Introduction
Recently, environmental issues closely associated with human health have become matters of primary concern, as the quality of life becomes higher along with the enhancement of the living standard. Across industries, eco-friendly management, especially for waste and water quality, and establishment of sustainability policies have gained importance. Microorganisms have been receiving increasing attention owing to their potential in replacing agricultural chemicals such as chemical fertilizers and pesticides (Dwivedi et al., 2021; Sharma et al., 2021). The culture of beneficial microorganisms is being researched in more than 130 laboratories across Korea, and the cultured microorganisms are coming into wide use (Cho et al., 2019). Photosynthetic bacteria (PSB), lactic acid bacteria, actinomyces, and filamentous fungi are some examples of such microorganisms (Higa, 1996). PSB are diversely utilized: to increase plant growth by soil amendment (Lee & Song, 2010; Sunayana et al., 2005); to prevent animal diseases by improving barn environment (Han et al., 2008); to purify water; to remove odors (Do et al., 2003; He et al., 2010; Lu et al., 2019b); to produce single-cell proteins (Kornochalert et al., 2014; Saejung & Thammaratana, 2016); and to produce polysaccharides, carotenoids, chlorophylls, coenzyme Q10, and 5-aminolevulinic acid (Meng et al., 2017; Wang et al., 2017; Zhou et al., 2015b). Moreover, vitamin B12 produced by PSB is used for the treatment of anemia and neuritis and can also be used as an animal growth-stimulating feed source (Sasaki et al., 2005). Moreover, the technology of clean hydrogen production by PSB is noteworthy because hydrogen produced from organic waste is considered a promising substitute energy source (Cai et al., 2019; Moreira Silva et al., 2016). As a high-value material, microorganisms are also used in foods, medicines, and cosmetics (Kars & Ceylan, 2013; Lu et al., 2019a; Sasaki et al., 2005). Particularly, the Rhodobacter species are used to treat organic compounds at high concentrations in wastewater because it prefers organic compounds to inorganic compounds as hydrogen donors for photosynthesis (Cheong et al., 1997; Lee, 1971). This bacterial genus is also used as a component in food and fertilizers.
PSB have been extensively used in environmental bioremediation and bioenergy production owing to their versatile metabolic abilities. They also play a role in biofertilization, biostimulation, and biocontrol mechanisms to promote plant growth (Lee et al., 2021). However, culturing PSB is challenging and expensive because of difficulties in culturing them without contamination, which necessitates quality control (Cho et al., 2006). This leads to problems in scaling up for mass production, which makes it complicated to make PSB available for diverse applications. Therefore, although PSB have diverse applications in many fields, they have not come into widespread use. To date, Rhodobacter capsulatus and Rhodobacter sphaeroides are the species currently registered as a subsidiary feed in Korean law of animal feed standards (The Korea Law Information Center, 2016). To ensure distribution of good-quality PSB for public use, more research on cultural characteristics and scale-up cultivation of PSB is indispensable, and a simple culture technique that can be used by non-experts needs to be developed.
Therefore, in the present study, a simple culture technique for a photosynthetic bacterium was developed, and its suitability for environmental fields was demonstrated not only to simplify the cultivation of useful PSB but also to disseminate this technique to the public. Using this technique, owing to the maintenance of high cell activity, is expected to be cost effective over the long term in comparison with purchasing microbial products available in the market on several occasions. In this study, the cultivation of the standard species R. capsulatus was first investigated on biomass analysis, and then compared with three other species of useful PSB to select a species suitable for scale-up cultivation. Subsequently, the selected species was cultivated in a 500 L reactor to identify the optimum culture conditions for scale-up. Then, these cultivated PSB were used as seed culture for cultivation in a 15 L simple reactor to develop an easier culture technique suitable for public use. Moreover, the cultivated PSB were preserved at 4°C, and then the preserved PSB were used as seed culture to perform cultivation in a 250 L simple reactor to determine the optimum preservation period that allows cell reactivation. Finally, the selected PSB species cultivated by a simple culture technique was tested its potential in in the removal of NH4+-N in shrimp aquaculture water.
Materials and Methods
The species of PSB used in this study was R. capsulatus BR, which was obtained from Ecobiznet Company (Chuncheon, Korea). R. capsulatus BR was cultivated on various culture media (Table 1). To eliminate the possibility of contamination, red colonies of R. capsulatus BR formed on a solid MYC culture medium containing 1.5% agar was transferred with a loop to a 1 L flask (with a working volume of 600 mL) using the MYC culture medium; the flask was incubated for 5 d at 30°C and 14.86×g under a light intensity of 1,000 Lux (Saejung & Thammaratana, 2016). The condition of cells was periodically observed under a microscope, and the optical density (OD) and cell viability were also measured to assess cell growth during the cultivation period.
Scale-up cultivation of PSB is requisite to ensure their effective use in diverse applications. Accordingly, the suitability of R. capsulatus for the scale-up cultivation was explored, and its cultural characteristics were compared with those of other generally utilized PSB species in environmental fields (Ansari et al., 2021; He et al., 2010)—Rhodobacter azotoformans EBN-7, R. sphaeroides BL6, and R. sphaeroides GB1—which were obtained from the Ecobiznet Company.
Red colonies of each PSB species formed on a solid MYC culture medium was transferred with a loop to a 1 L flask (with a working volume of 600 mL) using the MYC culture medium, and then the flask was incubated for 5 d at 30°C and 14.86×g under a light intensity of 1,000 Lux. To increase cell activity, each bacterial species was preliminarily incubated in the MYC culture medium three times for adaptation. The carefully adapted seed culture was used for the scale-up cultivation, and the selection of species was based on the specific growth rate, number of viable cells, and cell purity.
After a PSB species and its optimum culture medium were selected, pilot-scale cultivation was performed in a 500 L bioreactor (KoBio Tech, Chuncheon, Korea). The seed culture was prepared in a 1 L flask incubated at 30°C and 14.86×g under a light intensity of 1,000 Lux. During cultivation, the cell activity was periodically observed under a microscope, and the color tone of cell broth was compared with the red–green–blue (RGB) color chart to check the possibility of contamination. When the seed culture reached a stage at which high cell activity and high cell purity were maintained, a 500 L bioreactor (with a working volume of 250 L) was inoculated with 3% (v/v) inoculum and incubated at 30°C for 5 d under a light intensity of 1,000 Lux. During the cultivation, aeration and agitation rates were adjusted on the basis of cell growth and consumption of dissolved oxygen (DO). Air was initially supplied at 55 L/min, and this flow rate was gradually increased to 70, 85, and 100 L/min at 24, 48, and 72 h, respectively. Simultaneously, agitation speeds were gradually increased to 2.19, 3.06, and 3.55×g at 24, 48, and 72 h, respectively.
With the aim of developing a simple technique for cultivating the seed culture of PSB, cultivation was performed using a simple reactor to explore whether this technique is applicable to seed culturing. The simple reactor was a 15 L, open-type, rectangular glass tank (with a working volume of 10 L) with a continuous air supply at 0.05 vvm with a submersible pump (10 W; UP100, Hyup-Shin Water Pump, Seoul, Korea). The culture medium used in this study was the MYC culture medium (initial pH 7; Ecobiznet Company). The 500 L bioreactor was inoculated with 1% (v/v) PSB inoculum and incubated at 30°C and 0.11×g for 3 d under a light intensity of 1,000 Lux. The final culture broth was tested for its suitability as seed culture.
The possibility of preserving PSB for a long period at 4°C is important for its practical use. To assess the optimal preservation period, the PSB cultivated in a 500 L bioreactor was preserved at 4°C for various periods (1, 2, 3, and 4 months, respectively) and then cultivated in a simple pilot-scale reactor (250 L; Ecobiznet Company) to investigate the optimal preservation period to maintain cell activity at 4°C. The 250 L reactor was made of type 304 stainless steel, and the installed apparatus included those for aeration, agitation, and temperature control with cooling and sterilization. With the touch of a button, sterilization process (with increase in temperature up to 100°C) started for 2 h after the reactor was filled with the MYC culture medium. Then, the reactor temperature was reduced to 30°C, followed by inoculation with 1% (v/v) seed culture preserved at 4°C for different periods. This cultivation was automatically continued for 3 d at 30°C and 0.11×g with an aeration rate of 0.05 vvm under a light intensity of 1,000 Lux according to the reactor system program initially inputted. In this manner, the effect of preservation at 4°C on cell activity was investigated to determine the optimal preservation period that allows for cell reactivation.
PSB cultivated using a simple technique was applied to environmental fields to explore its suitability as seed culture. The cultivated PSB species was R. azotoformans EBN-7, and it was used in a shrimp aquaculture farm (Ganghwa-gun, Incheon, Korea). Before breeding shrimps, R. azotoformans EBN-7 was added to the aquaculture farm at 100 mL/ton (with viable cells of 1.75 ± 0.23 × 109 CFU/mL) every week to improve water quality, and the concentration of NH4+-N was measured as an index using the indophenol method (The Korea Law Information Center, 2022).
The change in OD of cells during the cultivation period was examined by measuring the absorbance. The OD was determined at 660 nm using a UV-VIS spectrophotometer (Hach, Berlin, Germany) against distilled water as a control. Cell viability were periodically assessed by counting red colonies formed on the solid MYC culture medium, represented as CFU per mL. The pH of the culture broth was also measured. All measurements were performed in triplicate.
Results and Discussion
The species R. capsulatus is one of the most used PSB with wide applications. Therefore, the growth of R. capsulatus BR was first studied on various culture media (Fig. 1). The lag phase was approximately 20 h in all cultivation experiments on various culture media, which was followed by exponential growth, and the maximum growth was observed when a stationary phase was reached (Fig. 1A). The best growth was achieved when R. capsulatus BR was cultivated on the basal medium. When cultivated on the basal medium, the number of viable cells of R. capsulatus BR maximally reached 4.75 × 109 after 89 h, which was relatively higher than that previously reported—3 × 108 under dark-aerobic conditions or 3 × 109 under photo-anaerobic conditions (Costa et al., 2017). Although pH change was not significant during the lag phase, pH increased as cells grew exponentially (Fig. 1B), indicating that the change in pH was closely related to cell growth. The trend of change in OD was somewhat different from that in cell number (Fig. 1C). The OD started to increase after 24 h incubation, and thereafter it increased steadily even after the stationary phase of cell growth. Photosynthetic pigments are known secondary metabolites that are not essential for survival but are useful to increase bacterial viability under environmental stress (Orlandi et al., 2021). In the present study, there was a time gap between the maximum cell number and the maximum OD, which signifies a time gap between cell growth and the production of the red pigment by R. capsulatus BR. To identify the optimal culture medium for R. capsulatus BR, the maximum OD at 660 nm was determined. The maximum OD values were 3.96, 2.75, and 1.60 for the basal medium, van Niel’s medium and MYC medium, respectively. The growth profile of R. capsulatus BR on the basal medium indicates that the maximum specific growth rate was 0.12 h−1. On the basis of the above parameters, growth characteristic of R. capsulatus BR were distinctly identified.
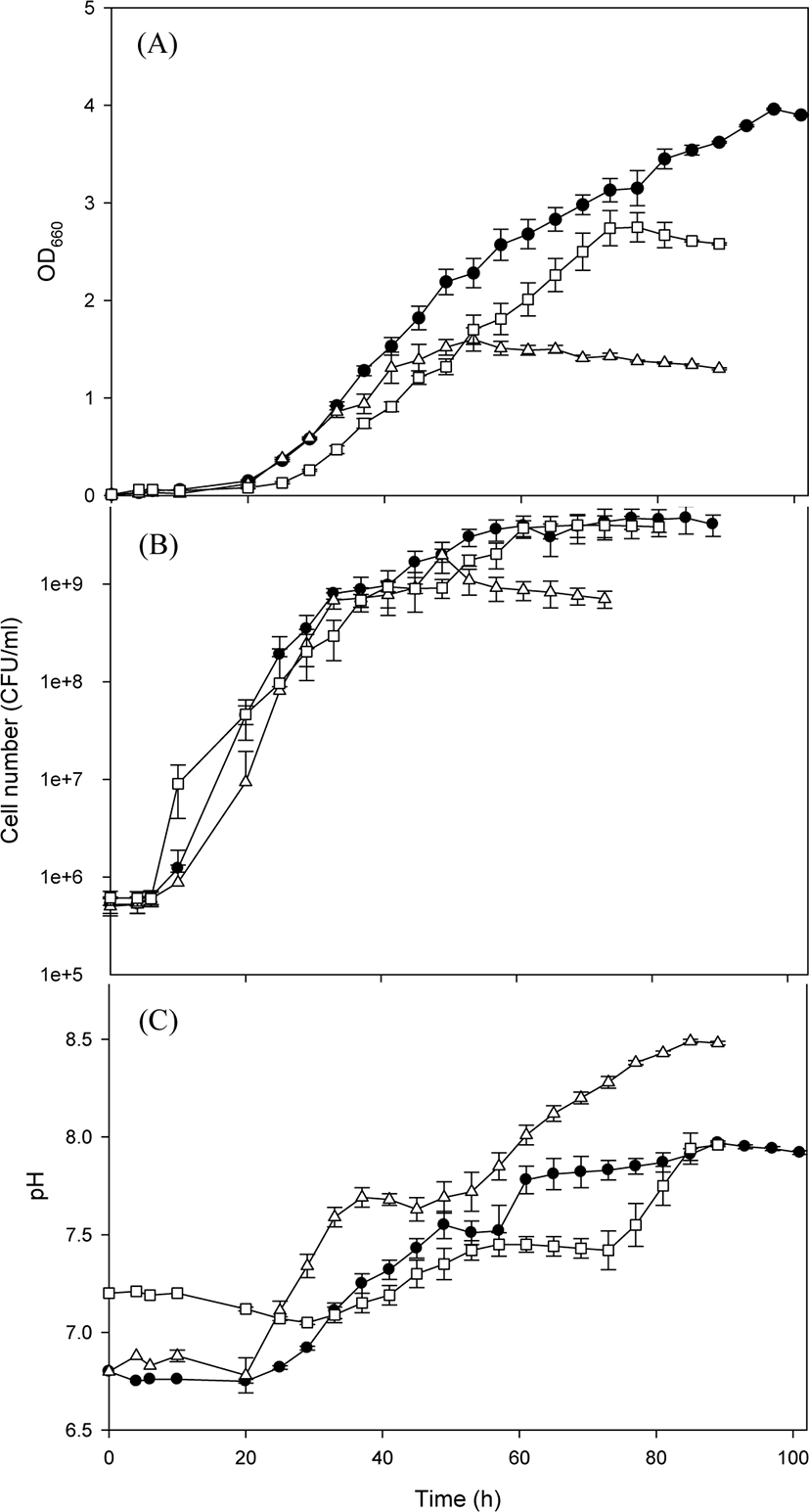
The color tones of R. capsulatus BR cultivated on various culture media were explored (Fig. 2). When R. capsulatus BR was cultivated on both the basal medium and the MYC medium, distinct changes in the color tone were revealed after approximately 20 h. According to the RGB color chart, the color tone changed from gold (#FFD700) to light coral (#F08080). This result was consistent with previous findings on PSB cultivation in which the color of the culture broth changed within 24 h (Meng et al., 2017). The change in the color tone to red is caused by the production of carotenoids or bacteriochlorophyll a and b that absorb the light beyond 800 nm wavelength (Kim et al., 2005; Madukasi et al., 2011; Maoka, 2020). In contrast, the color tone did not perceptibly change when R. capsulatus BR was cultivated on van Niel’s medium. This retardation in the change in color tone was presumed to result from low growth on this medium, as seen in Fig. 1. The highest red tone (red; #ff0000) was observed in the MYC culture broth at 24 h (Fig. 2B). This difference in red tone may be the effect of casamino acid included only in the MYC medium, indicating that a special nitrogen source is probably critical to exhibit the highest red tone. Because casamino acid is reported to contain a stimulating factor for the growth of PSB (Kumagai et al., 1968), the active growth of R. capsulatus BR by the addition of casamino acid may result in a redder tone. All culture media turned red (red; #ff0000) after 29 h cultivation. Subsequently, however, the color tone of R. capsulatus BR was different in different media: crimson (#DC143C) in the MYC medium and dark red (#8B0000) in both basal and van Niel’s media. Although the cultivation of R. capsulatus BR in the MYC medium revealed the highest red tone, the red color tone turned pale at the end of cell growth period owing to low cell growth (as observed in Fig. 1C).
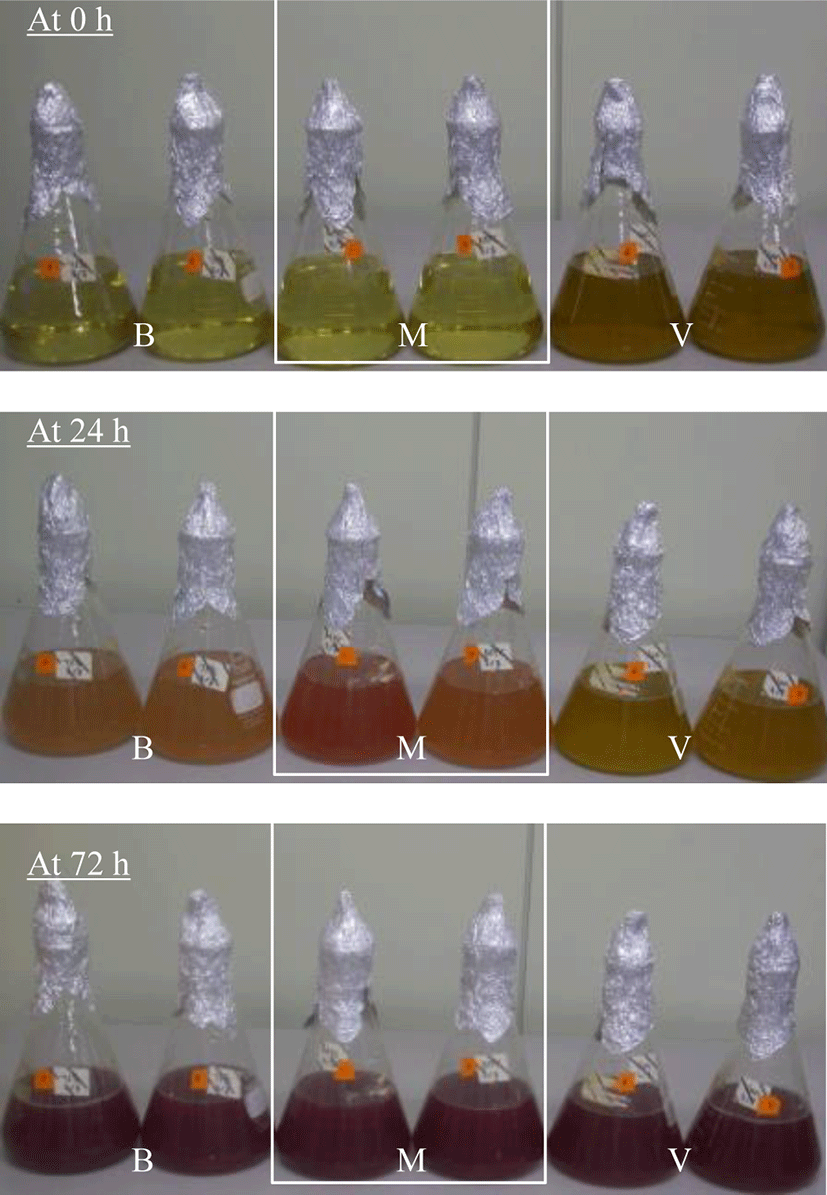
The morphology of R. capsulatus BR cultivated on various culture media for 101 h was explored by microscopy. The cells grown on the basal medium were relatively long (3−4 µm) and 1 µm wide, whereas those grown on the MYC medium were longer (4−5 µm) and less wide (0.5 µm). Cells grown on van Niel’s medium were shorter (1−2 µm) and oval (1 µm width). Thus, the morphology of R. capsulatus BR varied with the composition of the culture medium. The strain R. capsulatus has been reported to exhibit pleiomorphism dependent on environmental conditions. For example, a globular cell shape (below pH 7) changes to a rod shape above pH 7, and the cells lengthen as pH increases (van Niel, 1944). In this study, the strain R. capsulatus exhibited morphological changes on different carbon sources. During the cultivation period, cells often show aggregation and settle down, with a change in red tone, when they are contaminated or not properly cultured. Therefore, periodic observations of cell morphology are indispensable because contamination is the primary concern in the scale-up cultivation of PSB. The cultural characteristics (growth profile, color tone, and morphological observations) of R. capsulatus BR suggested that the basal medium was more suitable than MYC or van Niel’s medium for R. capsulatus BR culture.
After the basal medium was determined to be an appropriate medium, four test PSB species were cultivated in a 1 L flask using the basal medium to explore their growth characteristics (Fig. 3). The four PSB species showed logarithmic growth after a lag phase of approximately 15 h. Compared with the previous cultivation experiment, the duration of the lag phase was reduced owing to preliminary adaptation in the same culture medium. The measured maximum OD660 values were as follows: 6.67 for R. azotoformans EBN-7 after 109 h; 3.58 for R. capsulatus BR after 154 h; 5.15 for R. sphaeroides BL6 after 141 h; and 6.30 for R. sphaeroides GB1 after 154 h (Fig. 3A). On the basis of the OD660 values, the specific growth rate of R. azotoformans EBN-7 was calculated to be 0.20 h−1, which was superior to that of R. capsulatus BR (0.13 h−1), R. sphaeroides BL6 (0.17 h−1) or R. sphaeroides GB1 (0.18 h−1). These specific growth rates are fairly good in comparison with previously reported values: the specific growth rate of R. sphaeroides was 0.08 h−1 when cultivated in a medium containing succinate as a C source and (NH4)2SO4 as an N source (Jeon & Kim, 2010), and that of R. sphaeroides was 0.12 h−1 when cultivated in the modified Sistrom’s minimal medium containing trace elements and vitamins (Orsi et al., 2019). Thus, the basal medium provided test PSB with sufficient nutrition, and R. azotoformans EBN-7 was selected to be used for scale-up cultivation.
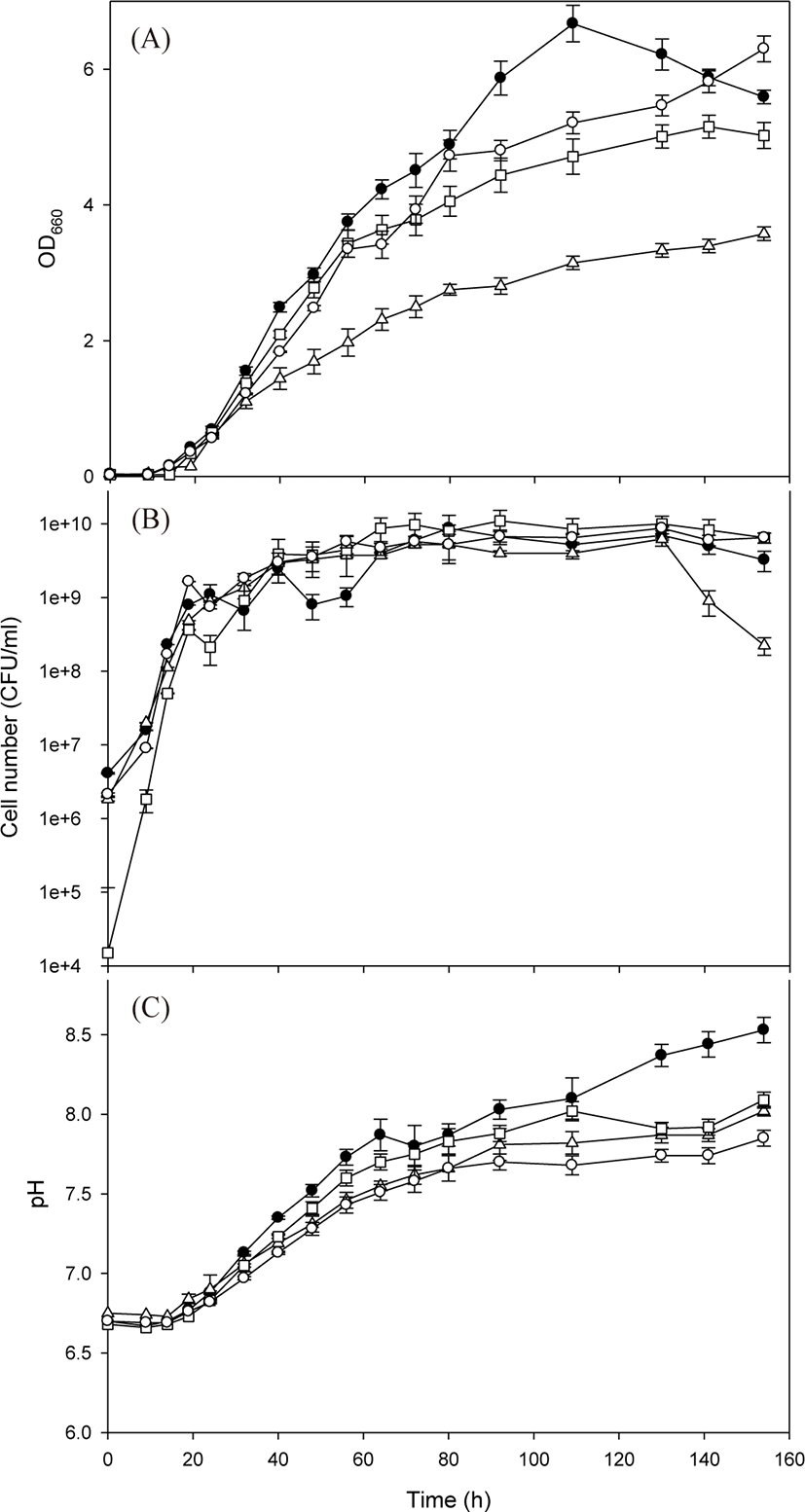
In the cultivation of all four PSB species, pH tended to increase gradually with cell growth (Fig. 3B). However, the tendency of increase in pH diminished after 80 h, except in R. azotoformans EBN-7. The time point at which the increase in pH diminished was almost consistent with that at which the increase in OD660 was reduced, particularly in the cultivation of R. capsulatus BR. After 154 h, the maximum pH values of 8.53, 8.09, 8.02 and 7.85 were recorded for R. azotoformans EBN-7, R. sphaeroides BL6, R. capsulatus BR, and R. sphaeroides GB1, respectively. The highest numbers of viable cells of four PSB species were 8.75 × 109 after 80 h for R. azotoformans EBN-7, 6.25 × 109 after 130 h for R. capsulatus BR, 1.10 × 1010 after 92 h for R. sphaeroides BL6, and 8.75 × 109 after 130 h for R. sphaeroides GB1, respectively (Fig. 3C). The cultivation time to reach the highest number of viable cells was lower than that required to reach the maximum OD660 owing to the difference in cell materials used to measure these parameters. In all species, the highest number of viable cells reached over 1 × 109 between 32 h and 40 h. In a parallel experiment, the maximum dry-cell weight of R. azotoformans EBN-7 was observed to be 3.56 g/L after 109 h, whereas those of R. sphaeroides BL6, R. capsulatus BR, and R. sphaeroides GB1 were 3.49, 2.47, and 4.88 g/L, respectively, after 154 h. This tendency of maximum dry-cell weight was similar to that of maximum OD660 value for each PSB species. Therefore, there was a time lag between the point at which dry-cell weight or OD660 reached the maximum value and the point at which the highest number of viable cells was observed.
The four PSB species started to reveal a red tone after 19 h of cultivation, reached its maximum (Indian red; #CD5C5C) between 32 and 40 h, and thereafter the change in the red tone was not significant. The cultivation time to reach the highest red tone was similar to that required to reach the maximum number of viable cells (over 1 × 109 CFU/mL). After 154 h of cultivation, R. capsulatus BR culture broth was tinged with dark red (dark red; #8B0000), whereas R. sphaeroides GB1 culture broth was tinged with light red or orange red (crimson; #DC143C). The species R. azotoformans EBN-7 and R. sphaeroides BL6 lay almost halfway between the two red tones.
The morphological characteristics of the four PSB species cultivated on the basal medium were observed under a microscope at 154 h of cultivation (Fig. 4). The species R. azotoformans EBN-7 and R. sphaeroides BL6 were short rods (1 µm wide and 1−2 µm long), whereas the other two species were relatively longer rods (1 µm wide and 3−4 µm long for R. capsulatus BR; and 1 µm wide and 1.5−2 µm long for R. sphaeroides GB1, respectively). The motility of the longest rod, R. capsulatus BR, was inferior to that of the other three species. In addition, R. capsulatus BR formed 1−2 chains. On the basis of these findings, the species R. azotoformans EBN-7 was found to be the most appropriate for scale-up cultivation, with the highest specific growth rate (0.20 h−1). Therefore, R. azotoformans EBN-7 was used for scale-up cultivation in the subsequent experiments.

Following the selection of an ideal PSB species and an optimal culture medium, scale-up cultivation was performed in a 500 L reactor for 96 h (Fig. 5). At approximately 15 h after inoculation, R. azotoformans EBN-7 cells grew exponentially. This growth tendency was consistent with that observed in laboratory-scale cultivation. The pH reached 8.14 after 16 h and thereafter was intentionally maintained at 7. This pH was decided on the basis of the pH (8.1) at the maximum OD660 in laboratory-scale cultivation to avoid the retardation in cellular growth when the optimum pH was not maintained. The DO level decreased rapidly from the beginning of cultivation, and increased to 70% saturation immediately after simultaneously increasing the air flow rate and agitation speed at 24 h. However, DO level again declined rapidly owing to active cell growth and did not recover after 48 h, indicating that cells consumed more oxygen than that supplied. Further increase in aeration rate and agitation speed had an adverse effect on biomass concentration owing to cell aggregation. At the end of cultivation at 96 h, the number of viable cells reached 4.50 × 1010 (Fig. 5). Therefore, the scale-up cultivation resulted in a slightly higher number of viable cells with a slightly lower specific growth rate (0.15 h−1) than those obtained during cultivation in a 1 L flask. This slightly reduced value in the specific growth rate during scale-up experiments has also been reported in previous studies. The specific growth rate was decreased from 0.21 h−1 to 0.18 h−1 when R. capsulatus cultivated in a 1 L flask was transferred to a 500 L bioreactor (Cho et al., 2006). In addition, the specific growth rate of Rhodopseudomonas palustris decreased from 0.12 h−1 (in 1 L culture) to 0.068 h−1 (in 5 L culture) in batch-type bioreactors (Kim & Lee, 2000). This denotes the difficulty in scale-up cultivation.
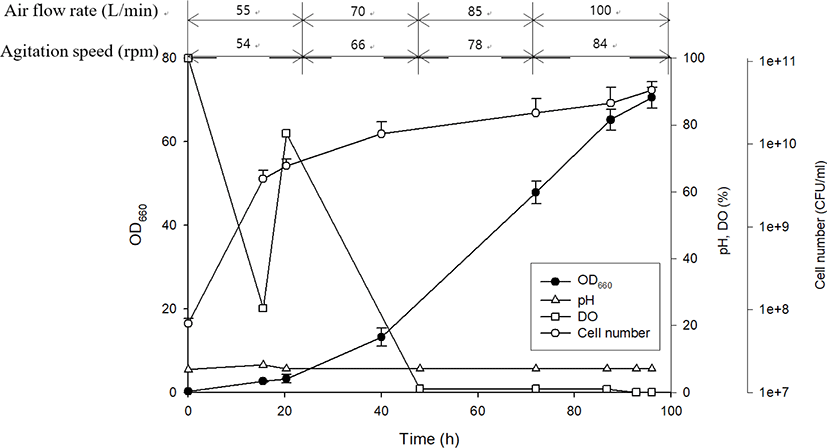
Prior to application to environmental fields, mass-cultivated PSB must be preserved. Moreover, it is cost effective if they can be preserved simply by storing at 4°C without reduction in cell activity. Therefore, R. azotoformans EBN-7 preserved at 4°C for different periods was used as seed culture for cultivation in a 250 L bioreactor to assess the extent of cell reactivation (Fig. 6). All cultivation systems using seed cultures preserved for different periods exhibited OD660 in the range of 1.02−1.56 and the number of viable cells in the range of 6.25 × 108−1.75 × 109. When used as seed culture, the cell reactivation ability of R. azotoformans EBN-7 decreased with increasing storage period, resulting in a decrease in both levels of cell growth and the number of viable cells in comparison with control PSB (not preserved). This phenomenon was severer when cells preserved for 4 months were seeded. The effect of preservation period on cultivation was also observed on color tone. The final culture broths exhibited a dark red (#8B0000) color after cells preserved for 1 to 3 months were seeded. However, the final culture broth exhibited the an Indian red (#CD5C5C) color after cells preserved for 4 months were seeded. Moreover, the cultivation system using seed culture preserved for 4 months exhibited two-phase separation (transparent in the upper phase and thick in the lower phase) and developed an odor. This likely occurred because of the prolonged maintenance under dark and anaerobic conditions. In conclusion, for use as seed culture, the maximum allowable period of preservation at 4°C for R. azotoformans EBN-7 was 3 months.
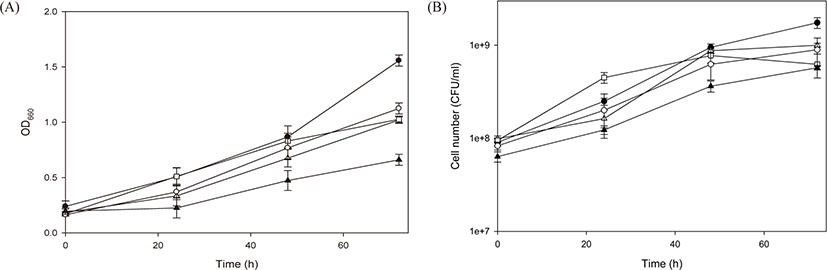
R. azotoformans EBN-7 was cultivated in a simple 15 L reactor to develop a simple culture technique for seed culture. R. azotoformans EBN-7 cultivated in a 500 L reactor was used as seed culture. As the seeded R. azotoformans EBN-7 grew, the color turned red (crimson; #DC143C) after 3 d (Fig. 7). This indicates that R. azotoformans EBN-7 easily proliferated in the reactor, which was confirmed by microscopic observation. After R. azotoformans EBN-7 was cultivated by the simple technique, it was applied to a shrimp aquaculture farm for the removal of NH4+-N from the water to test its potential. In the control group, the initial concentration of NH4+-N in the shrimp aquaculture water was 0.25 mg/L; the concentration of NH4+-N increased to 0.27 mg/L after 6 d and to 0.61 mg/L after 10 d (Fig. 8). In the treatment group, the cultivated R. azotoformans EBN-7 was applied at day 6 (0.27 mg/L of NH4+-N), and the NH4+-N concentration was reduced to 0.24 mg/L at day 10. Therefore, the treatment of shrimp aquaculture water with the cultivated R. azotoformans EBN-7 resulted in a 60.7% reduction in 4 d in comparison with the control. In aquaculture water treatment, ammonia-oxidizing archaea and anaerobic ammonia-oxidizing bacteria play a key role in the removal of nitrogen substances (Lu et al., 2021). Among those microorganisms, PSB were reported to reduce ammonia accumulation in an aquaculture pond. The NH4+-N content in the treatment group decreased by 29.81 % in comparison with control when R. palustris was applied to grass carp aquaculture water (Zhang et al., 2014). The potential ability of PSB in NH4+-N removal were also reported in other applications. Idi et al. (2015) demonstrated the ability of R. sphaeroides ADZ101 in the treatment of a synthetic wastewater containing NaNO3 as the sole nitrogen source. During the 7 d cultivation, the strain R. sphaeroides ADZ101 maximally removed 62% of NH4+-N from an initial concentration of 52 mg/L under anoxic growth conditions. In addition, Zhou et al. (2015a) reported that 83.2% NH4+-N was removed after 4 d when an ammonia-tolerant photosynthetic bacterium (ISASWR2014) was applied to chicken manure wastewater, which indicates a higher NH4+-N removal efficiency than that in our study. This difference may be caused by the difference in PSB species used for treatment. Moreover, the seed culture of PSB species used in this study was cultivated using a simple technique, and thus its quality may be somewhat lower than the PSB cultivated in a bioreactor under optimal conditions. Nevertheless, the cultivation of R. azotoformans EBN-7 using a simple culture technique exhibited a relatively good removal efficiency of NH4+-N. This enables seed culture prepared by a simple culture technique to be easily used for public.
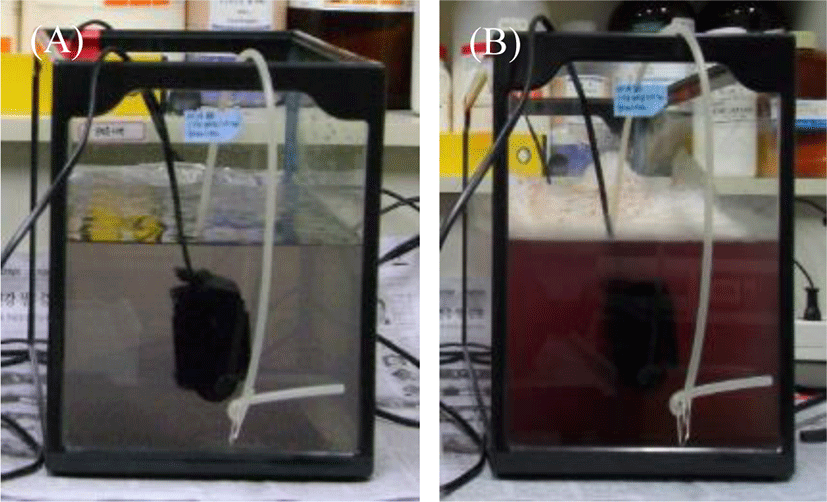
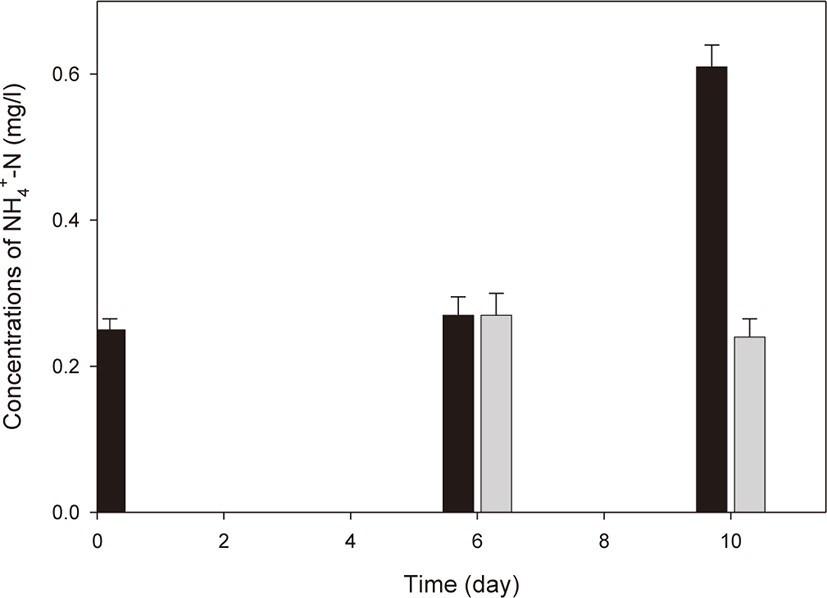
Conclusion
PSB are diversely utilized owing to their versatile metabolic abilities; nevertheless, their cultivation is difficult. Therefore, a generalized culture technique has not been previously reported. Considering the importance of cultivation, a simple culture technique suitable for public use was investigated. The strain Rhodobacter azotoformans EBN-7 was found to be the most appropriate for scale-up cultivation, with the highest specific growth rate (0.20 h−1) on basal medium, and it showed 4.50 × 1010 colony-forming units mL−1 (number of viable cells), dry cell weight of 26.8 g/L, and a specific growth rate of 0.15 h−1 in scale-up cultivation (500 L). As seed culture, this final culture broth was successfully cultivated in a 15 L simple reactor, with maintenance of cell activity evident. The maximum allowable preservation period of R. azotoformans EBN-7 at 4°C was 3 months for use as seed culture. In this study, cultivation of R. azotoformans EBN-7 in a simple reactor and preservation of R. azotoformans EBN-7 at 4°C for < 3 months exhibited its potential in the removal of NH4+-N by 60.7% (from 0.61 to 0.24 mg/L) in shrimp aquaculture water, allowing its use as seed culture without reduction in cell growth potential. Therefore, this simple technique showing a good removal efficiency of NH4+-N would considerably contribute to the dissemination of useful PSB for varied applications.