Introduction
Rainbow trout (Oncorhynchus mykiss), a highly valued foodfish native in the cold-water rivers and lakes of Northern Pacific has been locally domesticated and cultured in South Korea for over 50 years (Kim et al., 2017; Woynarovich et al., 2011). In 2019, rainbow trout accounts 97% of the total volume of trout production, and 1.5% of the total inland world aquaculture production in 2020 (FAO, 2022). In South Korea, there is a recent decrease in production of rainbow trout from 3,825 tons in 2019 to 2,414 tons in 2020 (KOSTAT, 2021). Nevertheless, it is still one of the sought fish products in the local seafood industry. In the global arena, the husbandry of this species is still continuously expanding owing to several aquaculture advantages including easy spawning, rapid growth, wide tolerance to environmental factors and spawning manipulation to produce year-round eggs (Hardy, 2002). According to Valente et al. (2013), increased fry output is essential for the aquaculture sector to grow successfully and competitively. In rainbow trout aquaculture, hatching and development of sac-fry and rearing of fry are parts of works in the hatchery, in addition to selection of suitable broodfish and collection and incubation of eggs (Hoitsy et al., 2012). Proper larval rearing of this species ensues a high survival rate and maximum growth potential for a continuous supply of seeds for the grow-out culture.
The somatic growth is one of the important aspects in the aquaculture industry and is regulated by various endogenous (e.g., physiological), nutritional, genetics and environmental factors (Sumpter, 1992). Generally, in mature fish, body growth is compromised due to energy partitioning brought by simultaneous sexual maturation (Jobling, 1993). However, this effect is concealed in immature or juvenile fish (Piferrer et al., 2009; Sheehan et al., 1999) which is not yet physiologically ready for gonadal maturation. To augment the effect of reproductive growth on body growth, triploid induction is often used in aquaculture (Maxime, 2008; Tiwary et al., 2004). Triploidy, however, does not only deal with sexual sterility, but also on the behavior, cell size and possession of genomic heterozygosity (Garner et al., 2008; Piferrer et al., 2009).
Triploidy is a genetic manipulation technique employed to attain three sets of chromosomes, instead of two in diploids (Maxime, 2008). This is a result of blockage of second meiotic division or prevention of the extrusion of the second polar body in the egg (Tiwary et al., 2004). The resulting triploid animal over diploid counterparts has increased cell size, possession of high level of total genomic heterozygosity and genetically infertile or sterile incapable of sexual maturation (Ihssen et al., 1990; Piferrer et al., 2009; Tiwary et al., 2004). Due to its economic importance, O. mykiss has also been a popular experimental fish for various triploid research, including methods of induction and biological production (Solar et al., 1984), method of detection (Thorgaard et al., 1982) and comparative growth (Guo et al., 1990; Sheehan et al., 1999; Weber et al., 2014). In Korea, the first triploid induction of O. mykiss was reported in 1986 (Kim et al., 1986). Consequently, Kim et al. (1988) studied growth and gonad maturation in 4-,8- and 12-month-old triploid fish. Since then, no comparative growth data between diploid and triploid rainbow trout reared in South Korea have been available, particularly during sac-fry development. Since, triploid induction happens during post-fertilization, it is inevitable to think that it can affect early-growth of fish (Solar et al., 1984). Hence, the current study compared the growth of diploid and triploid O. mykiss focused in the early life stages, from alevins (post-hatching) to fry stage.
Materials and Methods
Rainbow trout diploid and triploid eyed eggs (n ≈ 40,000 each) obtained from a private fish farm in Pyeongchang, Gangwon province were transferred and stocked in plastic trays (net size 3 × 3 mm) floated on polypropylene tanks (4 × 4.5 × 0.5 m) located in a fish farm in Andong, North Gyeongsang province, South Korea. These culture tanks were continuously supplied with freshwater via a flow-through system. The fish were cultured from stocking of eggs until 134 days post-hatching. After hatching, the swim-up fry was hand-fed twice daily (7:00 AM and 5:00 PM) with commercial feed. Dead fish were daily monitored and removed from the tanks. The water conditions during the rearing period were as follows: temperature (13 ± 0.5°C), pH (6.6 ± 0.3) and dissolved oxygen (6.9 ± 0.3 ppm).
The O. mykiss eyed-egg samples were subjected to polypoidy detection using flow cytometric method as per Chaiton & Allen (1985). Briefly, 100 diploid and triploid eggs were separated on the day of stocking and immediately frozen in liquid nitrogen and stored at –80°C before the analysis. Each sample sets were treated with nuclei extraction buffer (Partec GmbH, Meckenheim, Germany) and stained subsequently with 4´6-diamidino-2- phenylindole (DAPI). The samples were run on a flow cytometer (Flow Cytometer, Partec GmbH) to measure the amount of DNA present.
The egg-yolk from thirty diploid and triploid rainbow trout alevins was incised from the body of the fish after fixation in 10% neutral buffered formalin and subjected to morphological measurements. The yolk weight was measured using an electronic balance to the nearest 0.01 g. The yolk length (YL) and yolk height (YH) were measured using a vernier caliper to the nearest 0.01 mm. The yolk volume (YV) was calculated as YV = (π/6) (YL) (YH)2 (Blaxter & Hempel, 1963). Egg-yolk measurements were done zero days after hatching (DAH 0), seven days after (DAH 7) and interval of three days thereafter until full absorption at DAH 22 (Fig. 1).
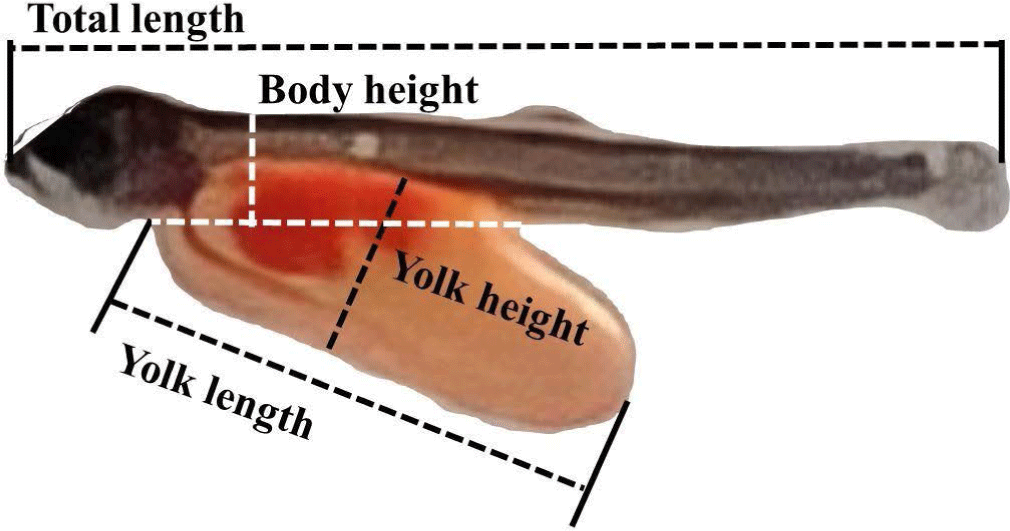
The body morphometrics of diploid and triploid rainbow trout (n = 30) alevins were measured at the same timepoints as the egg-yolk measurements. Further, the body growth of fry was measured from DAH 36 until DAH 134 with sampling interval of every 14 days. The body weight was measured using an electronic balance to the nearest 0.01 g. The total length and body height were measured using a vernier caliper to the nearest 0.01 mm (Fig. 1).
Results
The amount of DNA in diploid and triploid rainbow trout is shown in Fig. 2 revealed by flow cytometric analysis. The triploid fish had a slightly over the expected amount of DNA, which is generally 1.5× than that of diploid.
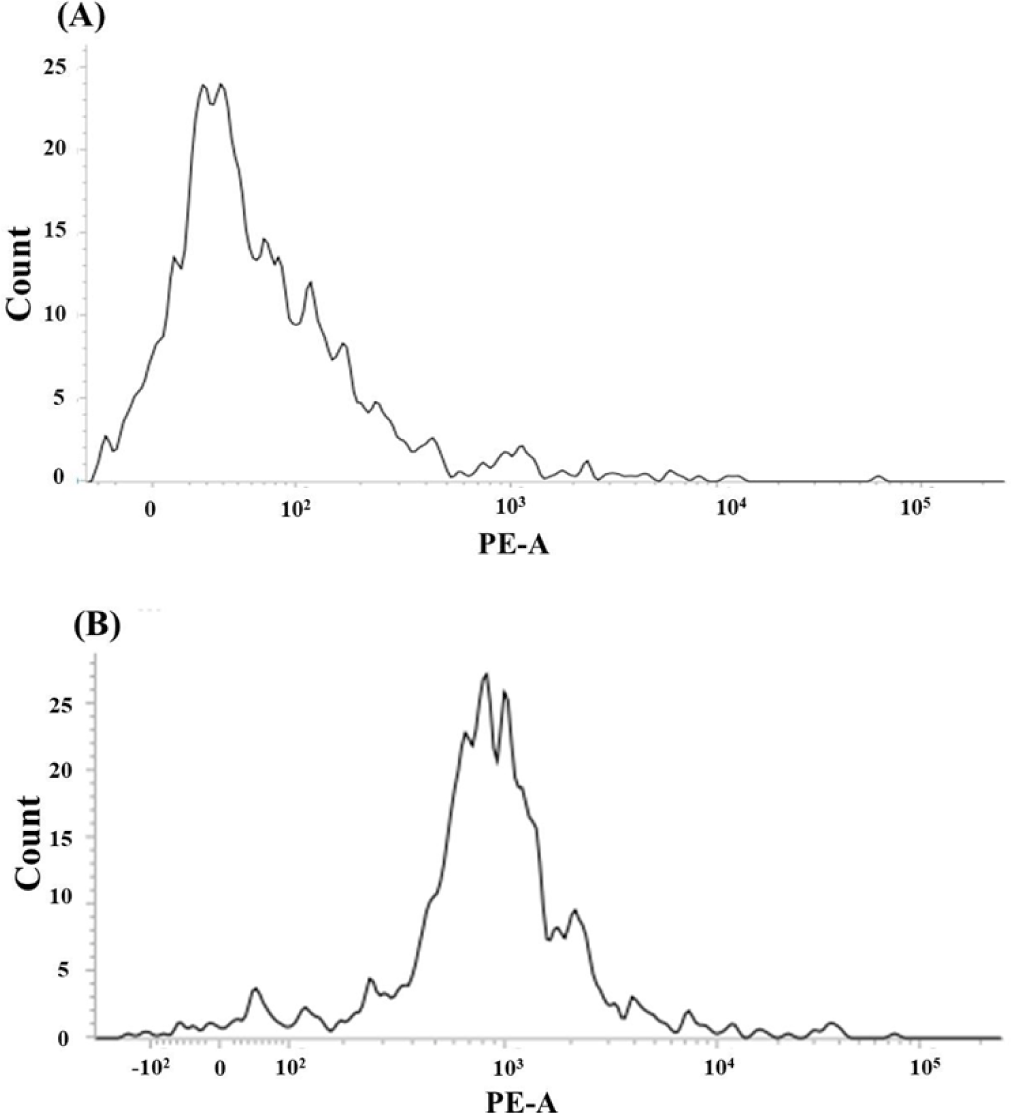
The changes in the egg-yolk morphometrics are shown in Fig. 3. The egg yolk length and height are longer, egg yolk weight is heavier and egg yolk volume is larger in triploid over diploid alevins in every sampling point from DAH 0 to DAH 22. Nevertheless, difference was not statistically significant (p > 0.05).
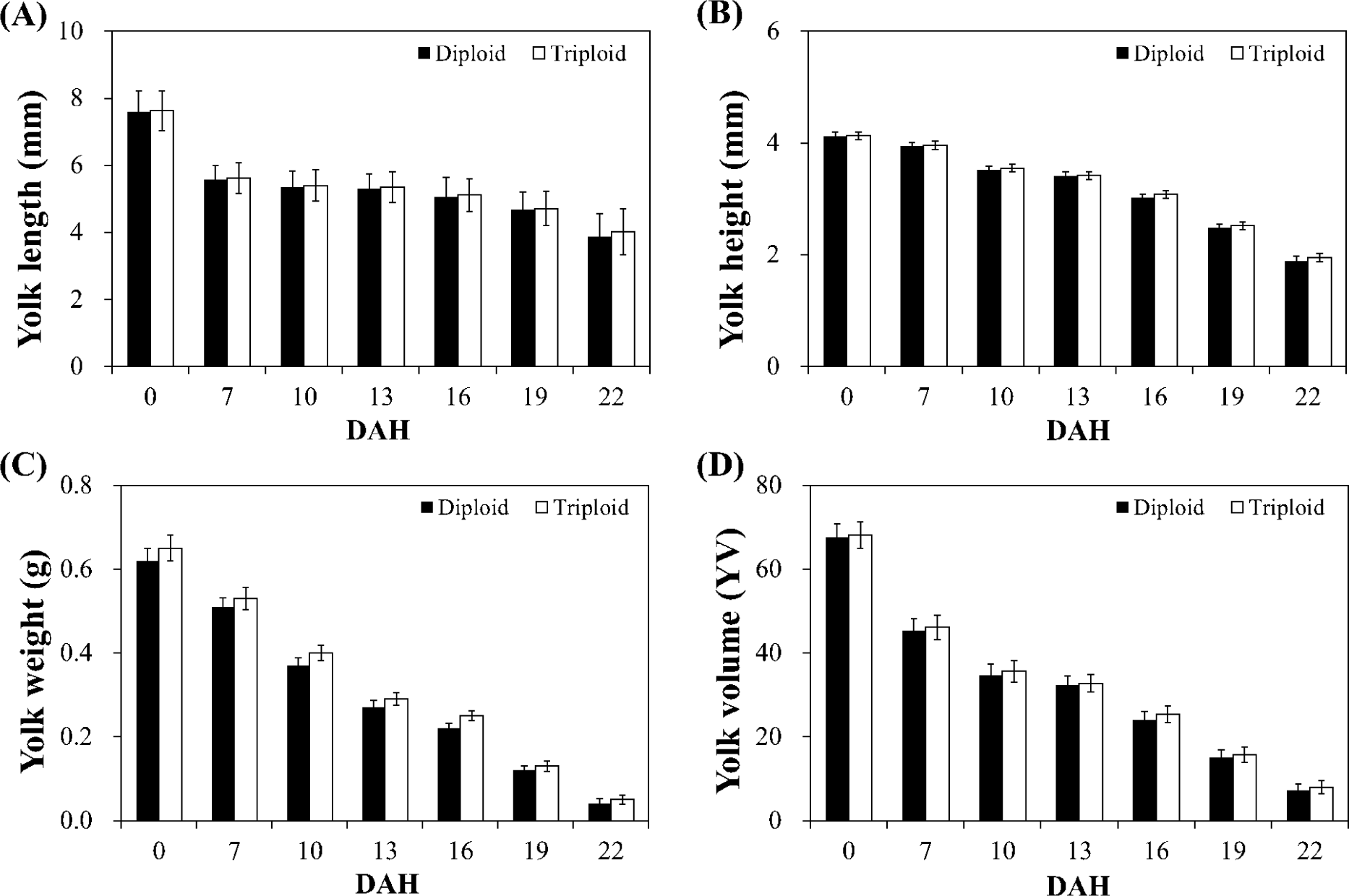
Diploid rainbow trout had a significantly (p < 0.05) longer total body length from DAH 10 until DAH 92, body height at DAH 7, DAH 13, DAH 50, DAH 64 and DAH 92, and heavier body weight from DAH 16 to DAH 22 and from DAH 50 to DAH 92. However, starting from DAH 106 until the completion of the study at DAH 134, triploid overtook diploid fish and had a significantly better growth performance (p < 0.05) in three body morphometrics measured (Fig. 4).
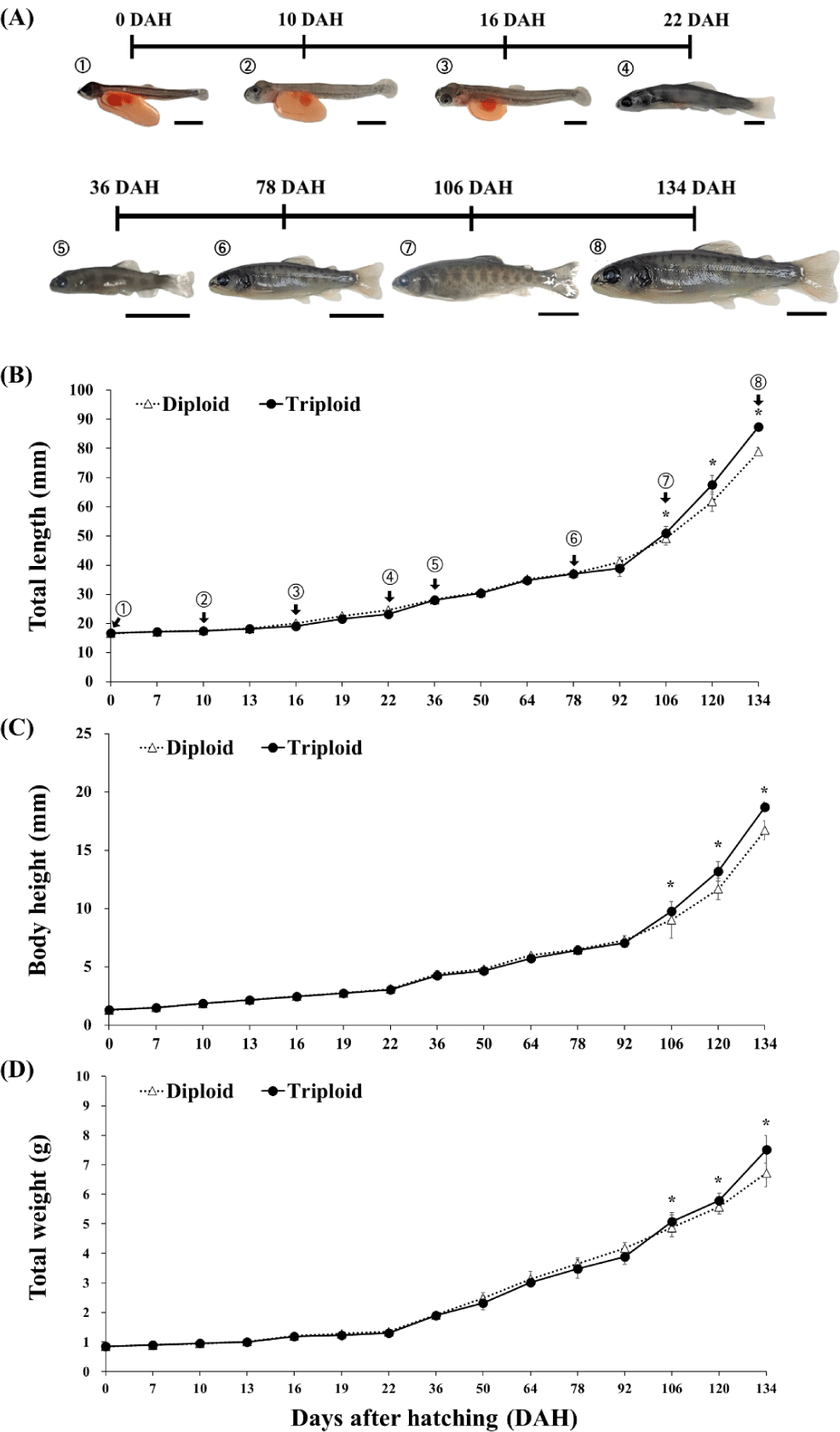
Discussion
Flow cytometry has been used to discriminate between diploid and triploid fish populations (Tiwary et al., 2004). Flow cytometry is a rapid and accurate ploidy detection method that involve cells staining with a DNA fluorescent dye and quantification of its fluorescence upon light excitation (Allen, 1983). Ploidy analysis by flow cytometry has been conducted in fish and shellfish (Chaiton & Allen, 1985; Thorgaard et al., 1982). In the current study, the amount of DNA present in the fish samples were determined through flow cytometry and discriminated diploid and triploid rainbow trout. The methods of induction routinely used to produce triploids are described and reviewed elsewhere (Piferrer et al., 2009; Tiwary et al., 2004). In the current study, the method of induction of rainbow trout triploids is unknown due to the disclosure policy imposed by the facility where the eggs were sourced. To determine the ploidy level of the received eggs, flow cytometry was employed. The results showed that the DNA content of the triploid level fish had an over than expected amount of DNA, which warrants possible explanations. The amount of DNA in triploids is generally, on average, 1.5 times that of diploids and can reach up to 1.8 times (McCarter, 1988). The variation described herein can result from combinations of several reasons, such as the limited number of specimens analyzed, differential staining of specimens, and contamination during sample preparation (Chaiton & Allen, 1985). In addition, there is also a possible unintentional variation from the analysis conditions, which influence cytochemical equilibria, and further differences in the DNA activity, which modulate the quantity of stain that binds to the DNA that can ultimately affects fluorescence quantification (Chaiton & Allen, 1985; and references therein). Collectively considered, flow cytometry is an efficient tool in discriminating diploid and polyploid populations, but to be handled carefully to produce reliable and unquestionable results.
In salmonids, alevins (hatched larvae) are accompanied with dense, yellowish liquid called yolk-sac containing globulins and oil droplets. At hatching, yolk-sac of the alevins could reach up to 75% of the entire body weight (Hoitsy et al., 2012). Alevins feed on this yolk-sac through absorption before and even before exogenous feeding began (Woynarovich et al., 2011). The available amount of yolk is shown to be positively correlated to the larval growth rate (Happe et al., 1988) and that fast absorption of the yolk means fast growth (Myoung & Kim, 1993). In grass carp (Ctenopharyngodon idella), larvae produced from the mating of diploid and triploid fish had smaller yolk areas compared to the larvae of both diploid parents, and small yolk areas correlates to slow yolk utilization and consequently affect survival before exogenous feeding began (Van Eenennaam et al., 1990). Indeed, comparable yolk areas would suggest indifference in metabolism, growth and survival (Blaxter & Hempel, 1963). In the current study, no significant difference in the egg-yolk morphometrics and non-significant better alevin growth (DAH 0 to DAH 22) in triploid fish was observed which means that triploid induction weakly influenced growth at this early larval stage.
The comparative somatic growth study between diploid and triploid juvenile fish has been studied, however, there are inconclusiveness due to contrasting results (Piferrer et al., 2009; Tiwary et al., 2004). Swarup (1959) made the first report of comparing growth in three-spine stickleback (Gasterosteus aculeatus), and reported that triploid fish did not show any increment in body size against the diploids. Since then, various attempts on triploidy induction have been published and consequently investigated growth difference. In non-salmonid species, Valenti (1975) reported that triploid tilapia (Tilapia aurea) had a better growth within 14 weeks of age. Similar result was obtained in Far Easter catfish (Silurus asotus; Kim et al., 2001). In contrast, triploid juvenile or immature fish was inferior in common carp (Cyprinus carpio; Cherfas et al., 1994), or had similar growth with diploid, for example, in tilapia (Oreochromis niloticus; Brämick et al., 1995).
In the current study, in over three months post-hatching (until DAH 92) diploid rainbow trout had either similar or slightly better growth but subsequently, triploid overtook diploid until the 19th week post-hatching (DAH 134). This is quite similar from the study of Guo et al. (1990) wherein triploid O. mykiss had inferior body length and weight than diploid at first 50 days of post-hatching, and one triploid strain had a better growth than its diploid counterparts at the end of a 233-days post-hatching growth measurement. Similarly, growth of induced triploid O. mykiss started slower than the diploids but then accelerated thereafter in 8-to-22 week early-growth observation (Weber et al., 2014). Galbreath et al. (1994) similarly observed the same pattern in Atlantic salmon, Salmo salar. Nevertheless, there are also contrasting results within the salmonid family. For instance, triploid rainbow trout, Salmo gardneiri (= O. mykiss) had inferior body weight versus the diploid fish during the 24-to 48-week growth trial (Solar et al., 1984). Similarly, the growth of diploid juvenile coho salmon Oncorhynchus kisutch was faster than triploids (Withler et al., 1995). Kim et al. (1988) in rainbow trout published that diploid fish showed a better growth rate before maturation. Interestingly, other reports showed no difference. For example, in O. kisutch (Withler et al., 1998) and Oncorhynchus tshawytscha (Garner et al., 2008).
A consistent difference in growth performance of diploid and triploid fish seemed nil (Garner et al., 2008), and contrasting difference could stem from several hypothetical and experimental reasons (Felip et al., 2001; Maxime, 2008; Piferrer et al., 2009). This includes concerned species, environmental conditions (Piferrer et al., 2009), rearing procedures (Maxime, 2008; and references therein), methods of induction, difference in cell size and number (Felip et al., 2001; and references therein), specific strains (Guo et al., 1990) and life stages e.g., immature versus mature (reviewed by Piferrer et al., 2009). Sheehan et al. (1999) suggested that, before sexual maturation, triploids had no intrinsic higher growth rate over diploid counterparts. However, several studies (Galbreath et al., 1994; Guo et al., 1990) and the current findings revealed that triploids can grow faster than diploids during the fry/juvenile/immature stage, and comparative difference could result from one or combined reasons mentioned.
The positive effect of triploid induction in somatic growth was reviewed by Piferrer et al. (2009) and include the gigantism effect in cell size, total genomic heterozygosity and energy partitioning between somatic and reproductive growth. The last effect holds true only in adult fish; thus, the former two are applicable to immature fish. There is a general rule that an increase in chromosome number positively correlates to the size of the nucleus and overall cell size (Swarup, 1959), however, triploids having more, do not intrinsically correlate to better growth performance over diploids (Ihssen et al., 1990). This is due to the concurrent decrease in the number of cells (Benfey, 1999). Triploids contain higher genomic heterozygosity over diploids (Leary et al., 1985) due to coding of three different alleles at every polymorphic locus (Piferrer et al., 2009). Growth and heterozygosity had contrasting positive and negative relationship in published studies (Garner et al., 2008). Triploid heterozygosity is also affected by how triploid animal was produced. Ihssen et al. (1990) presented that the triploid produced from mating a tetraploid and diploid had higher viability over physically-induced triploids. Overall, the increase in triploid growth in the present study can stem from either or both positive effects, and is suggested for further study.
Ultimately, triploidy is a useful genetic technique to attain fast growth of cultured animals, reduced mortality, and financial profitability due to improved production (Berrill et al., 2012), and its effect is not only seen in adult fish but also during the early-growth of fry and juveniles, as seen in the current study in rainbow trout.