inhabit a variety of habitats and exhibit different ecological traits depending on their species. We have summarized previous studies based on the habitat and behavior of fish species through the following classification and schematic figure (Fig. 3).
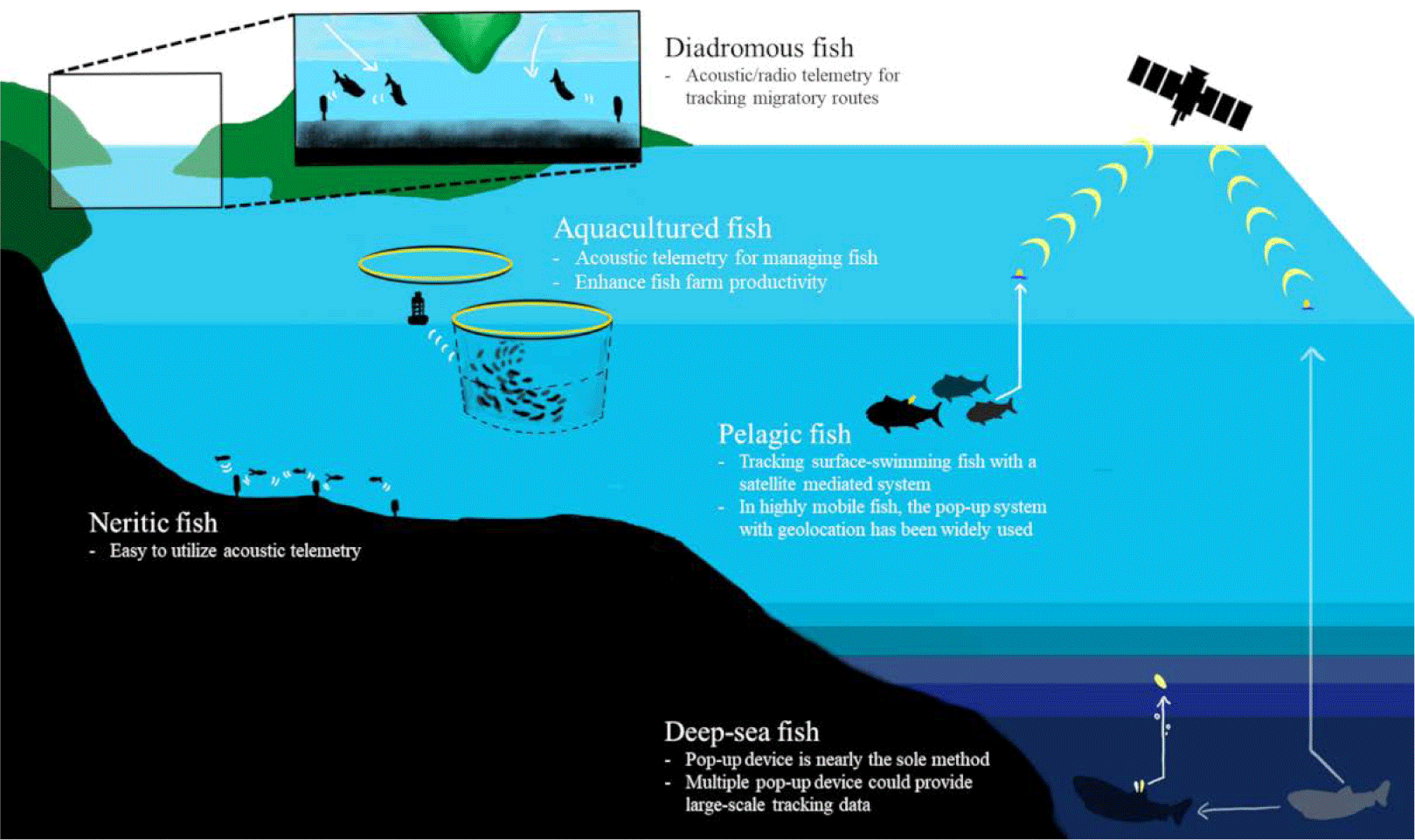
The dependence of acoustic telemetry on fixed receivers that detect transmissions over small distances (< 1,000 m) has focused the majority of research on coastal, estuarine, and freshwater ecosystems (Hussey et al., 2015). Although detection ranges decrease with noise (Pincock & Johnston 2012) and water stratification (How & de Lestang, 2012), it has advantages for fine-scale estimates of space use. In freshwater, the cover ranges are relatively smaller and shallower, compared to other ocean studies, radio-transmission has been the most used technique but passive integrated transponder (PIT) technology and acoustic telemetry have been more commonly used recently (Cooke et al., 2013).
Pelagic sharks have been tracked with ARGOS satellite trackers. When sharks swim near the surface, the bio-loggers on the dorsal fin or darting on the body could directly communicate with the satellites (Hammerschlag et al., 2011). One of the commercial satellite tags is the Wildlife Computers SPOT products (https://wildlifecomputers.com/our-tags/spot-argos-satellite-tags/). Location accuracy is up to 250 meters and the pressure sensor covers 2,000 meters depth.
In highly mobile pelagic fish, such as tuna and billfish, light sensor-based geolocation with pop-up archival tags (PAT tags) has been widely used (Hammerschlag et al., 2011). Such PAT tags are installed to track the long-term and large-scale movement. When animals do not perform enough surface diving for satellites, this pop-up can help researchers collect logged data. The light data for determining sunrise and sunset time provides geolocation, and the pressure and ambient temperature under the water provide depth and water temperature data by sending the signals to the ARGOS satellite after being released from the animals. Equipped with other sensors, such as accelerometers and oxygen sensors, can also contribute to the behavioral and environmental dataset of the PAT tags.
Juvenile salmons hatch in freshwater migrate to the ocean for growth, and turn back to their natal sites for breeding (Furey et al., 2015). They are often called, ‘Anadromous fish’ and acoustic transmitters have been applied to track their migratory routes (Matley et al., 2022). Researchers inserted tiny acoustic- or radio- transmitters to the juveniles born in fresh water and installed acoustic transmitter-receivers along the candidate migratory routes to follow their expected tracks and examine the survival rates (Cooke et al., 2005; Furey et al., 2015; Thorstad et al., 2013). This technique was also utilized to study the oceanic migration routes of American eels (Béguer-Pon et al., 2014), which are categorized as ‘Catadromous fish’.
If fish live in deep-sea with little sunlight, it is not available to use the light sensor for locating. For instance, Greenland sharks dive as deep as 1,560 m in cold deep water (Fisk et al., 2012). Thus, the current light-based geolocation is not easily applicable to this species. Hussey et al. (2018) deployed multiple pop-up tags that had been sequentially released per individual and estimated their locations from the released water surface with the depth and temperature data. Because such horizontal tracks are complex to follow, researchers have focused on their vertical movement with depth and temperature sensors as well as other environmental sensors like dissolved oxygen (DO; Bluntnose sixgill shark in Coffey & Holland 2015; Coffey et al., 2020).
Fish aquaculture systems can induce stress and health problems in fish (Broom, 2007), which can result in decreased productivity of aquaculture. High population density or poor water quality within fish farms can affect the swimming behavior of cultured fish (Sneddon & Wolfenden, 2016). Acoustic telemetry is an effective method for observing fish movement within a sea-cage production system. There were several studies with acoustic telemetry in the aquaculture industry (Føre et al., 2011; Kolarevic et al., 2016) and it could provide a better strategy for fish farming. For instance, an acoustic telemetry study of Atlantic cod (G. morhua) exhibits higher swimming activity during daytime compared with swimming activity during nighttime (Rillahan et al., 2011). Furthermore, extending the day photoperiod using artificial lights has been found to increase the swimming activity of fish. Observing the movement of fish within fish farming provides not only an understanding of their ecology but also assists in managing the welfare of fish and enhancing production levels (Crossin et al., 2017; Føre et al., 2011, 2018).
Domestic fish bio-logging studies could provide insights into the ecology of fish and serve as effective methods for fisheries resource management. Acoustic telemetry, suitable for studying fish behavior within its detection range, has been consistently employed in various studies (reviewed in Choi et al., 2022), including marine fish movement studies related to artificial structures (Shin et al., 2004) and comparisons between the movement of wild and cultured fish (Kang et al., 2008; Shin et al., 2005). Furthermore, recent studies have been conducted on migration of Mottled skate (Beringraja pulchra) (Im & Jo, 2015), Pacific cod (Gadus macrocephalus) (Lee et al., 2015), and yellowtail (S. quinqueradiata) (Kim et al., 2021) using pop-up satellite archival tag devices. Fish bio-logging can identify the impacts on fish caused by domestic anthropogenic disturbances. Although there is a study indicating that noise from marine construction affects the swimming behavior of marine fish (Heo et al., 2019), there is still a significant gap in research on the potential impacts of various marine development projects on fish. Recently in South Korea, large-scale marine facility constructions such as offshore wind farms have been underway, and these could have an impact on the marine ecosystem (Mooney et al., 2020). Therefore, we need active domestic research on the interaction between marine development and the marine ecosystem (Choi et al., 2022).
Although acoustic telemetry has been widely used since the 1950s and is still one of the most popular methods to track fish movement, it is limited to a short range from receivers. To increase the data recovery rates, researchers share their underwater receivers (Reubens et al., 2021; Young et al., 2020). Also, the collected information is strongly encouraged to share for collaboration among researchers, such as the Ocean Tracking Network, Integrated Marine Observing System in Australia, Integrated Tracking of Aquatic Animals in the Gulf of Mexico, and the Acoustic Tracking Array Platform in South Africa (Abecasis et al., 2018; Cooke et al., 2012). Thus, we expect that an active international platform for receiver sharing and data networks would increase the chances for collaborative studies across the continents and oceans.
Since GPS can only transmit the signal to the satellite at the surface of water, it can only be used on surface swimming sharks, rays, or large pelagic fishes. Thus, the geolocation method, which calculates the location based on sunrise and sunset times, is widely used for estimating location despite its low accuracy. Acoustic telemetry could be a more accurate method, but generally, this method is used for studying neritic species because of its short detection range. That is why we need a new tracking technology. The RAFOS Ocean Acoustic Monitoring (ROAM) tag, currently in the testing phase, is a long-range fish-tracking method with acoustic signals (Braun et al., 2019). In contrast to typical acoustic telemetry, the ROAM tag system utilizes low-frequency sound which is more appropriate for long-range communication than high-frequency sound. According to a field test conducted at the Mississippi River Delta (Rossby et al., 2017), the tag detected a low-frequency signal 60 km away from the sound source and the estimated accuracy of location tracking ranged from 70 to 560 m. This developing underwater positioning technology could provide a more accurate and long-range tracking method for studying fish.
Despite the clear benefits and advantages of bio-logging for fish studies, the potential impacts of the tagged individuals have been underestimated (reviewed in Klinard & Matley 2020). For a long-term study, researchers should consider the impacts of the bio-loggers and mortalities of the tracking results. Light weight sensors could provide better options. A newly tested tiny acoustic transmitter injection showed that it highly increased fish survival rates compared to surgically implanted acoustic transmitters (Deng et al., 2017). Non-invasive tagging system, with no injection, such as a skin-type surface bandage, would help fish individuals have less impact (Nassar et al., 2018). Furthermore, the miniaturization of sensors will provide more chances for researchers to conduct a broader range of studies. Current bio-loggers are mainly used for large animals that can tolerate the weight and dragging forces of the devices. For example, fluorometers (Lander et al., 2015) and DO sensors (Coffey & Holland 2015; Logan et al., 2022) are being used for sharks or large fish. We look forward to making the loggers miniaturized to apply the loggers even for small fish and multiple devices simultaneously.