Introduction
The trend of declining fish stocks remains the main challenge in today’s global fisheries (Pauly & Zeller, 2016). Various studies suggest that effective fisheries management could help reverse the trend of declining stocks but requires essential supporting preconditions, such as management capacities, policies, and fisheries data. Sufficient fisheries data for stock assessment is the basis in designing effective management strategies (Hilborn et al., 2020). Nevertheless, costs and limited technical capacities are two main challenges for obtaining ‘sufficient’ data in most fisheries (Ault et al., 2019). As one of the options to address this problem, Prince & Hordyk (2019) suggested the implementation of size selectivity in fishing as an initial fisheries management measure to reverse declining stocks. Managing size selectivity means that fisheries should be responsible for controlling the catch above the size or age of fish’ maturity (Prince & Hordyk, 2019), although the implementation remains a challenge without studies on the age and capacity in catch control.
Studies on size selectivity in fisheries aim to estimate the length or age at first capture in particular targeting species and represent combined factors affecting fish vulnerability as part of the stock assessment (Maunder et al., 2014). Study in fishery-specific selectivity includes two model functions, contact selectivity and availability. The former is the probability of a fish being captured when encountering fishing gear, while the latter shows the probability of fish being present or encountering fishing gear due to their spatial and temporal movement or distributions (Maunder et al., 2014). Fishing gear selectivity could be estimated and generally assumed as a function of the fish’s length or size; hence, the length composition of the catch could be used to approximate the age structure of the catch (Piner et al., 2018).
In fisheries management, the application of size selectivity to control catch size could be a simple approach to achieve stock and yield sustainability objectives in a data-poor fishery. Size selectivity is determined based on a certain reference point (e.g., spawning potential ratio or SPR) according to the context of the stock being managed, for example, by increasing the minimum catch length above the length at maturity (Prince & Hordyk, 2019). The size-selectivity approach could also serve as an initial fisheries management measure to prevent stock decline (Prince & Hordyk, 2019). For instance, hook size regulations for fisheries have been applied for freshwater fishing in the US Fisheries Order of Michigan State No. 202.19 of 2018 on Special Hook Size Fishing Regulations. In the Persian Gulf, hook size regulations were proposed for recreational marine fisheries in the area, since fishing with larger hooks and larger bait affected the exploitation pattern of the targeted species (Herrmann et al., 2018). Moreover, the prospect of using hook sizes as a tool for input control has been studied by the Western and Central Pacific Fisheries Commission (WCPFC) in the Scientific Committee Regular Session in 2018 (Gilman et al., 2018).
Groupers (Serranidae: Epinephelinae) play an essential role in coastal community fisheries in tropical and sub-tropical countries, providing a source of protein and livelihoods, particularly for small-scale fisheries (Frisch et al., 2016; Halim et al., 2019; Sadovy de Mitcheson et al., 2013). Global grouper production from capture fisheries has continuously increased in the last ten years, where the 2017 total production (443 thousand tons) almost doubled the production of the previous decade in 2007 (226 thousand tons) (FAO, 2019). Indonesia, one of the world’s top fish-producing countries, plays a key role in global grouper production, contributing 25% to global grouper production (FAO, 2019).
Groupers have unique biological and reproductive characteristics, where the fish can attain 40 years of age, have late sexual maturation (i.e., between 5 to 10 years), and spawn in specific aggregation sites (Sadovy de Mitcheson et al., 2013). Groupers also have a complex sexual mechanism, where most of the fish are protogynous hermaphrodites (i.e., being females during the early life stages and change the sex to males; Avise & Mank, 2009). These biological characteristics could make groupers vulnerable to fishing pressure and over-exploitation. For example, groupers’ slow growth and late maturity might not give enough time for the fish to reproduce and compensate for high fishing pressure (Sadovy de Mitcheson et al., 2013).
In the Saleh bay, West Nusa Tenggara province, Indonesia, groupers have been the primary target of the small-scale fisheries using various fishing methods and gear, including hooks and lines, nets, spearfishing, and traps (DKP NTB, 2018; Farmer & Ault, 2011; Retnoningtyas et al., 2021). The Saleh bay plays a significant role for local people, providing important fisheries resources for approximately 5,800 fishers in the area (Yulianto et al., 2016). The Saleh bay comprises 26 coastal villages inhabited by ~67,000 people, where the grouper production can reach around 4,800 tons annually (Asrial et al., 2018), comprised of around 50 groupers’ species (DKP NTB, 2018). To improve stock condition of the targeted grouper and snapper species in Saleh bay, Fisheries Management Plan of Action (FMPoA) was initiated in 2017 and was established in early 2018, which is the first provincial-level FMPoA in Indonesia (Retnoningtyas et al., 2021). Since the FMPoA establishment, various management strategies have been implemented to achieve the objectives, including to limit the catch size (length or weight of the fish caught) (DKP NTB, 2018; Retnoningtyas et al., 2021). In this context, understanding the selectivity of fishing gear is essential to inform management to ensure sustainable grouper fishery in the area. This study aims to examine the impact of different hook sizes on the length at first capture (Lc) using the two targeted grouper species (Plectropomus leopardus and Plectropomus maculatus) in Saleh bay, West Nusa Tenggara province, Indonesia.
Materials and Methods
Within the Saleh bay (Fig. 1), the fishers use spear gun, boat liftnet, hand line, troll line, and longlines to catch the grouper and snapper. Dominant fishing gear used (based on the fishing activity) were speargun (36%), longline (34%), and handlines (15%). Longlines and handlines use small hook size (number 7, gap size = 15 cm, see Fig. 2). In one of the villages within the Saleh bay, namely Labuhan Kuris village, the catch size (length) limit approach was implemented by changing the hook sizes. In response to the implementation a fisher group (called Sunu Merah) in the village voluntarily changed their fishing hooks to a larger size in 2017. The fishers changed their hook size from a smaller size (number 7, gap size = 15 cm) to a larger size (number 4, gap size = 20 cm; Fig. 2). The fishers also used modified troll lines by attaching a sinker made of fibre/plastic to the hook. This modification allows the troll line commonly used for pelagic fish to target demersal fish (e.g., grouper and snapper).
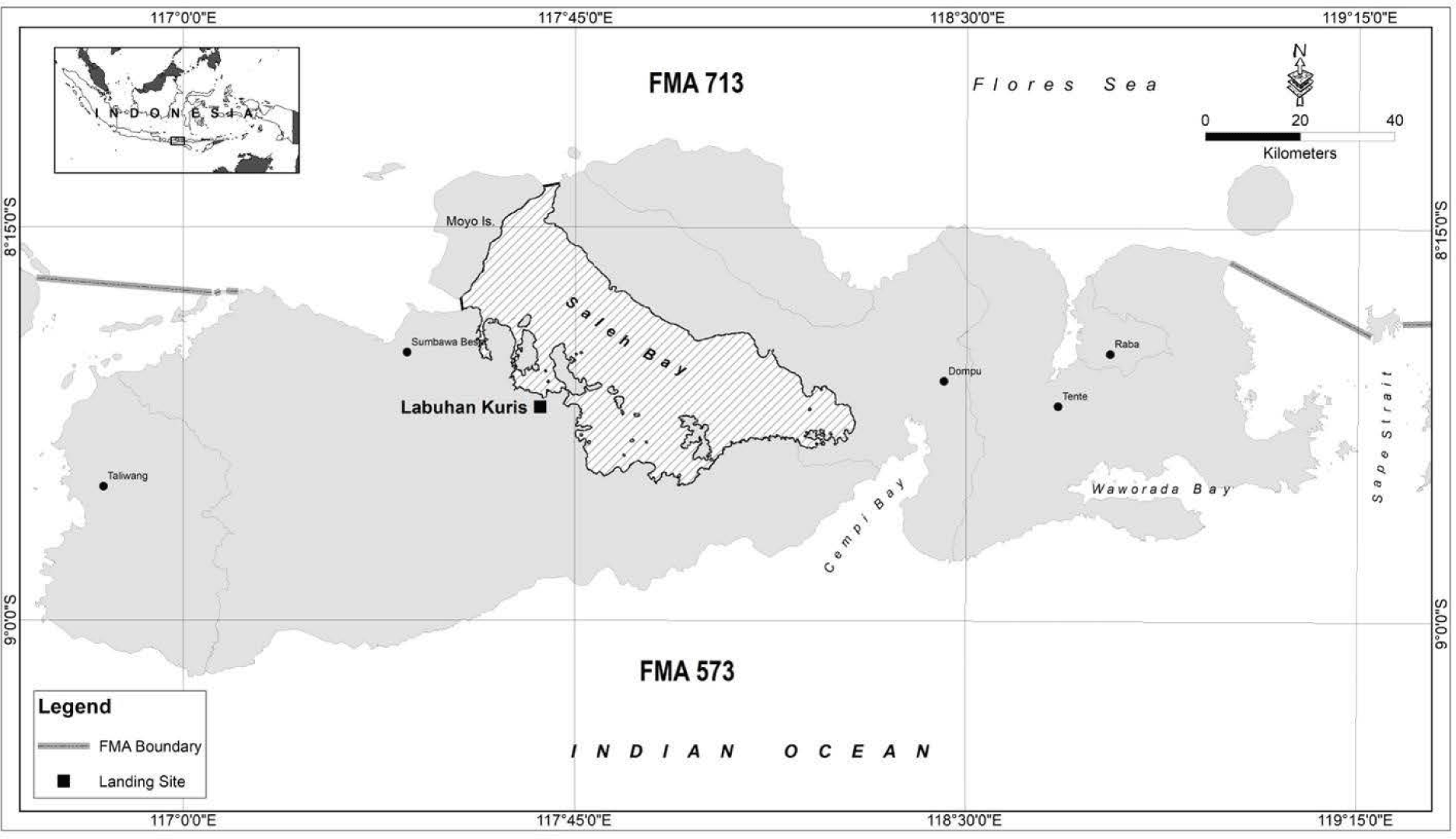
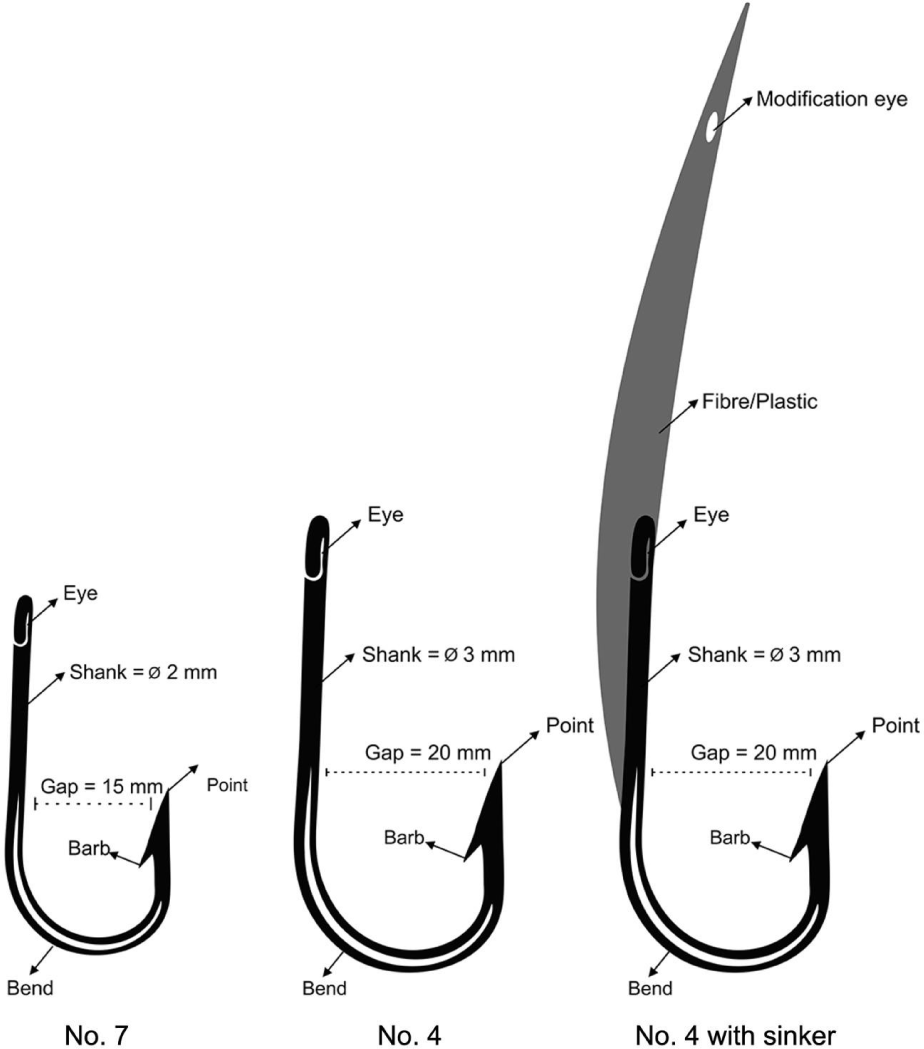
A trained enumerator collected the landed fish in the Labuhan Kuris Village from April 2016 to December 2018 for 10–15 days per month (Table 1). The enumerator recorded the number, species, and total length of each species landed, as well as recorded information about the fishing trip. A measurement board and a camera were used to measure the fish length and for further species identification. Fish catch data and trip information was recorded onto a tablet computer, equipped with a custom-made Android application. All collected data were then verified by a database manager who worked in parallel with a reef fish biologist to ensure correct species identification and size measurement.
During the study period, we recorded a total of 142 troll-line fishing trips that landed a total of 348 fish species. Of the fish collected, two species of P. leopardus and P. maculatus were the most dominant fish landed. Accordingly, further analysis of the selectivity was only for these species.
All the data recorded were grouped into two hook size numbers: 1) the landed fish that caught using the smaller hook size (hook size number 7) from April 2016 to September 2017, and 2) the landed fish that caught using the larger hook size (hook size number 4) from October 2017 to December 2018. For P. leopardus, 57 and 39 individuals were used for group analysis of the hook sizes number 7 and 4, respectively. While for P. maculatus, 125 and 45 individuals were used for group analysis of the hook sizes number 7 and 4, respectively. Catch composition was then described for two different hook size numbers and later the selectivity at size for two dominant species was estimated according to the two methods developed by Hoshino et al. (2020) and Millar & Holst (1997).
In the first analysis, the gillnet/bell-shaped selectivity method was used, applying four models to estimate the best selectivity curve, i.e., normal fix, normal scale, gamma and lognormal (Millar & Holst, 1997). Below are the four formulae used for the analysis:
-
1) The bell-shaped – normal fix selectivity function
-
2) The bell-shaped – normal scale selectivity function
-
3) The bell-shaped – gamma selectivity function
-
4) The bell-shaped – lognormal selectivity function
where L is length of fish caught by hook size mj, σ is standard deviation of length distribution, α and k is selection parameter need to be estimated, and µ is location parameter (Millar & Holst, 1997). The sizes of the hook used in the model were 15 cm for hook number 7 and 20 cm for hook number 4 (see Fig. 2). Length-frequency data were used for all models, and the best model according to the lowest deviance value was then selected.
In the second analysis, growth and natural mortality parameters of the targeted fish (Table 2) were used to estimate selectivity at size due to the ratio of M/K (natural mortality over growth rate) significantly influences the selectivity curve (Hoshino et al., 2020). Two types of selectivity were then selected: asymptotic selectivity and bell-shaped selectivity; to understand the impact of different hook sizes on both types. The selection was used following the likelihood ratio test where the null hypothesis (H0 = η0) is the asymptotic selectivity, and the alternative hypothesis (H1 = η1) is bell-shaped selectivity. The decision to reject the null hypothesis was then made according to the cumulative distribution at the test statistic 2 × (η1 – η0) (see Hoshino et al., 2020). Below are the two formulae used for the analysis:
No | Parameter | P. leopardus | P. maculatus |
---|---|---|---|
1 | Growth coefficient (k) | 0.12 | 0.10 |
2 | Asymptotic length (L∞) | 71.94 | 76.55 |
3 | Natural mortality over growth ratio (M/k) | 1.33 | 1.60 |
Adapted from DKP NTB (2018) with permission of author.
where l50 and l95 are the length classes at which 50% and 95% of maximum selectivity (assumed to be 1 henceforth) are reached, respectively. Lmax is the length class at which maximum selectivity is reached, and φ and Ψ control the rate of increase up to the maximum and the rate of decrease after the maximum, respectively (Hoshino et al., 2020).
All data analyses were conducted using R software (R Core Team, 2021). The gillnet function package provided by Millar (2009) was used to perform the first analysis following (Millar & Holst, 1997), while the R script provided by Hoshino et al. (2020) was used to perform the second analysis following Hoshino et al. (2020). In addition, the Kolmogorov-Smirnov test was used to determine the difference of length-frequency data between the hook sizes.
Results
The mean lengths of landed P. leopardus were 47.06 cm (range = 33.3–67.0 cm) and 47.56 cm (range = 31.6–60.9 cm) when the fishers use the hook size numbers 7 and 4, respectively (Fig. 3). While for P. maculatus, the mean lengths of the landed individuals were 50.92 cm (range = 31.2–68.8 cm) and 54.91 cm (range = 40.9–66.6 cm) when the fishers use the hook size numbers 7 and 4, respectively (Fig. 3). No difference was found between the hook sizes for length frequency of P. leopardus (KS test; D = 0.17004, p-value = 0.5148), while for P. maculatus, a significant difference was found between the hook sizes (KS test; D = 0.27667, p-value < 0.01).
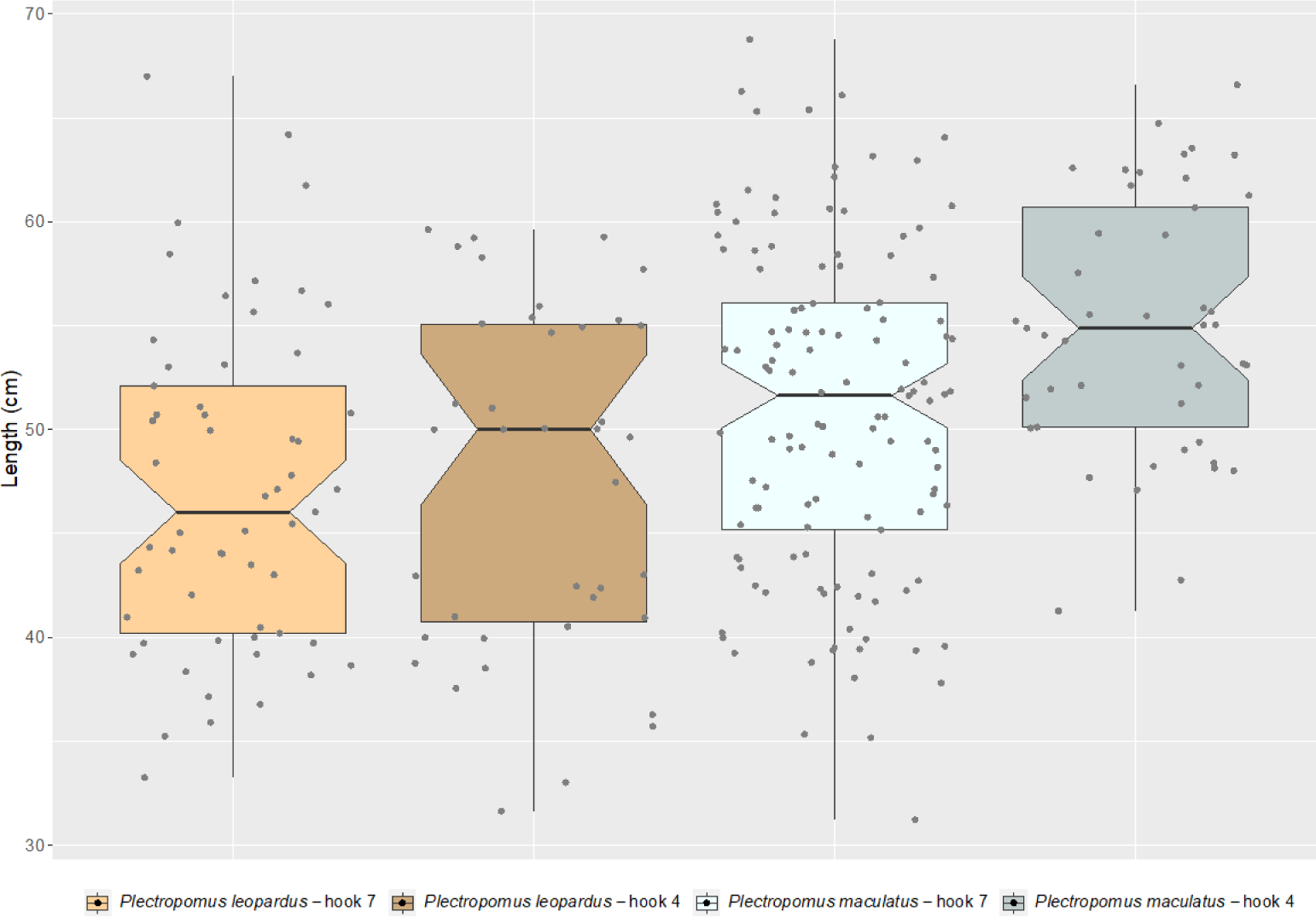
Based on the models introduced by Millar & Holst (1997), the best-fit models for P. leopardus and P. maculatus are normal scale and lognormal, respectively (Table 3). The selectivity curve and deviance residuals from the best-fit models are presented in Figs. 4 and 5 for both species. The maximum selectivity of P. leopardus reached 65 cm (hook number 7) and 87 cm (hook number 4; Fig. 4), while for P. maculatus, the maximum selectivity reached 60 cm (hook number 7) and 79 cm (hook number 4; Fig. 5).
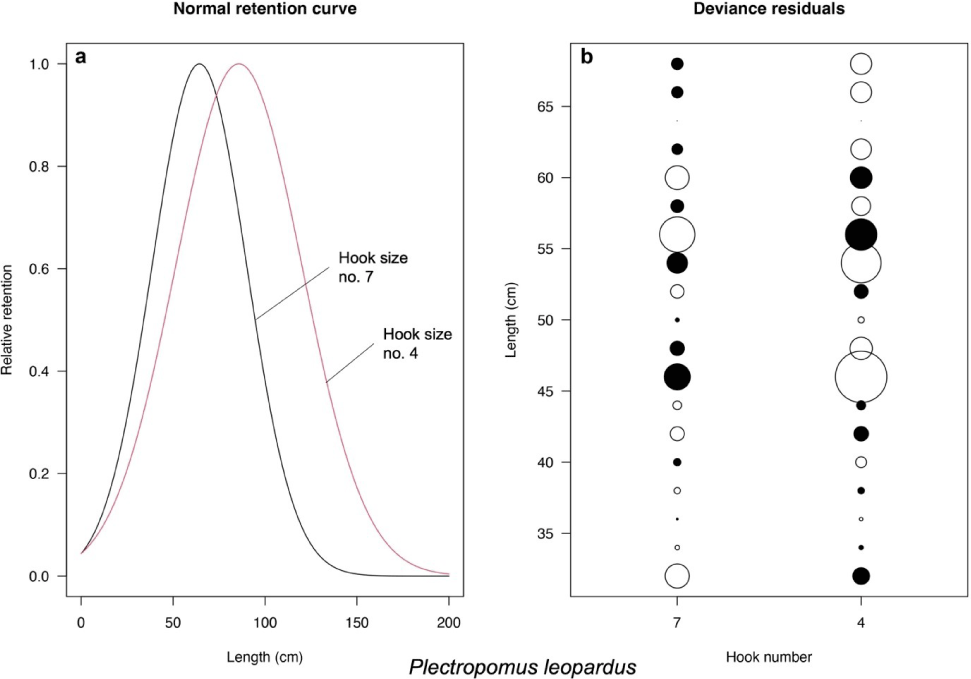
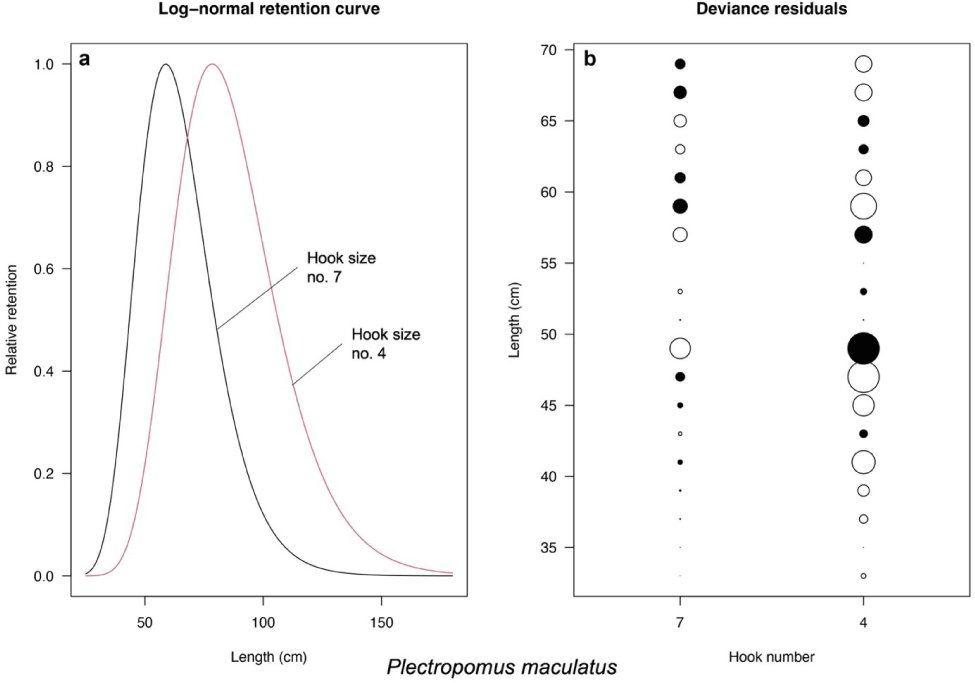
According to the likelihood ratio test of the model introduced by Hoshino et al. (2020), P. leopardus length fitted the asymptotic selectivity when the fishers use the hook size number 7 and fitted the bell-shaped selectivity when the fishers use the hook size number 4 (Fig. 6). The asymptotic selectivity analysis of P. leopardus resulted in the Lc (L50) of 45.28 and 51.77 cm and the L95 of 52.19 and 63.68 cm when the fishers use the hook size numbers 7 and 4, respectively (Fig. 6A). The bell-shaped selectivity analysis of P. leopardus resulted in Lmax of 56.01 cm (hook size number 7) and 63.00 cm (hook size number 4; Fig. 6B). The bell-shaped retention widths RW50 of P. leopardus were 19.34 cm (hook number 7) and 11.18 cm (hook number 4), resulting in a thinner shape of the curve, indicating a higher selectivity when the fishers use the larger hook size (hook number 4; Fig. 6B).
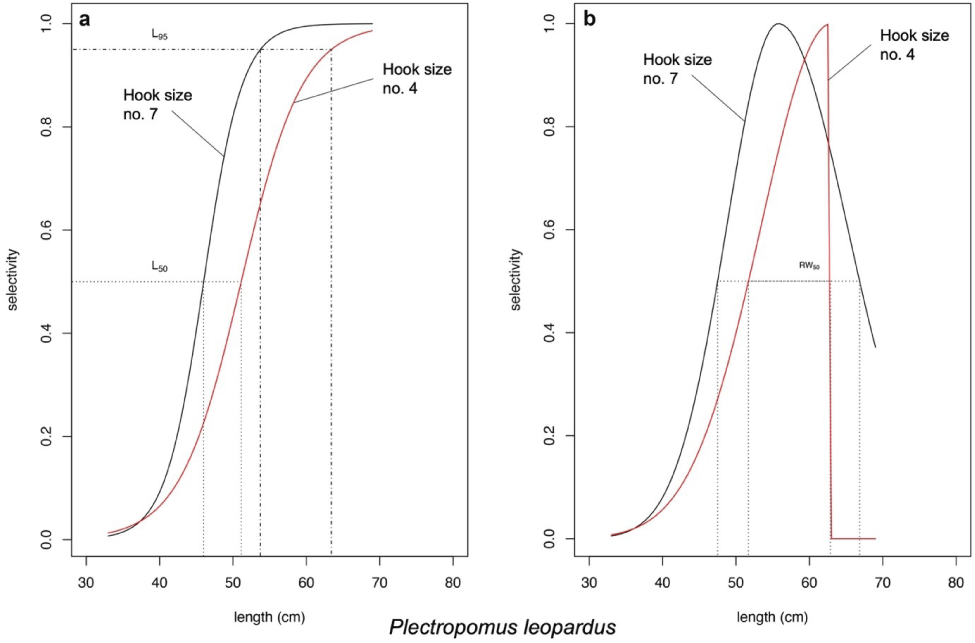
P. maculatus length fitted only the bell-shaped selectivity for both hook size numbers (Fig. 7). Similarly, like P. leopardus, the bell-shaped selectivity analysis of P. maculatus resulted in Lmax of the hook size number 7 was smaller (62.47 cm) than the hook size number 4 (65.75 cm; Fig. 7). The bell-shaped retention widths RW50 were 14.33 cm (hook size number 7) and 10.23 cm (hook size number 4; Fig. 7).
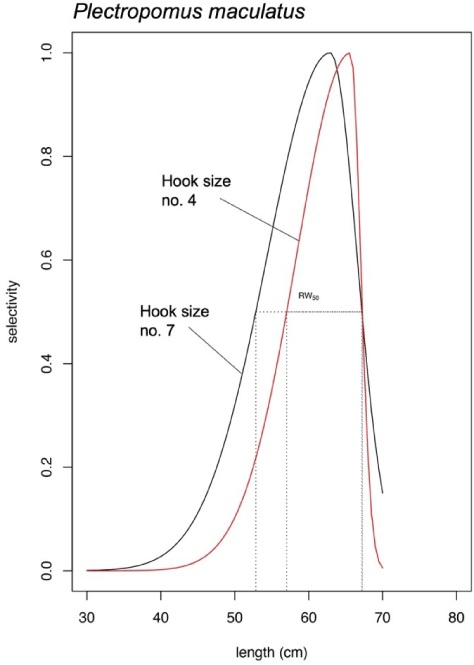
Discussion
This study shows that increasing hook size influence grouper’s catch size, increasing the Lc of both P. leopardus and P. maculatus. However, note should be taken that the normal scale model of P. leopardus and the lognormal model of P. maculatus generated maximum selectivities beyond the length infinity of each species (max. selectivity of 87.0 and 79.0 cm; length infinity of 71.9 and 76.6 cm, respectively). Accordingly, selectivity curves of the larger hook size (hook number 4; Figs. 4 and 5) seem impossible. Based on this finding, we then only discuss the result of selectivity based on the model from Hoshino et al. (2020).
According to the model from Hoshino et al. (2020), asymptotic selectivity of P. leopardus shows an increment of ~5 cm of Lc between the hook sizes. The use of larger hook size (hook number 4) generated a new curve (the red solid line in Fig. 6A) that shifted the curve to the right, reflecting more fish (fish length < 45.28 cm) could escape from fishing. This asserts that fishing activity using the larger hook size has given more opportunity for fish to grow until it reaches adult size.
The difference in Lmax generated from bell-shaped selectivity for both P. leopardus and P. maculatus were at least ~3 cm. The use of larger hook size (hook number 4) generated new curves (the red solid lines in Figs. 6B and 7) which show narrower retention probability. The area under the curves indicates the group of fish being retained by the gear, while the one above the curve indicates the group of fish could escape from fishing. For P. maculatus, the use of larger hook size generated a thin shape curve (the red solid line in Fig. 7) which shows that the probability of fish being retained by the gear is smaller than when the fishers use the smaller hook size (the black solid line in Fig. 7), thus increasing the probability of P. maculatus to reach maturity before being caught. The bell-shaped selectivity was also used by Sistiaga et al. (2019) for shrimp fishery, and the span length of the catch was explained by referring to retention probability.
There are various forms of fishing selectivity. Four are broadly defined by Sampson & Scott (2012), while Cadrin et al. (2016) added ‘knife-edged’ selectivity as the simplest form of asymptotic selectivity. Choosing the accurate model selectivity can be based on field experiments or statistical assumptions, as presented in this study.
When the idea of changing hook size was first proposed in Saleh bay, the objective was to increase the minimum length of catch (Lmin) from 19.86 cm to 25 cm for P. leopardus and from 20.69 cm to 26 cm for P. maculatus to achieve the spawning potential ratio (SPR) of 30% (DKP NTB, 2018). Lmin was used as a monitoring parameter for the FMPoA of groupers and snappers in the Saleh bay as it is easy to be implemented. The monitoring parameter of Lmin was part of the agreement between local stakeholders after a series of discussions, which also involved simulation on length-based spawning potential ratio analysis. Prior to the enactment of FMPoA in early 2018, a set of SPR values were generated for 11 priority species, including P. leopardus and P. maculatus, and the SPR of these 2 species were 24% and 21%, respectively. Following Ault et al. (2019), for reef fish species, the range SPR of 20%–30% is considered as fully exploited, hence fishing regulation to control catch size is necessary to maintain its sustainability. Even though the FMPoA uses SPR as a stock health indicator, estimation of gear selectivity provides a quick look into whether hook size regulations would have a positive impact on the stock itself.
The size of fish caught by the fishing gear is affected by the compatibility between the hook size and fish mouth opening (Kurnia et al., 2015). The larger the hook size, the larger the fish being caught (Otway & Craig, 1993). However, changes in hook sizes might not significantly change the selectivity curve, as catch size selectivity can be affected by other factors, such as fish distribution, feeding competition, and hook design (Erzini et al., 1996). This might explain no significant difference of length frequency distribution of P. leopardus between the hook sizes in the present study. No significant change was also found for the SPR of the P. leopardus from SPR of 24% (in 2017) to 25% (in 2019; Agustina et al., 2020). In contrast, a significant difference was observed for P. maculatus, where a larger hook size significantly resulted the increase of SPR from 21% (in 2017) to 30% (in 2019; Agustina et al., 2020). However, follow-up studies and long-term monitoring are still needed to have a better understanding of the impact of hook size and hook design on catch size including impact on other species and family.
Strategy for optimizing yields can be generated from production models (Gulland, 1983) and involve the specification of fish size at which becomes susceptible to capture by the gear used (Millar, 1992). Various efforts, such as modification of gillnet mesh size (Najamuddin et al., 2018; Sbrana et al., 2007) and change of hook size (Alós et al., 2008; Otway & Craig, 1993) have been proposed to reduce by-catch or non-target species as well as to protect juvenile fish caught by non-selective fishing gear. The importance of selectivity for fisheries sustainability where only matured fish being caught promotes high sustainable yields at low levels of stock depletion (Vasilakopoulos et al., 2016). Sustainability, as proposed by Froese (2004), can be described through three indicators: 1) let them spawn, 2) let them grow, and 3) let the mega-spawners live. Following this rule-of-thumb, it is crucial for hook and line fishery to implement control by effort levels appropriate to the Lc that occurs (Mees, 1996). Estimating Lc for long-lived species, such as grouper, can result in an overall effort limit for a multispecies fishery as it will ensure maximum return from the fishery, while protecting also all the species within it. Biologically, members of family Serranidae are long-lived and slow growing with relatively low rates of natural mortality. Their length at maturity tends to be high, but their reproductive capacity is limited, and they are prone to overfishing (Hoggarth et al., 2006). The length at maturity is also useful, but if unknown, management measures may be based on knowledge of Lc (Hoggarth et al., 2006). This study, especially for P. maculatus, shows how changing the hook to the greater size affects a larger Lmax and shifts selectivity curve to the right, potentially beyond the length at first maturity; to let them grow and spawn to achieve sustainability for slow growing species.
Development of a fishing regulation requires knowledge of fishing gear selectivity (Huse et al., 2000) as the appropriate specification is necessary when constructing fishery stock assessment models (Maunder et al., 2014). In the case of current fishing regulation implemented in the West Nusa Tenggara province, it was the local fishers’ genuine knowledge that hook number 7 (commonly used by the fishers) will be suitably replaced by hook number 4 to achieve the targeted catch size. From a practical point of view, changing the hook to a larger size could essentially increase fishing efficiency, since fish at the larger size will be caught, resulting higher market price compared to the smaller fish. From an economic perspective, changing hook does not require significant investment, since the difference of price between hook number 4 and hook number 7 is less than 1,350 IDR (< 0.1 USD) per hook, and per fishing trip it needs about 3 hooks, or increasing investment about < 0.3 USD. This small investment resulted in an increasing average size of P. maculatus, from 50.92 cm to 54.91 cm (or from 1.8 kg to 2.2 kg, based on length-weight relationship by Froese & Pauly 2023). Based on our data, the catch per trip did not change between using hook number 7 and hook number 4, about 1.6 individuals per trip, when the price of P. maculatus was 89,000 IDR per kg, the income of fishers increased 66,000 IDR (> 4.5 USD) per trip. However, convincing fishers to change the hook size is a challenging task unless they are involved in the decision-making process. High resistance of fishers to follow minimum-size regulations, particularly in small-scale fisheries, is likely to happen, and conflicts with other fishers who use gill nets might arise as there is no in-place regulation applied for gill nets mesh size. To minimize undesirable effects, fisheries managers are encouraged to develop management plans based on a mutual agreement involving related parties, including fishers and market players (capital owners/investors) who have a strong influence in small-scale fisheries economy systems. A regulation should be applied not only at the fish harvesting level (i.e., fishers) but also to a business level where the fish traders and collectors are not allowed to sell the fish under the agreed size.
Conclusion
This study shows that changing hook sizes affects the catch size. Larger hook size increases Lc of the fish and results in higher maximum selectivity. The use of a larger hook size can reduce the likelihood of catching smaller fish and allow sufficient time for them to reach sexual maturity and spawn before being caught. Although the effects may vary for different species, hook size can be used as a management measure of harvest control rules to improve grouper stock in Indonesia. Further study is needed to investigate the effect of hook size and hook design to other species, total catch, and efficiency since it will benefit fishers and increase the compliance to the fishing rules.